Abstract
Background and Purpose
The receptor for advanced glycation end products (RAGE) may contribute to the development of diabetic neuropathy. To assess its relevance in humans, this study examined the expression of RAGE in the skin biopsy samples of patients with diabetes mellitus, and investigated its correlation with intraepidermal nerve-fiber density (IENFD) and clinical measures of neuropathy severity.
Methods
Forty-four patients who either had type 2 diabetes or were prediabetes underwent clinical evaluation and a 3-mm skin punch biopsy. The clinical severity of their neuropathy was assessed using the Michigan Diabetic Neuropathy Score. IENFD was measured along with immunohistochemical staining for RAGE in 29 skin biopsy samples. The expression of RAGE was also quantified by real-time reverse-transcription PCR in the remaining 15 patients.
Results
RAGE was localized mostly in the dermal and subcutaneous vascular endothelia. The staining was more intense in patients with a lower IENFD (p=0.004). The quantity of RAGE mRNA was significantly higher in patients with severe neuropathy than in those with no or mild neuropathy (p=0.003). The up-regulation of RAGE was related to dyslipidemia and diabetic nephropathy. There was a trend toward decreased sural nerve action-potential amplitude and slowed peroneal motor-nerve conduction with increasing RAGE expression.
Diabetic neuropathy is a common complication of diabetes mellitus, typically manifesting as chronic symmetric length-dependent sensorimotor axonal polyneuropathy.1 It can cause significant functional impairment and disability in diabetic patients, leading to a reduced quality of life as well as considerable costs for health care.2 There is no specific disease-modifying therapy, and the exact pathogenesis and molecular mechanisms remain elusive.3,4,5 Skin punch biopsy and quantification of intraepidermal nerve-fiber density (IENFD) have recently emerged as an early surrogate marker of both diabetic neuropathy and small-fiber neuropathy.6,7,8,9 IENFD is negatively associated with the duration of diabetes and with neuropathic deficits,10,11 and is significantly reduced in patients even at the early stage of diabetic neuropathy when nerve conduction is normal.9,12
Advanced glycation end products (AGE) and their receptors (RAGE) are of particular interest with respect to their potential contribution to development of microvascular complications of diabetes.13,14 AGEs result from the nonenzymatic glycation of proteins, lipids, and nucleotides, and chronic hyperglycemia in diabetes enhances their formation and accumulation causing possibly detrimental structural and functional modifications of macromolecules.15 AGEs also trigger intracellular signaling pathways by binding to one of their cell-surface receptors, RAGE, leading to the activation of nuclear factor kappa B (NF-κB) and nicotinamide adenine dinucleotide phosphate (NADPH) oxidases, which ultimately increases the levels of oxidative stress and inflammation.16,17,18,19
Recent studies have implicated the activation of RAGE as a possible mechanism of diabetic neuropathy.17,18,19,20 The expression of RAGE was found to increase in the peripheral nervous system of experimental animal models of diabetic neuropathy.13,17,20 Deletion of RAGE was found to protect mice from diabetic neuropathy, while its overexpression promoted diabetic neuropathy.13,17,19,20 In addition, loss of pain perception and the structural changes of neuropathy were largely prevented in RAGE-knockout mice.17,20 However, the relevance of RAGE expression, particularly in terms of small-fiber loss, has not been investigated in human diabetic neuropathy. The aim of this study was to determine the association between RAGE expression in the skin and IENFD by examining the expression of RAGE using immunohistochemistry and real-time reverse-transcription (RT) PCR (RT-PCR). We also analyzed the correlations between RAGE expression and the overall clinical severity of diabetic neuropathy, large-fiber pathology measured by nerve conduction studies, metabolic status, and markers of the microvascular complications of diabetes.
