Abstract
Background and Purpose
Involvement of the corpus callosum (CC) is reported to be a consistent feature of amyotrophic lateral sclerosis (ALS). We examined the CC pathology using diffusion tensor tractography analysis to identify precisely which fiber bundles are involved in ALS.
Methods
Diffusion tensor imaging was performed in 14 sporadic ALS patients and 16 age-matched healthy controls. Whole brain tractography was performed using the multiple-region of interest (ROI) approach, and CC fiber bundles were extracted in two ways based on functional and structural relevance: (i) cortical ROI selection based on Brodmann areas (BAs), and (ii) the sulcal-gyral pattern of cortical gray matter using FreeSurfer software, respectively.
Results
The mean fractional anisotropy (FA) values of the CC fibers interconnecting the primary motor (BA4), supplementary motor (BA6), and dorsolateral prefrontal cortex (BA9/46) were significantly lower in ALS patients than in controls, whereas those of the primary sensory cortex (BA1, BA2, BA3), Broca's area (BA44/45), and the orbitofrontal cortex (BA11/47) did not differ significantly between the two groups. The FreeSurfer ROI approach revealed a very similar pattern of abnormalities. In addition, a significant correlation was found between the mean FA value of the CC fibers interconnecting the primary motor area and disease severity, as assessed using the revised Amyotrophic Lateral Sclerosis Functional Rating Scale, and the clinical extent of upper motor neuron signs.
Amyotrophic lateral sclerosis (ALS) is a fatal neurodegenerative disease that is characterized by progressive upper and lower motor neuron degeneration.1 However, the pathology of the disease is not limited to the pyramidal motor system, instead involving various extramotor regions to varying degrees.2 The corpus callosum (CC) is of particular interest, with degeneration thereof being specifically noted in a seminal postmortem study as a prominent feature of ALS.3 Mirror movements, which reflect CC motor dysfunction, have been reported in some ALS patients.4 Transcranial magnetic stimulation studies revealed that the earliest impairment of transcallosal inhibition occurred even before the appearance of upper motor neuron signs.5,6 Conventional magnetic resonance imaging (MRI) studies have also revealed atrophy or a high signal intensity of the CC on proton-density and T2-weighted spin-echo images.7,8
Diffusion tensor imaging (DTI) is an established MRI tool that can be used to evaluate white matter pathology by quantifying the diffusion properties of water molecules in vivo. This quantification of the diffusion properties, typically described as fractional anisotropy (FA), has been used to evaluate the neuronal fiber integrity, directional coherence, and tissue microstructure in the brain.9 Studies using DTI in ALS have demonstrated abnormal changes in diffusion properties in the pyramidal and various extramotor regions including the CC.10,11,12,13,14,15 However, alteration of the CC reportedly forms part of the widespread white matter changes in ALS, based on the results of tract-based spatial statistics and voxel-based analysis. Furthermore, the use of different techniques for diffusion tensor tractography and different analysis methods has made it difficult to precisely delineate the specific CC fibers involved in ALS.
In this study we reconstructed the CC fibers connecting different cortical regions of interest (ROIs) in order to assess the CC pathology in ALS patients. We used whole brain seeding-based tractography, which is better than the commonly used regional seeding for overcoming the partial volume effect, and is more robust for constructing fiber tracts that cross and fan out along their paths.16 To extract the fiber tracts of interest (i.e., cortical connections through the CC), a selection process was followed that preserved only those tracts that traverse through the CC and target specific cortical ROIs.16 Two different approaches were adopted to parcellate the different cortical regions: one based on Brodmann areas (BAs) for functional relevance, and the other based on the sulcal-gyral borderlines for anatomical precision.17,18
Fourteen patients with a diagnosis of definite, probable, or laboratory-supported probable ALS using the revised El Escorial criteria were recruited consecutively.19 Patients with history of hypertension, diabetes mellitus, cerebrovascular disease, or other neurological disorders, and who had severe cognitive impairments suggestive of dementia were excluded. Disease severity was measured using the revised Amyotrophic Lateral Sclerosis Functional Rating Scale (ALSFRS-R).20 The number of regions that exhibited definite upper motor neuron signs was counted as described previously [i.e., scored from 0 (control group) to 5 (bulbar and four limbs)].21 Clinical evaluations were performed by a single clinician who was blinded to the brain MRI findings. Sixteen age-matched healthy controls (mean age 52.2 years; range 45-62 years; 3 males, 13 females) were recruited for comparison. All of the patients and control subjects were right-handed. This study was conducted in compliance with the Declaration of Helsinki and ethics approval was provided by the institutional review board of the Seoul National University Hospital. Informed consent to participate was obtained from both the ALS patients and the controls.
