Abstract
The risk of iatrogenic damage is very high in surgical interventions in or around the brainstem. However, surgical techniques and intraoperative neuromonitoring (ION) have evolved sufficiently to increase the likelihood of successful functional outcomes in many patients. We present a critical review of the methodologies available for intraoperative monitoring and mapping of the brainstem. There are three main groups of techniques that can be used to assess the functional integrity of the brainstem: 1) mapping, which provides rapid anatomical identification of neural structures using electrical stimulation with a hand-held probe, 2) monitoring, which provides real-time information about the functional integrity of the nervous tissue, and 3) techniques involving the examination of brainstem reflexes in the operating room, which allows for the evaluation of the reflex responses that are known to be crucial for most brainstem functions. These include the blink reflex, which is already in use, and other brainstem reflexes that are being explored, such as the masseter H-reflex. This is still under development but is likely to have important functional consequences. Today an abundant armory of ION methods is available for the monitoring and mapping of the functional integrity of the brainstem during surgery. ION methods are essential in surgery either in or around the brainstem; they facilitate the removal of lesions and contribute to notable improvements in the functional outcomes of patients.
Surgery related to lesions in or around the brainstem represents one of the most challenging procedures in neurosurgery and requires a detailed understanding of the microsurgical and functional anatomy. In the past, due to the high risk of severe postoperative neurological deficits, this surgery was restricted to biopsy procedures. The development of intraoperative neurophysiology over the last two decades, together with the emergence of more refined imaging techniques, better surgical instruments, and more effective neuroanesthesia and postoperative intensive care, have increased the feasibility and safety of brainstem surgery.
Classical methods of neurophysiologic monitoring such as somatosensory-evoked potentials (SEPs) and brainstem auditory-evoked potentials (BAEPs) were extensively used in the past. However, these two methods are suitable for evaluating functioning in less than 20% of brainstem areas. This situation prompted the development of new methods for evaluating the functional integrity of the motor pathways passing through the brainstem based on recording the corticospinal motor evoked potentials (MEPs) in limb muscles1 and the corticobulbar MEPs (CoMEPs) in cranial-nerve-innervated muscles.234 However, these methods are not mapping techniques, and so they cannot help to locate specific areas of the brainstem. Therefore, a methodology for mapping—the neurophysiologic identification of anatomical structures—is still required.
Compression-related displacement of classical anatomical landmarks such as the facial colliculus or the striae medullaris is a common difficulty for neurosurgeons. Even when the anatomy is not significantly distorted, the identification of areas overlapping the lower cranial nerve motor nuclei (CMN) can represent a major challenge. Mapping techniques can be used to help with the intraoperative identification of key structures such as the CMN of cranial nerves III, IV, V, VII, IX, X, and XII on the floor of the fourth ventricle.56 Results from direct electrical stimulation of the brainstem may be invaluable for choosing the safest approach for intrinsic brainstem tumors. It should be stressed that mapping techniques should never replace monitoring techniques, since only the latter allows the real-time assessment of the functional integrity of neuronal pathways. The optimal choice of the methodology to be used depends on the stage of surgery.
In this review we consider three main groups of neurophysiologic techniques that may help monitoring the brainstem functional integrity: 1) mapping, which provides rapid anatomical identification of neural structures by electrical stimulation with a hand-held probe, 2) monitoring, which provides real-time information about the functional integrity of the nervous tissue,7 and 3) intraoperative testing of the brainstem reflexes. Mapping and monitoring techniques complement each other and should be used together during challenging surgical procedures such as tumor resection in or around the brainstem. We summarize the techniques already widely used for the intraoperative assessment of brainstem functional integrity and provide in-depth descriptions of those that are less well known, such as assessment of the blink reflex (BR), and those that are still under development, such as assessment of the masseter H-reflex.