Forty-four patients who either had type 2 diabetes or were prediabetes (impaired fasting glucose or impaired glucose tolerance) as diagnosed according to the American Diabetes Association Clinical Practice Recommendations were included in this study, and all were recruited at the Seoul Metropolitan Government Boramae Medical Center between June 2010 and March 2011.21 The exclusion criteria included a history of inherited neuropathy, toxin exposure, any medical conditions including thyroid disease, nutritional deficiency, renal disease, and patients on medications with the potential for causing peripheral neuropathy such as chemotherapy drugs or tuberculosis treatment. All patients underwent a clinical evaluation that comprising a detailed neurological examination and laboratory tests that included fasting blood glucose, oral glucose tolerance test, hemoglobin A1c (HbA1c), lipids, thyroid function test, and vitamin B12. The degree of albuminuria was assessed, and diabetic retinopathy was graded into the following five categories: none, mild, moderate, severe nonproliferative retinopathy, and proliferative diabetic retinopathy.22 Written informed consent to participate was obtained from all patients, and the study was approved by the Institutional Review Board of Seoul Metropolitan Government Boramae Medical Center. The clinical severity of diabetic neuropathy was evaluated using the Michigan Diabetic Neuropathy Score, and the patients were categorized into four groups (none, mild, moderate, and severe neuropathy) based on the neurological examination and electrophysiological test results.23 The typical chronic, symmetric, length-dependent sensorimotor axonal polyneuropathy was the only variety of diabetic neuropathy in our study. Patients with diabetic neuropathy of focal/multifocal varieties or with demyelinating features were excluded, as were those with a generalized, but atypical polyneuropathy in terms of mode of onset and course.
Intraepidermal nerve-fiber density was measured, and the expression of RAGE was assessed by immunohistochemical staining of the skin biopsy samples from 29 patients. A skin sample was obtained by a 3-mm punch biopsy from the distal leg (10 cm above the lateral malleolus) from each of the selected patients after applying lidocaine for topical anesthesia.18 The biopsied skin was immediately fixed with 2% paraformaldehyde-lysine-periodate for 12-24 hours, and cryoprotected at 4℃. Half of the skin samples were sectioned at a thickness of 50 µm using a freezing sliding microtome, and the other half were reserved for immunohistochemical staining of RAGE. The 50-µm-thick skin sections were stained with polyclonal rabbit antihuman protein gene product 9.5 (PGP9.5) antibody (diluted 1:1,200; Chemicon, Billerica, MA, USA). The PGP9.5-immunoreactive intraepidermal nerve fibers were counted under a light microscope at a magnification of ×400; only those fibers crossing the dermal-epidermal junction were counted. The mean value of IENFD was derived from at least three sections and expressed as the fiber count per unit length.
The reserved skin specimens were thawed, washed three times with phosphate buffered saline, fixed in 10% neutral formalin, and then embedded in paraffin. Formalin-fixed paraffin-embedded skin samples were then sectioned and subjected to immunohistochemical staining for RAGE. The tissues were stained with monoclonal mouse antihuman RAGE antibody (diluted 1:200; Santa-Cruz Biotechnology, Santa-Cruz, CA, USA), and the staining intensity was graded from I to IV as follows: grade I, absence of staining; grade II, faint staining (only visible under high power); grade III, moderate staining (easily visible under low power); and grade IV, intense staining. The examiner (Y. Kim) was blinded to the identity of the specimens.