Diffusion-weighted images (DWIs) along 25 noncollinear diffusion gradients and baseline images with no diffusion gradient were acquired using a 3.0-T MRI whole-body imaging system (GE, Milwaukee, WI, USA). A double spin-echo planar imaging sequence was employed to minimize unwanted eddy-current-induced distortion of the DWIs. The detailed imaging parameters were as follows: matrix=256×256, field of view=240×240 mm2, axial slice thickness=3.5 mm, and number of excitations=1. All DWIs were realigned onto the B0 images using 12-parameter affine linear transformation. A cubic spine interpolation was conducted to compensate for the effects of anisotropic image voxel size, resulting in an isotropic diffusion image voxel size of 0.94×0.94×0.94 mm3. The diffusion tensor was estimated on a voxel-by-voxel basis using a general logarithm ratio linear least-square fitting method.
We performed a general tractography method called whole brain tractography with a multiple-ROI approach in order to construct the white matter tracts of interest. By placing the seed ROI on the midsagittal CC and the target ROI on the parcellated cortical gray matter, we were able to delineate the CC fibers specific to each cortical gray-matter parcel. The midsagittal ROI was defined using a principal-diffusion-direction-based automated ROI selection method that mimics how human raters define the midsagittal CC ROI using a color-coded DTI map. The details of this procedure are described elsewhere.22
Two distinct approaches were adopted for cortical parcellation in order to cluster the large CC fiber bundles. The first used a BA template, which is a cytoarchitectonic atlas-based cortical map that separates the cerebral cortex according to the cellular composition, whereby each BA is associated with a particular neuropsychological function.23 The second approach is based on a sulcal-gyral segmentation of the cortical gray matter produced by a program called FreeSurfer (version 5.0, http://surfer.nmr.mgh.harvard.edu/).24 For the BA ROI approach, the BA template was projected onto the canonical 3D T1-weighted image of all participants, as in our previous study.18 Our FreeSurfer ROI approach was similar to that of Park et al.,17 in which the FreeSurfer parcellation with the sulcus/gyrus distinction (aparc2009+aseg segmentation in FreeSurfer software) provides a very fine subdivision of the cortical gray matter. However, the resulting small area might be too sensitive, and possibly cause spatial mismatch between the structural image (T1 spoiled gradient-recalled echo) and the DTI. Therefore, we used another version of FreeSurfer parcellation that does not distinguish between sulci and gyri (aparc+ aseg segmentation in FreeSurfer software).
We selected bulky fiber bundles passing through the midsagittal CC ROI from the reconstructed whole brain tracts. An FA threshold of 0.15 was used for both the seeding and the stopping criteria for the fiber tracing; the procedure was stopped when the curvature exceeded 40°/mm. The entire CC tract was then segmented into several parts, with each part being specific to an individual cortical ROI. Fibers containing unwanted noisy portions were removed using a sensitivity tool (part of the Slicer package, http://www.slicer.org) technique that was developed by San José Estépar et al.25
All statistical analyses were performed using SPSS for Windows (ver. 17.0, SPSS Inc., Chicago, IL, USA). Mean±SD values were calculated for each variable. The Mann-Whitney U test was used to compare group differences in the mean FA values of corresponding fiber tracts. Correlations between the diffusion parameters and clinical variables such as the ALSFRS-R score and the clinical extent of upper motor neuron signs were evaluated using Spearman's correlation analysis. The cutoff for statistical significance was set at 5%.
The clinical features of the patients are summarized in Table 1 and 2. All of the ALS patients were taking riluzole and they did not have percutaneous endoscopic gastrostomy or respiratory symptoms at imaging acquisition periods. The fibers passing through the midsagittal CC were extracted from the whole brain using seeding diffusion tensor tractography (Fig. 1A). The CC fibers were then divided into those interconnecting BA4 (the primary motor cortex), BA1/2/3 (the primary sensory cortex), BA6 (the supplementary motor area), BA9/46 (the dorsolateral prefrontal cortex), BA11/47 (the orbitofrontal cortex), and BA44/45 (Broca's area; Fig. 1B, upper panel). The mean FA values of each fiber tract are listed in Table 3. The mean FA value was significantly lower in ALS patients than in controls only for BA4 (p=0.003), BA6 (p=0.01), and BA9/46 (p=0.0005) projections. However, the mean FA values did not differ significantly between ALS patients and control subjects for the fiber tracts interconnecting BA1/2/3, BA11/47, and BA44/45.
With the FreeSurfer cortical ROI approach, the selected cortical regions included the precentral gyrus, postcentral gyrus, and the superior, middle, and ventral frontal cortices (Fig. 1B, lower panel). ALS patients exhibited significantly lower mean FA values than the controls for the fibers interconnecting the precentral gyrus (p=0.0036) and the middle (p=0.016) and superior (p=0.0079) frontal cortices, but not the postcentral gyrus or ventral frontal connections (Table 3).
We also compared the topography of the CC fibers connecting each selected cortical areas using the two different graymatter parcellation methods (Fig. 1C). The CC fibers interconnecting the primary motor cortex (BA4) mostly passed through the isthmus and posterior midbody. Fibers from the primary sensory cortex (BA1/2/3) crossed the isthmus, while the fibers from the supplementary motor area (BA6) passed through the anterior and posterior midbody. Fibers interconnecting the dorsolateral prefrontal cortex (BA9/46) mainly crossed the rostral body, but some also crossed the splenium, and the fibers from the orbitofrontal cortex (BA11/17) were located mostly in the genu and rostrum, but some were also located in the splenium.