Brainstem mapping is a neurophysiologic method for locating the CMN. These nuclei are located in the vicinity of specific anatomical landmarks on the floor of the fourth ventricle, where their presumed location can be identified with the aid of anatomical landmarks such as the striae medullaris or facial colliculi.8 However, this is difficult when the anatomy is compromised, and becomes even more difficult in the presence of a tumor that might have displaced the landmarks. Strauss et al.5 used electrical stimulation to identify the CMN, and their technique was subsequently improved by Morota and Deletis (Fig. 1).6 The use of CMN mapping helps the surgeon to understand the functional anatomy when the floor of the fourth ventricle is distorted, and is therefore an indispensable tool when performing challenging brainstem surgery.
Intraoperative neurophysiologic localization of the CMN reveals specific patterns of displacement by brainstem tumors. Pontine tumors displace the CMN of cranial nerve VII around the edge of the tumor; medullary tumors displace the low CMN ventrally, while cervicomedullary spinal cord tumors displace the low CMN cranially and laterally. Understanding the patterns of CMN displacement can help to establish a surgical plan that minimizes the risk of damaging the CMN, determines a safe location for the surgical incision into the brainstem, and improves the safety of surgery for brainstem tumors (Fig. 2).
Liscić et al.9 reported on how brainstem tumors affect the location of CMN. Those authors mapped the lower CMN of 26 hypoglossal nerves and compared patients who underwent surgery for either medullary or pontine tumors. Their data showed that the latency of compound muscle action potentials of the genioglossus muscles was significantly longer in patients with medullary tumors than in those with upper brainstem tumors (5.3±0.3 ms vs. 4.1±0.6 ms, mean±SD). Those patients did not exhibit clinical neurological deficits of the lower cranial nerves. The data indicated an unrecognized compressive neuropathy in this group of patients, but did not show any predictive value regarding the neurological outcome after surgery.
The first goal of cranial nerve mapping is to find regions of the tumor that do not affect the nervous tissue, which the surgeon can therefore remove without the risk of permanent damage to the nervous tissue. This can be achieved most easily using a monopolar or bipolar hand-held stimulating electrode. The second goal of cranial nerve mapping is to identify nerve trajectories, and the third is to detect the exact time when injuries to the cranial nerve occur.
Continuous EMG monitoring of the cranial nerves supplying muscles in the absence of electrical stimulation may be useful for the third of these goals. Although a wide variety of EMG patterns has been described, the descriptions have been based on acoustic EMG characteristics. Romstöck et al.11 developed a classification system for EMG activity that was based on visual offline analysis of the waveform characteristics, frequencies, and amplitudes of a multichannel, free-running EMG tracing. They divided the train activity into three groups. One of these particular patterns, the "A" train, which was described as a sinusoidal shape with a high frequency and homogeneous appearance, was demonstrated to be the only pattern that clearly indicated postoperative paresis with high sensitivity and specificity.12 However, large numbers of false-positive and false-negative results were obtained. Fals-enegative results might be due to not all muscles innervated by the studied nerve being monitored, resulting in some ininformation being missed, while Prell et al.13 attributed false-positive results to manipulation of the intermediate nerve leading to A-train activity without any clinical correlation. For these reasons, free-running EMG is not sufficiently accurate for monitoring the functional integrity of the cranial nerves. Alternative techniques for continuous monitoring of the cranial nerves are described in detail in the Monitoring Techniques section.
Damage to the corticospinal tract (CST) is a major concern when performing surgery close to the cerebral peduncle or the ventral part of the medulla. Like other areas of neurosurgery, brainstem surgery has benefited significantly from the introduction of techniques for the intraoperative recording of MEPs.56 Besides continuous recording of muscle or epidural MEPs, mapping of the CST at the level of the cerebral peduncle has also become both feasible and reliable (Fig. 3).14 Monitoring of the CST functional integrity is described later in the text.