Total RNA was extracted, isolated, and purified from a frozen sample of each 3-mm skin punch biopsy specimen from 15 patients using the homogenizer extraction technique with a commercially available kit (RNeasy Mini Kit, Qiagen, Valencia, CA, USA). The RNA quantity and quality were assessed by UV spectroscopy (NanoDrop ND-1000, NanoDrop Technologies Inc., Wilmington, DE, USA), which assessed the ratio of the absorbances at 260 nm and 280 nm.24 The integrity of the total RNA was quantified by electrophoresis on a denaturing agarose gel with ethidium bromide.25 Reverse transcription using dissolved RNA in diethylpyrocarbonate-treated water was performed using a GeneAmp EZ rTth RNA PCR kit (Perkin Elmer) at 60℃ for 30 min.26 The real-time RT-PCR was carried using the LightCycler system (Roche Diagnostics, Basel, Switzerland) with SYBR Green as the fluorescent dye.27 The complementary DNA samples were amplified with specific primers. The RAGE primer sequences were as follows: forward, 5'-GGAAAGGAGACCAAGTCCAA-3'; backward, 5' CATCCAAGTGCCAGCTAAGA-3'. The glyceraldehyde 3-phosphate dehydrogenase (GAPDH) sequences were as follows: forward, 5'-CAATGACCCCTTCATTGACC-3'; backward, 5'-TGGACTCCACGACGTACTCA-3'.28 The relative quantification method 2-ΔΔCT was applied to detect changes in target samples relative to an internal control in the analysis of the quantitative real-time RT-PCR.29,30 The total quantity of RAGE messenger RNA (mRNA) was assessed by threshold cycle for amplification of the target gene, and GAPDH was used as an internal control gene.29 The amplification efficiencies of both the target and control genes were almost the same (close to 2), validating the calculation method. The amount of RAGE mRNA relative to GAPDH was determined for each sample and divided by the ratio (RAGE/GAPDH) of a calibrator tissue (lung in the present study) to normalize all samples within and between runs.29,30
Correlations were analyzed using Spearman correlation analysis. The Kruskal-Wallis test was used in comparisons involving more than two groups. Fisher's exact test was performed for categorical variables. The cutoff for statistical significance was set at p<0.05. Statistical tests were performed using R (http://www.R-project.org).
The clinical characteristics of the patients are summarized in Table 1, and the results of clinical and histological measures of diabetic neuropathy are given in Table 2. The patients with a greater severity of diabetic neuropathy had a significantly lower IENFD (p<0.001) (Table 2, Fig. 1A). We also found a significant correlation between the Michigan Diabetic Neuropathy Score and IENFD (rho=-0.76, p<0.0001) (Fig. 1B). Immunohistochemical staining revealed that RAGE was localized almost exclusively in the dermal and subcutaneous vascular endothelia, with only slight expression in the basal cells of the epidermis (Fig. 2). The patients with a greater severity of diabetic neuropathy exhibited a higher grading in RAGE immunohistochemical staining (p=0.028) (Table 2).
As illustrated in Fig. 3, IENFD was also significantly lower in patients with a higher intensity of RAGE staining: the median (interquartile range) IENFD values were 6.7 (5.3-8.5), 4.2 (2.3-6.7), 1.9 (1.7-2.0), and 2.3 (0.8-2.9) per mm3 for grades I, II, III, and IV of RAGE immunostaining, respectively (p=0.0037) (Fig. 3). Furthermore, the quantity of mRNA as measured by real-time RT-PCR was significantly higher in patients with severe neuropathy than in those with no or mild neuropathy (p=0.0028); the median relative change relative to GAPDH was 1.06×10-3 (range, 0.51×10-3 to 0.42×10-3) and 5.46 (range, 1.35×10-3 to 0.11) in the group with no and mild neuropathy combined (n=9) and the group with severe neuropathy (n=6), respectively.
The relevance of RAGE expression in terms of nerve-conduction abnormalities was also analyzed; the results are illustrated in Fig. 4. The sural sensory-nerve action potential (SNAP) amplitude and peroneal motor-nerve conduction velocity (MNCV) were the two key electrophysiological parameters used, which are well known to be correlated with characteristic structural lesions of myelinated fibers.23 These nerve-conduction parameters did not differ significantly between the RAGE-immunostaining groups (Kruskal-Wallis rank sum test: p=0.32 for sural SNAP amplitude and p=0.63 for peroneal MNCV). Furthermore, the correlations between these nerve-conduction parameters and RAGE mRNA expression were not significant (Spearman analysis: p=0.19 for sural SNAP amplitude and p=0.07 for peroneal MNCV). However, despite this lack of statistical significance there was a definite trend toward decreased sural SNAP amplitudes and slowed peroneal MNCVs with increasing RAGE expression.