The CC topography determined by FreeSurfer cortical ROI selection exhibited a very similar pattern (Fig. 1C, lower panel). The vast majority of fibers from the precentral gyrus passed through the posterior midbody and isthmus, and the fibers from the postcentral gyrus crossed the isthmus. The superior frontal gyri were interconnected via the long CC segment between the genu and the posterior midbody. The middle frontal gyri were interconnected via the rostrum, genu, rostral body, and anterior midbody, while the ventral frontal gyri were connected mostly via the splenium.
Considering all of the healthy control data (assigning their ALSFRS-R score as 48 and the clinical extent of upper motor neuron signs as 0), we found a significant correlation between the ALSFRS-R score and the mean FA values of the fibers interconnecting BA4 and the precentral gyrus (p=0.006 and r=0.50, and p=0.02 and r=0.42, respectively) (Fig. 2A and B). A weak but significant correlation was also found between the ALSFRS-R score and the clinical extent of upper motor neuron signs and mean FA values (p=0.02 and r=-0.42, and p=0.022 and r=-0.42, respectively) (Fig. 2C and D).
Our use of two distinct approaches for cortical parcellation has revealed a preferential involvement of specific CC fiber tracts in patients with ALS. With BA cortical ROI selection, the mean FA values of the fibers interconnecting the BA4 (the primary motor cortex), BA6 (the supplementary motor area), and BA9/46 (the dorsolateral prefrontal cortex) regions were significantly reduced in ALS patients; the FA values for those interconnecting BA1/2/3 (the primary sensory cortex), BA11/47 (the orbitofrontal cortex), and BA 44/45 (Broca's area) did not differ between ALS patients and controls. The FreeSurfer ROI approach revealed a very similar pattern, with a significant reduction in FA in the CC fibers interconnecting the precentral, superior, and middle frontal cortices, but not in those targeting the postcentral and ventral frontal cortices. Furthermore, there was a weak but significant correlation between the mean FA values of the CC fibers interconnecting the primary motor cortex and both disease severity, as assessed with the ALSFRS-R, and the clinical extent of upper motor neuron dysfunction.
The two different methods used for cortical parcellation in this study may provide different data regarding patterns of CC fiber topography and fiber integrity,17,26 and have their own pros and cons. The BA-based ROI approach is considered to be more functionally relevant, while the FreeSurfer method has a higher anatomical precision.27 We expected that the two methods for cortical parcellation would provide a more detailed and complementary view into the topography of the CC fibers and the distribution of ALS pathology than has hitherto been reported.
The CC is the largest white matter tract in the human brain, consisting of more than 200 million axonal fibers that interconnect the cerebral hemispheres.28 Their primary role is the interhemispheric integration of various brain functions, including basic sensorimotor and high-level cognitive integration.29 The involvement of the CC in ALS was first proposed in seminal histologic studies that found numerous degenerating fibers in the CC in almost all areas of ALS brains, although the pathology was most conspicuous in the middle portion of the CC.3 Atrophy of the CC with anterior predominance has been identified in several MRI studies,7,30 while others have found no size differences in patients with ALS.31,32 However, these studies were limited to the midsagittal CC cross-section either grossly or with geometric subdivisions, and thus did not address those CC fibers projecting into different cortical regions. Many recent DTI studies have examined the degeneration of not only the pyramidal but also the various extramotor white matter tracts in ALS.10,11,12,13,14,15,33,34,35 Although the CC appears to be consistently involved in these studies, there have been some discrepancies regarding the precise distribution of the ALS pathology within the CC.10,12,13,14,33,34,35 The midbody of the CC was reported to be affected in the vast majority of studies, whereas involvement of the genu and splenium remains uncertain. This disparity might be explained by the different techniques used for DTI and fiber tractography. The heterogeneity of the disease phenotypes, particularly regarding the dominance of upper motor neuron dysfunction and cognitive and behavioral disturbances, could be another possible explanation. The topography of the CC fibers in the midsagittal CC as found in the present study was consistent with that previous results for healthy subjects.17,26 Furthermore, the results obtained using the two different methods for cortical parcellation were highly consistent, although there were some minor disparities that could probably be attributed to different definitions of the target ROIs and interindividual variability. This supports the robustness of diffusion tensor tractography of the CC fibers, irrespective of the cortical parcellation approach.
The findings of the present study are in line with those of recent studies, suggesting that the involvement of the CC is a consistent feature in ALS.12 From the clinical perspective, the involvement of the CC could be utilized as an imaging marker for cerebral white matter abnormalities in ALS patients. Mirror movement as an indicator of CC dysfunction can be explained by the preferential involvement of CC fibers interconnecting the primary motor cortex.4 Furthermore, there is an emerging concept of prion-like propagation of abnormal proteins in ALS. The CC fibers could be a conduit in the process of interhemispheric spread of pathologies such as phosphorylated transactive 43-kDa transactive response DNA-binding protein.36