Only two methods were available for monitoring the functional integrity of the brainstem in the early 1990s: BAEPs and SEPs. According to Fahlbusch and Strauss,15 these two methods could evaluate only 20% of the brainstem area, assessing only two pathways within the brainstem that were not necessarily particularly relevant to the postoperative functionality of the patients. Those two methods were useful for documenting but not for preventing surgically induced neurological injury to the somatosensory and auditory pathways. As an example, Fig. 4 shows data from a patient who experienced postoperative hemihypoesthesia caused by an incision to the lower brainstem and damage to one side of the dorsal column nuclei. Monitoring of SEPs documented but did not prevent this neurological deficit during the initial incision in the brainstem.
Polo et al.16 established new criteria for BAEP changes during brainstem surgery in order to prevent hearing loss. They identified the following warning signals based on the latency delay of peak V compared to baseline: a delay of 0.4 ms could be considered within the limits of safety, a delay of 0.6 ms is a warning for possible hearing impairment, and a delay of 1 ms may indicate irreversible hearing loss.
The CST is anatomically compressed within a small portion of the brainstem surface, and is therefore at risk during many brainstem-related procedures. Recording MEPs from limb muscles elicited by transcranial electrical stimulation (TES) using a short train of stimuli provides real-time information on the functional integrity of the CST. Quiñones-Hinojosa et al.17 demonstrated that MEP changes were more robust and occurred earlier than SEP changes in the presence of a basilar artery aneurysm, indicating that MEPs may be more sensitive than SEPs to brainstem ischemia caused by insufficient blood flow in the basilar artery and its perforating arteries. The predictive value of MEPs with regard to the patient motor outcome was stressed by Neuloh et al.,1 who concluded that MEP monitoring—but not SEP monitoring—is a valid indicator of corticospinal functional integrity during brainstem-related surgery, regardless of the type of lesion being operated on.
When feasible, it is worth simultaneously recording responses to TES using epidural electrodes (which measures the D-wave), although attention should be paid to potential confounding technical factors. The criteria for assessing lesions in the CST and related confounding factors have been reported by Deletis et al.14 and Macdonald et al.18
CoMEP monitoring was developed for continuous monitoring of the functional integrity of the corticobulbar pathways and cranial motor nerves together with their nuclei. Since the first description of recording the MEPs in facial muscles by Dong et al.,2 techniques for the assessment of corticobulbar pathways to other cranial nerves have also been reported.34 The method consists of TES applied to the motor strip (M1) for the cranial motor nerves and recordings of CoMEPs over appropriate muscles innervated by cranial motor nerves (Fig. 5).
In order to activate the corticobulbar tract (CBT) transcranially by TES, we used montage C3(+) vs. Cz(-) for left hemispheric stimulation and C4(+) vs. Cz(-) for right hemispheric stimulation (Fig. 5). Verst et al.19 advocated montage C5(+) vs. Cz(-) for left hemispheric stimulation and C6(+) vs. Cz (-) for right hemispheric stimulation because this corresponds better to the anatomy of M1 for the corticobulbar pathways. The following stimulation parameters were used in the present study: a short train consisting of three to five stimuli each with duration of 0.5 ms, separated by an interstimulus interval of 2 ms, with a train repetition rate of 2 Hz, and an intensity typically ranging from 50 to 150 mA, seldom up to 200 mA. We apply a single stimulus to the same electrodes at 90 ms after delivering a short train of stimuli (Fig. 6). The rationale for this kind of stimulation is that only a short train of stimuli can elicit "central" responses (activation of the CBT for the cranial nerves) generated by the motor cortex or the subcortical part of the CBT in most patients under general anesthesia. If a single stimulus elicits a response, this should be considered a "peripheral" response due to the spreading of current distally and activation of the cranial nerve directly rather than through the CBT. This allows for continuous feedback20 and represents an improvement on the original methodology described by Dong et al.2 Our previous study we showed that single facial nerve stimuli generated responses only when hyperexcitability was present in the facial nerve and facial nuclei.21 The intensity of the TES train was adjusted to obtain the maximum amplitude of CoMEPs while TES using identical intensity applying a single stimulus did not activate the peripheral part of the facial nerve. We chose a 90-ms interval between the first train and the beginning of the next single stimulus in order to prevent stimulation of the facial nerve when it became hyperexcitable after the first train of stimuli ("subthreshold hyperexcitability"22).