We also examined the correlations between RAGE expression and various metabolic and vascular parameters of diabetic neuropathy; the results are illustrated in Supplementary Fig. 1. Serum triglyceride was significantly higher in groups with mild-to-moderate levels of RAGE immunostaining (Kruskal-Wallis test, p=0.0043). There were also significant correlations between serum cholesterol [total and low-density lipoprotein (LDL)] and RAGE mRNA expression (Spearman analysis: rho=0.64 and p=0.028 for total cholesterol, and rho=0.76 and p=0.007 for LDL). However, we found no significant correlations for other metabolic parameters including fasting and postprandial 2-hour plasma glucose and HbA1c. As for the markers of microvascular complications of diabetes, the up-regulation of RAGE was correlated with a lower estimated glomerular filtration rate (eGFR) and increased 24-hour urine albumin, although these correlations had marginal significance (Spearman analysis: rho=-0.51 and p=0.09 for eGFR, and rho=0.62 and p=0.06 for 24-hour urine albumin). The severity of diabetic retinopathy was not significantly associated with the level of RAGE expression.
The present study found that RAGE immunoreactivity was localized mostly in the dermal and epidermal vascular endothelia. The staining was more intensity in patients with a lower IENFD. We further measured RAGE gene expression, and found that patients with severe neuropathy had higher levels of RAGE mRNA. The relevance of RAGE expression specifically in terms of large-myelinated-fiber pathology was also suggested by the finding of decreased sural SNAP amplitudes and slowed peroneal MNCVs with increasing RAGE, although the statistics did not reach significance. The level of RAGE mRNA was also found to be correlated with markers of diabetic nephropathy. In addition, the up-regulation of RAGE was related to dyslipidemia, which supports an emerging concept that dyslipidemia may play an important role in the progression of diabetic neuropathy.31
Receptor for advanced glycation end products is currently the best-characterized receptor for AGE, and belongs to the immunoglobulin superfamily of cell-surface molecules.32 The up-regulation of RAGE has been suggested to play a crucial role in the development of the microvascular complications of diabetes.33,34 Binding with its ligands is known to trigger downstream intracellular signaling pathways, culminating in NF-κB activation and subsequent transcription of several inflammatory mediators such as endothelin-1, tissue factor, interleukin (IL)-1, IL-6, and tumor necrosis factor-α.35,36,37 NADPH oxidases may be other important mediators activated by an AGE-RAGE interaction, increasing the level of cellular oxidative stress and microangiopathy.38,39 A particularly notable feature is that the RAGE-NF-κB pathway seems to play a similar pathophysiological role in neuronal tissue, leading to neuronal dysfunction and progressive degeneration. Cumulative increases in RAGE mRNA and protein were shown in axons and Schwann cells in diabetic mice, along with up-regulation of NF-κB and protein kinase C pathways.20 RAGE blockade with soluble RAGE or knockout was reported to limit activation of these signaling pathways, and to attenuate the features of neuropathy.17,20
Receptor for advanced glycation end products is known to be expressed in various types of cell. RAGE expression has been demonstrated in the endothelial cells of perineural and endoneurial tissues, epidermal and sural axons, Schwann cells, and dorsal root ganglia sensory neurons in various experimental models of diabetic neuropathy.13,17,20 In sural nerve tissue biopsied from patients with diabetic neuropathy, RAGE was localized in the microvasculature of the peripheral nerve along with activation of NF-κB p65 unit and IL-6.17 To the best of our knowledge, the present study is the first to explore the expression of RAGE in skin biopsy samples obtained from diabetic patients. The localization of RAGE in dermal vascular endothelial cells along with its up-regulation with decreased IENFD observed in the present study suggests a pathogenic role of RAGE in the small-fiber damage associated with diabetic neuropathy. Our results are in line with previous investigations that support microangiopathy as a mechanism underlying the microvascular complications of diabetes.