There is an interesting notion that ALS may be a disease that originates inadvertently during the process of neocortical evolution in humans. In this hypothesis, the brain regions or circuits recently developed in the evolution of humans are proposed to be specifically vulnerable to the disease.37,38 One phenomenon that supports this hypothesis is the so-called split-hand phenomenon; that is, predominant wasting of the thenar muscles compared to the hypothenar muscles in patients with ALS.39 This phenomenon may be related to the evolutionary acquisition of opposable thumbs in humans. The involvement of the CC appears to underpin this hypothesis. The CC is thought to develop particularly in the process of neocortical evolution of human brains, allowing efficient interhemispheric transfer of information as well as functional specialization of each hemisphere.
Cognitive and behavioral changes are now considered integral features of ALS.40 Up to 15% of ALS patients have concomitant frontotemporal dementia, while a considerable proportion of patients exhibit specific and selective cognitive impairments and behavioral disturbances.41,42 Impairment of attention and executive functions are the most common cognitive deficits,43 while some patients exhibit significant changes in social cognition and language.44 None of the patients in the present study had overt dementia, which could account for the limited extent of CC fiber pathology observed; however, further study is required to confirm this.
Several limitations of the present study should be acknowledged. First, the study population may be too small to allow robust conclusions to be drawn from the findings, although the sample size was comparable to those of previous DTI studies. Second, we did not perform detailed neuropsychological tests. Since cognitive and behavioral changes are commonly found in a considerable proportion of ALS patients, it would be interesting to determine whether the topographic patterns of CC fiber pathology are correlated with specific cognitive and behavioral changes in ALS patients. Third, technical issues such as the noise and partial volume effects need to be overcome to enhance the use of DTI in clinical applications. High-angularresolution diffusion imaging such as Q-ball imaging or any other tractography methods such as stochastic approaches might solve these problems,45,46 although their long acquisition time makes them impractical to apply in the clinical setting.
In conclusion, the CC fibers interconnecting the primary motor and dorsolateral prefrontal cortices may be preferentially involved in ALS. The findings of the present study suggest that there is some degree of selectivity or a gradient in the CC pathology in ALS. The distribution of CC fiber pathology needs to be studied further in ALS patients with wide spectra of cognitive and behavioral deficits.
Figures and Tables
![]() | Fig. 1A: Whole brain seeding-based diffusion tensor tractography of the fibers passing through the midsagittal corpus callosum (CC; top, lateral, and anterior views presented in order). The CC fibers were extracted according to their connection to a specific cortical region in the bilateral hemispheres. B: The target region of interest (ROI) was selected based on Brodmann areas (BAs; upper panel) and on the sulcal-gyral pattern of the cortex using FreeSurfer software (lower panel). C: Midsagittal CC maps of the CC fibers from a single subject. The CC fibers (diameter of 0.2 mm) passing through the midsagittal CC are mapped with different colors, which represent selected cortical regions. Atlases of BAs and FreeSurfer ROIs were adapted from http://en.wikipedia.org/wiki/Brodmann_area and the aparc+aseg segmentation in FreeSurfer software. DLPFC: dorsolateral prefrontal cortex, MFC: middle frontal cortex, OFC: orbitofrontal cortex, PMC: primary motor cortex, PostC: postcentral gyrus, PreC: precentral gyrus, PSC: primary sensory cortex, SFC: superior frontal cortex, SMA: supplementary motor area, VFC: ventral frontal cortex. |
![]() | Fig. 2A-D: Correlations between the mean fractional anisotropy (FA) values of the corpus callosum (CC) fibers interconnecting the primary motor cortex (A and C) and precentral gyri (B and D), and disease severity as measured using the revised Amyotrophic Lateral Sclerosis Functional Rating Scale (ALSFRS-R) (r=0.50 and p=0.006 in A, r=0.42 and p=0.02 in B), and the clinical extent of upper motor neuron (UMN) signs (r=-0.42 and p=0.02 in C, r=-0.42 and p=0.022 in D). BA: Brodmann area. |
Table 3
Mean fractional anisotropy (FA) values of the corpus-callosum fibers interconnecting a specific cortical region of interest (ROI)
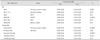
Cortical ROIs were parcellated using two different approaches: one using the Brodmann area (BA) template, and the other based on the sulcal-gyral pattern of cortical gray matter using FreeSurfer software.
DLPFC: dorsolateral prefrontal cortex, NC: normal control, OFC: orbitofrontal cortex, SMA: supplementary motor area.
Acknowledgements
This research was supported by Basic Science Research Program through the National Research Foundation of Korea funded by the Ministry of Education, Science and Technology (20090073877), and Grant no. 09-2007-0020 from the Seoul National University Hospital Research Fund.
References
1. Kiernan MC, Vucic S, Cheah BC, Turner MR, Eisen A, Hardiman O, et al. Amyotrophic lateral sclerosis. Lancet. 2011; 377:942–955.