CoMEP monitoring is interrupted if the surgeon wants to identify any portion of the cranial nerve using local electrical stimulation (mapping) with a hand-held probe.
The CoMEPs are monitored from different muscles of the face and neck. The methodology for selective recordings from target muscles was described by Deletis et al.34 A pair of "hook-wire" electrodes is used for these recordings, each consisting of Teflon-coated wire 76 µm in diameter passing through 27-gauge needles (hook-wire electrode, specially modified; Viasys Healthcare, Madison, WI, USA). The tip 2 mm of the Teflon insulation is stripped off the recording wires, and the tips are curved to form a hook to anchor them after the needle is withdrawn from the facial muscles. The impedance of the electrodes is less than 20 kOhm. After inserting each wire electrode into the muscle, the needle is withdrawn and the wires are braided to reduce mains-power interference and then covered with tape to reduce any stimulus artifacts to the skin and to protect the patient from accidental injury. These recently designed hook-wire electrodes allow convenient the withdrawal of the needle after inserting the wire into the muscle. The recording electrodes are placed in different muscles after patient intubation is completed.
During brainstem surgery, CoMEPs are elicited by TES, which is not a sufficiently selective technique for the stimulus to target the CBT. Therefore, in order to ensure that the recorded CoMEPs correspond to the cranial nerve under investigation, the recording must be selective; otherwise far-field activity from other cranially innervated muscles—activated by other corticobulbar pathways—can contaminate the CoMEP recordings from the muscle intended to record from, and give false-negative results.23 In order to prevent this, hook-wire electrodes with a diameter of 76 µm with small recording surfaces are recommended rather than a standard EEG needle. Their small surfaces selectively record activity from the target muscles, thus avoiding far-field recordings.
The monitoring of trigeminal SEPs (T-SEPs) has been an established technique in neurological diagnoses for decades. Several studies have been performed in awake neurological patients, with different techniques used to stimulate the branches of the trigeminal nerve. Measurements of T-SEPs in awake patients have been widely reported to be very sensitive to noise, which is frequently explained as a stimulus artifact or artifacts from contraction of the oral facial muscles. However, a reliable methodology for eliciting T-SEPs under general anesthesia was described by Malcharek et al.24 They showed that it is possible to obtain reproducible and stable recordings of T-SEPs after stimulation of the second and third branches of the trigeminal nerve. This methodology might yield important information on the functional integrity of sensory fibers for the trigeminal nerve during brainstem surgery, although more studies are needed in order to determine the correlation between T-SEP changes and clinical outcomes of the trigeminal nerve in this type of surgery.
Surgery of the brainstem may result in postoperative neurological deficits, including transient difficulties in coughing, swallowing, or other brainstem complex reflexes, in spite of preserved neurophysiologic parameters. Therefore, a method able to monitor some of these reflexes could be very useful. An obvious problem to achieving this is that most brainstem reflexes disappear during anesthesia, making it difficult or even impossible to elicit them. One solution to this problem is to use a short train of high-frequency stimuli, which is able to partly overcome the depressive effects of anesthetics. A methodology for eliciting the BR has been developed using this approach, as are methods for making intraoperative recordings of the masseter H-reflex.
Deletis et al.25 described a method for eliciting the R1 component of the BR in patients under general anesthesia. They applied one to seven rectangular constant-current stimuli with an interstimulus interval of 2 ms, an intensity of 20–40 mA, and a train repetition rate of 0.4 Hz over the supraorbital nerve (Fig. 7). The R1 corresponds to an oligosynaptic reflex arc and includes trigeminal afferents, brainstem connections between the trigeminal and facial nuclei, and the facial nerve. Therefore, this technique might be considered a new tool for intraoperative monitoring involving structures in the reflex arc. The second author recently found that there was a strong correlation between BR and facial and/or trigeminal CoMEPs during skull-base surgery in 21 patients undergoing posterior fossa surgeries, with this being a predictor of clinical outcome.