The potential utility of skin biopsy sampling and analysis could be extended beyond the quantification of IENFD and morphometric analysis. The skin biopsy could be used to determine molecular signatures that may be specific to peripheral neuropathies of various etiologies. RAGE in this study could be a representative example. Recent technical advances in RNA extraction from very small samples makes it possible to apply more sophisticated techniques for high-throughput gene-expression analysis.40 The results of the present study show that quantification of mRNA is feasible even for molecules that are expressed as weakly as RAGE in the skin.
While the number of patients in the present study was too small to enable us to draw robust conclusions, our findings suggest an association between RAGE up-regulation mainly in the vascular endothelial cells of the skin and both the small- and large-fiber components of diabetic neuropathy. These results should be confirmed in a larger study. It would also be interesting to investigate whether the up-regulation of RAGE observed in the present study is specific to diabetic neuropathy.41
Figures and Tables
![]() | Fig. 1Intraepidermal nerve-fiber density (IENFD) in skin biopsy specimens of patients with different clinical severities of diabetic neuropathy. A: Kruskal-Wallis rank sum test, p=0.00017. B: Spearman correlation analysis, rho=-0.76, p<0.0001. |
![]() | Fig. 2Immunohistochemical staining (×200) for receptor for advanced glycation end products (RAGE) in the vascular endothelium (arrowheads) of the superficial dermis (A), deep dermis (B), and subcutaneous region (C) in a patient with severe diabetic neuropathy. Basal cells in the epidermis are marginally stained for RAGE, although it is difficult to distinguish between the melatonin in those cells and the immunoreactivity (A). |
![]() | Fig. 3Intraepidermal nerve-fiber density (IENFD) in skin biopsy specimens of patients with different levels of immunostaining for receptor for advanced glycation end products (RAGE); Kruskal-Wallis rank sum test, p=0.0037 (A). Relative change in RAGE mRNA (relative to glyceraldehyde 3-phosphate dehydrogenase), normalized to the expression in the lung (calibrator tissue), as quantified by real-time reverse-transcription PCR (B). Each bar represents the result for an individual patient. The mean and upper (lower) errors were determined from triplicates of each sample. Wilcoxon rank sum test for comparison of severe vs. no or mild neuropathy, p=0.0028. |
![]() | Fig. 4Relationships between receptor for advanced glycation end products (RAGE) expression and nerve-conduction parameters. Sural sensory-nerve action potential (SNAP) amplitudes (A and B), and peroneal motor-nerve conduction velocities (MNCVs) (C and D). RAGE immunohistochemical staining (A and C), and RAGE reverse-transcription-PCR (B and D). |
Table 1
Clinical characteristics of patients categorized according to the clinical severity of their diabetic neuropathy
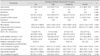
All continuous data are expressed as median (interquartile range) values.
*Significantly different at p<0.05.
BMI: body mass index, DM: diabetic mellitus, eGFR: estimated glomerular filtration rate, FBS: fasting blood sugar, HbA1c: hemoglobin A1c, HDL: high-density lipoprotein, IGT: impaired glucose tolerance, LDL: low-density lipoprotein, MDRD: Modification of Diet in Renal Disease, NPDR: nonproliferative diabetic retinopathy, OHA: oral hypoglycemia agents, PDR: proliferative diabetic retinopathy.
Acknowledgements
This study was supported by a grant from the Korea Healthcare Technology R&D Project, Ministry for Health, Welfare and Family Affairs, Republic of Korea (A090592), a grant no 04-2010-0250 from the Seoul National University Hospital Research Fund, and a clinical research grant-in-aid from the Seoul Metroplitan Government Seoul National University Boramae Medical Center (03-2007-7).
References
1. Callaghan BC, Cheng HT, Stables CL, Smith AL, Feldman EL. Diabetic neuropathy: clinical manifestations and current treatments. Lancet Neurol. 2012; 11:521–534.