2. Robberecht W, Philips T. The changing scene of amyotrophic lateral sclerosis. Nat Rev Neurosci. 2013; 14:248–264.


3. Smith MC. Nerve fibre degeneration in the brain in amyotrophic lateral sclerosis. J Neurol Neurosurg Psychiatry. 1960; 23:269–282.


4. Krampfl K, Mohammadi B, Komissarow L, Dengler R, Bufler J. Mirror movements and ipsilateral motor evoked potentials in ALS. Amyotroph Lateral Scler Other Motor Neuron Disord. 2004; 5:154–163.


5. Wittstock M, Wolters A, Benecke R. Transcallosal inhibition in amyotrophic lateral sclerosis. Clin Neurophysiol. 2007; 118:301–307.


6. Karandreas N, Papadopoulou M, Kokotis P, Papapostolou A, Tsivgoulis G, Zambelis T. Impaired interhemispheric inhibition in amyotrophic lateral sclerosis. Amyotroph Lateral Scler. 2007; 8:112–118.


7. Yamauchi H, Fukuyama H, Ouchi Y, Nagahama Y, Kimura J, Asato R, et al. Corpus callosum atrophy in amyotrophic lateral sclerosis. J Neurol Sci. 1995; 134:189–196.


8. Van Zandijcke M, Casselman J. Involvement of corpus callosum in amyotrophic lateral sclerosis shown by MRI. Neuroradiology. 1995; 37:287–288.


9. Beaulieu C. The basis of anisotropic water diffusion in the nervous system - a technical review. NMR Biomed. 2002; 15:435–455.


10. Ciccarelli O, Behrens TE, Johansen-Berg H, Talbot K, Orrell RW, Howard RS, et al. Investigation of white matter pathology in ALS and PLS using tract-based spatial statistics. Hum Brain Mapp. 2009; 30:615–624.


11. Senda J, Ito M, Watanabe H, Atsuta N, Kawai Y, Katsuno M, et al. Correlation between pyramidal tract degeneration and widespread white matter involvement in amyotrophic lateral sclerosis: a study with tractography and diffusion-tensor imaging. Amyotroph Lateral Scler. 2009; 10:288–294.