The masseter nerve can be stimulated by introducing percutaneously either a pair of hook-wire electrodes or a pair of monopolar EMG needle electrodes (902-DMG37, TECA, Pleasantville, NY, USA) just under the zygomatic arch, 0.5 cm lateral to the temporomandibular joint (Fig. 8). The insertion depth of the stimulating needle electrode is established when the stimulating current elicits a stimulus-related response in the masseter and temporalis muscles. Single stimuli with a duration of 0.2–0.5 ms and a steady increase in intensity are applied. The repetition rate is 0.7–1 Hz with a 20-ms epoch and bandpass digital filter from 1 to 2,133 Hz.
H-reflexes and M-responses can be recorded by inserting disposable twisted-pair subdermal needle electrodes (Viasys Healthcare) into the ipsilateral masseter and temporalis muscles.
The development of methods for intraoperatively identifying (mapping) and continuously testing the functional integrity of the nervous structure (monitoring) within the brainstem has become a "conditio sine qua non" during brainstem surgery. This is true for the mapping and monitoring of the CST as well as the CMN.
Intraoperative methods for monitoring the more complex functions of the brainstem based on reflexes must be developed in order to prevent damage to its very complicated functions. The development of a method to monitor complex and polysynaptic brainstem reflexes will obviously be a very challenging task, given that they disappear during general anesthesia. So far it has been possible to monitor the BR, while a method for monitoring the H-reflex in the masseter muscle is under development.
Figures and Tables
Fig. 1
Mapping of the brainstem cranial nerve motor nuclei (CMN). Upper left: drawing of the exposed floor of the fourth ventricle with the surgeon's hand-held stimulating probe in view. Upper middle: sites where hook-wire electrodes were inserted for recording muscle responses. Far upper right: compound muscle action potentials recorded from the orbicularis oculi and oris muscles after stimulation of the upper and lower facial nuclei (upper two traces), and from the pharyngeal wall and tongue muscles after stimulation of the CMN of cranial nerves IX, X, and XII (lower two traces). Lower left: photograph obtained with the aid of the operating microscope showing the hand-held stimulating probe placed on the floor of the fourth ventricle (F). A: aqueduct. Adapted from Morota et al., Neurosurgery 1995;37:922-929; discussion 929-930, with permission from Wolters Kluwer Health, Inc.10
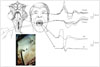
Fig. 2
Typical patterns of CMN displacement by brainstem tumors in different locations. Upper and lower pontine tumors typically push the facial nuclei around the edge of the tumor, suggesting that it is necessary to precisely locate the facial nuclei before tumor resection in order to avoid them being damaged during surgery. Medullary tumors typically grow more exophytically and compress the lower CMN ventrally; these nuclei may be located on the ventral edge of the tumor cavity. Because of the interposed tumor, in these cases mapping before tumor resection usually does not allow identification of the CMN of cranial nerves IX, X, and XII. However, responses may be obtained close to the end of the tumor resection after most of the tumor tissue between the stimulating probe and the motor nuclei has been removed. Repeated mapping is recommended at this point since the risk of damaging motor nuclei is significantly higher than at the beginning of tumor debulking. Cervicomedullary junction spinal cord tumors simply push the lower CMN rostrally when they extend into the fourth ventricle. Adapted from Morota N, et al., Neurosurgery 1996;39:787-793; discussion 793-794, with permission from Wolters Kluwer Health, Inc.8 CMN: cranial nerve motor nuclei.
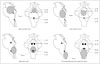
Fig. 3