2. Gordois A, Scuffham P, Shearer A, Oglesby A, Tobian JA. The health care costs of diabetic peripheral neuropathy in the US. Diabetes Care. 2003; 26:1790–1795.


4. Feldman EL. Oxidative stress and diabetic neuropathy: a new understanding of an old problem. J Clin Invest. 2003; 111:431–433.


5. Geraldes P, King GL. Activation of protein kinase C isoforms and its impact on diabetic complications. Circ Res. 2010; 106:1319–1331.


6. Lauria G, Cornblath DR, Johansson O, McArthur JC, Mellgren SI, Nolano M, et al. EFNS guidelines on the use of skin biopsy in the diagnosis of peripheral neuropathy. Eur J Neurol. 2005; 12:747–758.


7. England JD, Gronseth GS, Franklin G, Carter GT, Kinsella LJ, Cohen JA, et al. Practice Parameter: evaluation of distal symmetric polyneuropathy: role of autonomic testing, nerve biopsy, and skin biopsy (an evidence-based review). Report of the American Academy of Neurology, American Association of Neuromuscular and Electrodiagnostic Medicine, and American Academy of Physical Medicine and Rehabilitation. Neurology. 2009; 72:177–184.


8. Joint Task Force of the EFNS and the PNS. European Federation of Neurological Societies/Peripheral Nerve Society Guideline on the use of skin biopsy in the diagnosis of small fiber neuropathy. Report of a joint task force of the European Federation of Neurological Societies and the Peripheral Nerve Society. J Peripher Nerv Syst. 2010; 15:79–92.
9. Quattrini C, Tavakoli M, Jeziorska M, Kallinikos P, Tesfaye S, Finnigan J, et al. Surrogate markers of small fiber damage in human diabetic neuropathy. Diabetes. 2007; 56:2148–2154.


10. Shun CT, Chang YC, Wu HP, Hsieh SC, Lin WM, Lin YH, et al. Skin denervation in type 2 diabetes: correlations with diabetic duration and functional impairments. Brain. 2004; 127(Pt 7):1593–1605.


11. Polydefkis M, Hauer P, Sheth S, Sirdofsky M, Griffin JW, McArthur JC. The time course of epidermal nerve fibre regeneration: studies in normal controls and in people with diabetes, with and without neuropathy. Brain. 2004; 127(Pt 7):1606–1615.


12. Løseth S, Stålberg E, Jorde R, Mellgren SI. Early diabetic neuropathy: thermal thresholds and intraepidermal nerve fibre density in patients with normal nerve conduction studies. J Neurol. 2008; 255:1197–1202.


13. Wada R, Yagihashi S. Role of advanced glycation end products and their receptors in development of diabetic neuropathy. Ann N Y Acad Sci. 2005; 1043:598–604.


14. Yan SF, Barile GR, D'Agati V, Du Yan S, Ramasamy R, Schmidt AM. The biology of RAGE and its ligands: uncovering mechanisms at the heart of diabetes and its complications. Curr Diab Rep. 2007; 7:146–153.


15. Misur I, Zarković K, Barada A, Batelja L, Milicević Z, Turk Z. Advanced glycation endproducts in peripheral nerve in type 2 diabetes with neuropathy. Acta Diabetol. 2004; 41:158–166.


16. Wautier MP, Chappey O, Corda S, Stern DM, Schmidt AM, Wautier JL. Activation of NADPH oxidase by AGE links oxidant stress to altered gene expression via RAGE. Am J Physiol Endocrinol Metab. 2001; 280:E685–E694.


17. Bierhaus A, Haslbeck KM, Humpert PM, Liliensiek B, Dehmer T, Morcos M, et al. Loss of pain perception in diabetes is dependent on a receptor of the immunoglobulin superfamily. J Clin Invest. 2004; 114:1741–1751.


18. Haslbeck KM, Schleicher E, Bierhaus A, Nawroth P, Haslbeck M, Neundörfer B, et al. The AGE/RAGE/NF-(kappa)B pathway may contribute to the pathogenesis of polyneuropathy in impaired glucose tolerance (IGT). Exp Clin Endocrinol Diabetes. 2005; 113:288–291.


19. Vincent AM, Perrone L, Sullivan KA, Backus C, Sastry AM, Lastoskie C, et al. Receptor for advanced glycation end products activation injures primary sensory neurons via oxidative stress. Endocrinology. 2007; 148:548–558.


20. Toth C, Rong LL, Yang C, Martinez J, Song F, Ramji N, et al. Receptor for advanced glycation end products (RAGEs) and experimental diabetic neuropathy. Diabetes. 2008; 57:1002–1017.


21. Expert Committee on the Diagnosis and Classification of Diabetes Mellitus. Report of the expert committee on the diagnosis and classification of diabetes mellitus. Diabetes Care. 2003; 26:Suppl 1. S5–S20.
22. Wilkinson CP, Ferris FL 3rd, Klein RE, Lee PP, Agardh CD, Davis M, et al. Proposed international clinical diabetic retinopathy and diabetic macular edema disease severity scales. Ophthalmology. 2003; 110:1677–1682.


23. Feldman EL, Stevens MJ, Thomas PK, Brown MB, Canal N, Greene DA. A practical two-step quantitative clinical and electrophysiological assessment for the diagnosis and staging of diabetic neuropathy. Diabetes Care. 1994; 17:1281–1289.


24. Berglund SR, Schwietert CW, Jones AA, Stern RL, Lehmann J, Goldberg Z. Optimized methodology for sequential extraction of RNA and protein from small human skin biopsies. J Invest Dermatol. 2007; 127:349–353.


25. Fleige S, Pfaffl MW. RNA integrity and the effect on the real-time qRT-PCR performance. Mol Aspects Med. 2006; 27:126–139.


26. Chello M, Spadaccio C, Lusini M, Covino E, Blarzino C, De Marco F, et al. Advanced glycation end products in diabetic patients with optimized glycaemic control and their effects on endothelial reactivity: possible implications in venous graft failure. Diabetes Metab Res Rev. 2009; 25:420–426.


27. Ball TB, Plummer FA, HayGlass KT. Improved mRNA quantitation in LightCycler RT-PCR. Int Arch Allergy Immunol. 2003; 130:82–86.


28. Franke S, Sommer M, Rüster C, Bondeva T, Marticke J, Hofmann G, et al. Advanced glycation end products induce cell cycle arrest and proinflammatory changes in osteoarthritic fibroblast-like synovial cells. Arthritis Res Ther. 2009; 11:R136.


29. Livak KJ, Schmittgen TD. Analysis of relative gene expression data using real-time quantitative PCR and the 2(-Delta Delta C(T)) Method. Methods. 2001; 25:402–428.


30. Yuan JS, Reed A, Chen F, Stewart CN Jr. Statistical analysis of real-time PCR data. BMC Bioinformatics. 2006; 7:85.


31. Wiggin TD, Sullivan KA, Pop-Busui R, Amato A, Sima AA, Feldman EL. Elevated triglycerides correlate with progression of diabetic neuropathy. Diabetes. 2009; 58:1634–1640.


32. Neeper M, Schmidt AM, Brett J, Yan SD, Wang F, Pan YC, et al. Cloning and expression of a cell surface receptor for advanced glycosylation end products of proteins. J Biol Chem. 1992; 267:14998–15004.


33. Wendt T, Harja E, Bucciarelli L, Qu W, Lu Y, Rong LL, et al. RAGE modulates vascular inflammation and atherosclerosis in a murine model of type 2 diabetes. Atherosclerosis. 2006; 185:70–77.


34. Soro-Paavonen A, Watson AM, Li J, Paavonen K, Koitka A, Calkin AC, et al. Receptor for advanced glycation end products (RAGE) deficiency attenuates the development of atherosclerosis in diabetes. Diabetes. 2008; 57:2461–2469.


35. Bierhaus A, Illmer T, Kasper M, Luther T, Quehenberger P, Tritschler H, et al. Advanced glycation end product (AGE)-mediated induction of tissue factor in cultured endothelial cells is dependent on RAGE. Circulation. 1997; 96:2262–2271.


36. Quehenberger P, Bierhaus A, Fasching P, Muellner C, Klevesath M, Hong M, et al. Endothelin 1 transcription is controlled by nuclear factor-kappaB in AGE-stimulated cultured endothelial cells. Diabetes. 2000; 49:1561–1570.


37. Schmidt AM, Stern DM. RAGE: a new target for the prevention and treatment of the vascular and inflammatory complications of diabetes. Trends Endocrinol Metab. 2000; 11:368–375.


38. Nishikawa T, Edelstein D, Du XL, Yamagishi S, Matsumura T, Kaneda Y, et al. Normalizing mitochondrial superoxide production blocks three pathways of hyperglycaemic damage. Nature. 2000; 404:787–790.


39. Lin J, Bierhaus A, Bugert P, Dietrich N, Feng Y, Vom Hagen F, et al. Effect of R-(+)-alpha-lipoic acid on experimental diabetic retinopathy. Diabetologia. 2006; 49:1089–1096.


Supplementary Material
Supplementary Fig. 1
Relationships between receptor for advanced glycation end products (RAGE) expression and dyslipidemia (A: TG, B: Total cholesterol, C: LDL) and markers of diabetic nephropathy (D: eGFR, E: Albuminuria). eGFR: estimated glomerular filtration rate, LDL: low-density lipoprotein, MDRD: Modification of Diet in Renal Disease, TG: triglyceride.