Mapping and monitoring of the corticospinal tract (CST) during surgery for removing a left cerebral peduncle tumor in a 27-year-old woman. Bottom left: preoperative axial T1-weighted MR image with gadolinium enhancement revealed a high-intensity lesion in the left midbrain, dorsal to the cerebral peduncle. Bottom right: postoperative axial T1-weighted MR image with gadolinium enhancement showing that the tumor has been completely removed. The incision was performed in the area where no response to the stimulation of the cerebral peduncles was recorded. Top right: mapping of the CST on the cerebral peduncle is shown schematically. The cerebral peduncle is being mapped by a hand-held monopolar probe. As the probe neared the CST, responses were recorded from an epidural catheter (middle right). The responses were consistently reproducible. The stimulation intensity was 2 mA, the stimulation rate was 4 Hz, and four responses were averaged. Middle left: monitoring of the CST during tumor resection. After CST mapping, motor evoked potentials were continuously monitored by recording D-waves epidurally after transcranial electrical stimulation. The D-waves remained stable throughout the procedure and, the patient's preoperative motor function reappeared postoperatively. Adapted from Deletis V, et al., Operative Tech Neurosurg 2000;2:109-113, with permission from Elsevier.14
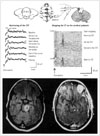
Fig. 4
Intraoperative microscope photograph (A) illustrating the location of the cavernoma in the left hemimedulla. Preoperative axial (B) and sagittal (C) T1-weighted MR images showed a hyperintense lesion in the left dorsal medulla in a 26-year-old patient. Monitoring (D) of the somatosensory-evoked potentials (SEPs) during surgical removal of the cavernoma disclosed a selective lesion in the left dorsal column nuclei. During the initial incision in the region of the dorsal column nuclei, SEPs after the left median nerve stimulation diminished (*) and subsequently disappeared (not shown), whereas SEPs from the right median nerve remained intact. The patient woke up after surgery with significant sensory loss in the left arm. In this patient we could only document the lesion, being unable to remove it. Adapted from Deletis V, et al., Operative Tech Neurosurg 2000;2:109-113, with permission from Elsevier.14
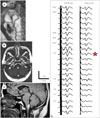
Fig. 5
Intraoperative schematic of eliciting and recording of corticobulbar motor-evoked potentials (CoMEPs). Lower left: schematic of positioning the stimulating electrode over the scalp. Upper left: schematic of the corticobulbar pathways innervating CMN (cranial nerves VII, IX, X, and XII). Middle: positioning the recording electrodes inserted in the orbicularis oris, pharyngeal, tongue, and vocal muscles (cranial nerves VII, IX, XII, and X, respectively) to monitor CoMEPs. To the right: typical examples of CoMEPs recorded from muscles innervated by cranial motor nerves.
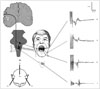
Fig. 6
CoMEPs recorded from the mentalis muscle after TES of the motor cortex in a patient with a vestibular schwannoma. A: Axial MR image showing a right vestibular schwannoma. B: Facial CoMEPs recorded with hook-wire electrodes (C) from the mentalis muscle. The facial nerve was accidentally damaged during surgery, after which the facial CoMEPs disappeared (closing). D: Facial CoMEPs recorded with an EEG needle (E) from the mentalis muscle. After damaging the facial nerve there was still a recordable response (red circle), which was considered to be a far-field response of surrounding muscles such as those of the tongue (closing). Adapted from Fernandez-Conejero I, et al., J Neurosurg 2014;120:291-292, with permission from American Association of Neurological Surgeons.21 CoMEPs: corticobulbar motor-evoked potentials.
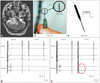
Fig. 7
Recording the R1 component of the blink reflex (BR). To the left: recordings of the R1 component of the BR from one patient. In this patient a train of four stimuli elicited the responses, whereas trains consisting of one, two, or three stimuli did not. Each trace represents the average of two responses. To the right: recordings of the R1 component of the BR from another patient showing the high reproducibility of the responses. Each trace represents the average of two responses. The last trace represents the superposition of the first six. Adapted from Deletis V, et al., Muscle Nerve 2009;39:642-646, with permission from Wiley Periodicals, Inc.25
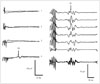
Fig. 8
Recording M-responses and H-reflexes from the masseter muscle. To the left: schematic of the anatomy of the masseter nerve innervating the masseter and temporalis muscles. To the right: after stimulation, masseter nerve recordings of the H-reflexes and M-responses of the masseter muscle were found to be correlated with the stimulus intensities. Adapted from Godaux E, et al., Brain Res 1975;85:447-458, with with permission from Elsevier26 (to the left) and Téllez et al. 2015 (personal communications) (to the right).
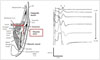
Table 1
Neurophysiological parameters for mapping cranial motor nerves nuclei
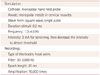
References
1. Neuloh G, Bogucki J, Schramm J. Intraoperative preservation of corticospinal function in the brainstem. J Neurol Neurosurg Psychiatry. 2009; 80:417–422.


2. Dong CC, Macdonald DB, Akagami R, Westerberg B, Alkhani A, Kanaan I, et al. Intraoperative facial motor evoked potential monitoring with transcranial electrical stimulation during skull base surgery. Clin Neurophysiol. 2005; 116:588–596.


3. Deletis V, Fernandez-Conejero I, Ulkatan S, Costantino P. Methodology for intraoperatively eliciting motor evoked potentials in the vocal muscles by electrical stimulation of the corticobulbar tract. Clin Neurophysiol. 2009; 120:336–341.


4. Deletis V, Fernández-Conejero I, Ulkatan S, Rogić M, Carbó EL, Hiltzik D. Methodology for intra-operative recording of the corticobulbar motor evoked potentials from cricothyroid muscles. Clin Neurophysiol. 2011; 122:1883–1889.


5. Strauss C, Romstöck J, Nimsky C, Fahlbusch R. Intraoperative identification of motor areas of the rhomboid fossa using direct stimulation. J Neurosurg. 1993; 79:393–399.


6. Morota N, Deletis V. The importance of brainstem mapping in brainstem surgical anatomy before the fourth ventricle and implication for intraoperative neurophysiological mapping. Acta Neurochir (Wien). 2006; 148:499–509. discussion 509.


7. Deletis V. Evoked Potentials. In : Lake CL, editor. Clinical Monitoring for Anesthesia and Critical Care. 2nd ed. Lake Carol: W.B. Saunders;1994. p. 282–314.
8. Morota N, Deletis V, Lee M, Epstein FJ. Functional anatomic relationship between brain-stem tumors and cranial motor nuclei. Neurosurgery. 1996; 39:787–793. discussion 793-794.


9. Liscić RM, Morota N, Deletis V. Intramedullar stimulation of the facial and hypoglossal nerves: estimation of the stimulated site. Croat Med J. 2000; 41:384–388.
10. Morota N, Deletis V, Epstein FJ, Kofler M, Abbott R, Lee M, et al. Brain stem mapping: neurophysiological localization of motor nuclei on the floor of the fourth ventricle. Neurosurgery. 1995; 37:922–929. discussion 929-930.
11. Romstöck J, Strauss C, Fahlbusch R. Identification of cranial nerve nuclei. Muscle Nerve. 2000; 23:1445–1446.


12. Romstöck J, Strauss C, Fahlbusch R. Continuous electromyography monitoring of motor cranial nerves during cerebellopontine angle surgery. J Neurosurg. 2000; 93:586–593.


13. Prell J, Strauss C, Rachinger J, Scheller C, Alfieri A, Herfurth K, et al. The intermedius nerve as a confounding variable for monitoring of the free-running electromyogram. Clin Neurophysiol. 2015; 126:1833–1839.


14. Deletis V, Sala F, Morota N. Intraoperative neurophysiological monitoring and mapping during brain stem surgery: a modern approach. Oper Tech Neurosurg. 2000; 2:109–113.


15. Fahlbusch R, Strauss C. [Surgical significance of cavernous hemangioma of the brain stem]. Zentralbl Neurochir. 1991; 52:25–32.
16. Polo G, Fischer C, Sindou MP, Marneffe V. Brainstem auditory evoked potential monitoring during microvascular decompression for hemifacial spasm: intraoperative brainstem auditory evoked potential changes and warning values to prevent hearing loss--prospective study in a consecutive series of 84 patients. Neurosurgery. 2004; 54:97–104. discussion 104-106.


17. Quiñones-Hinojosa A, Alam M, Lyon R, Yingling CD, Lawton MT. Transcranial motor evoked potentials during basilar artery aneurysm surgery: technique application for 30 consecutive patients. Neurosurgery. 2004; 54:916–924. discussion 924.


18. Macdonald DB, Skinner S, Shils J, Yingling C. American Society of Neurophysiological Monitoring. Intraoperative motor evoked potential monitoring - a position statement by the American Society of Neurophysiological Monitoring. Clin Neurophysiol. 2013; 124:2291–2316.


19. Verst SM, Sucena AC, Maldaun MV, Aguiar PH. Effectiveness of C5 or C6-Cz assembly in predicting immediate post operative facial nerve deficit. Acta Neurochir (Wien). 2013; 155:1863–1869.


20. Ulkatan S, Deletis V, Fernandez-Conejero I. Central or peripheral activations of the facial nerve? J Neurosurg. 2007; 106:519–520. author reply 520.


21. Fernández-Conejero I, Deletis V. Transcranial electrical stimulation and monitoring. J Neurosurg. 2014; 120:291–292.
22. Bostock H, Lin CS, Howells J, Trevillion L, Jankelowitz S, Burke D. After-effects of near-threshold stimulation in single human motor axons. J Physiol. 2005; 564(Pt 3):931–940.


23. Fernández-Conejero I, Ulkatan S, Sen C, Deletis V. Intra-operative neurophysiology during microvascular decompression for hemifacial spasm. Clin Neurophysiol. 2012; 123:78–83.


24. Malcharek MJ, Landgraf J, Hennig G, Sorge O, Aschermann J, Sablotzki A. Recordings of long-latency trigeminal somatosensoryevoked potentials in patients under general anaesthesia. Clin Neurophysiol. 2011; 122:1048–1054.