12. Filippini N, Douaud G, Mackay CE, Knight S, Talbot K, Turner MR. Corpus callosum involvement is a consistent feature of amyotrophic lateral sclerosis. Neurology. 2010; 75:1645–1652.


13. van der Graaff MM, Sage CA, Caan MW, Akkerman EM, Lavini C, Majoie CB, et al. Upper and extra-motoneuron involvement in early motoneuron disease: a diffusion tensor imaging study. Brain. 2011; 134(Pt 4):1211–1228.


14. Iwata NK, Kwan JY, Danielian LE, Butman JA, Tovar-Moll F, Bayat E, et al. White matter alterations differ in primary lateral sclerosis and amyotrophic lateral sclerosis. Brain. 2011; 134(Pt 9):2642–2655.


15. Rose S, Pannek K, Bell C, Baumann F, Hutchinson N, Coulthard A, et al. Direct evidence of intra- and interhemispheric corticomotor network degeneration in amyotrophic lateral sclerosis: an automated MRI structural connectivity study. Neuroimage. 2012; 59:2661–2669.


16. Huang H, Zhang J, van Zijl PC, Mori S. Analysis of noise effects on DTI-based tractography using the brute-force and multi-ROI approach. Magn Reson Med. 2004; 52:559–565.


17. Park HJ, Kim JJ, Lee SK, Seok JH, Chun J, Kim DI, et al. Corpus callosal connection mapping using cortical gray matter parcellation and DT-MRI. Hum Brain Mapp. 2008; 29:503–516.


18. Oh JS, Kubicki M, Rosenberger G, Bouix S, Levitt JJ, McCarley RW, et al. Thalamo-frontal white matter alterations in chronic schizophrenia: a quantitative diffusion tractography study. Hum Brain Mapp. 2009; 30:3812–3825.
19. Brooks BR, Miller RG, Swash M, Munsat TL. World Federation of Neurology Research Group on Motor Neuron Diseases. El Escorial revisited: revised criteria for the diagnosis of amyotrophic lateral sclerosis. Amyotroph Lateral Scler Other Motor Neuron Disord. 2000; 1:293–299.


20. Cedarbaum JM, Stambler N, Malta E, Fuller C, Hilt D, Thurmond B, et al. BDNF ALS Study Group (Phase III). The ALSFRS-R: a revised ALS functional rating scale that incorporates assessments of respiratory function. J Neurol Sci. 1999; 169:13–21.


21. Hong YH, Lee KW, Sung JJ, Chang KH, Song IC. Diffusion tensor MRI as a diagnostic tool of upper motor neuron involvement in amyotrophic lateral sclerosis. J Neurol Sci. 2004; 227:73–78.


22. Kubicki M, Shenton ME, Salisbury DF, Hirayasu Y, Kasai K, Kikinis R, et al. Voxel-based morphometric analysis of gray matter in first episode schizophrenia. Neuroimage. 2002; 17:1711–1719.


23. Brodmann K, Garey L. Brodmann's Localisation in the Cerebral Cortex: the Principles of Comparative Localisation in the Cerebral Cortex Based on the Cytoarchitectonics. 3rd ed. New York: Springer;2006.
24. Fischl B, van der Kouwe A, Destrieux C, Halgren E, Ségonne F, Salat DH, et al. Automatically parcellating the human cerebral cortex. Cereb Cortex. 2004; 14:11–22.


25. San José Estépar R, Kubicki M, Shenton M, Westin CF. A kernel-based approach for user-guided fiber bundling using diffusion tensor data. Conf Proc IEEE Eng Med Biol Soc. 2006; 1:2626–2629.
26. Chao YP, Cho KH, Yeh CH, Chou KH, Chen JH, Lin CP. Probabilistic topography of human corpus callosum using cytoarchitectural parcellation and high angular resolution diffusion imaging tractography. Hum Brain Mapp. 2009; 30:3172–3187.


27. Hagmann P, Cammoun L, Gigandet X, Gerhard S, Grant PE, Wedeen V, et al. MR connectomics: Principles and challenges. J Neurosci Methods. 2010; 194:34–45.


28. Aboitiz F, Scheibel AB, Fisher RS, Zaidel E. Fiber composition of the human corpus callosum. Brain Res. 1992; 598:143–153.


29. van der Knaap LJ, van der Ham IJ. How does the corpus callosum mediate interhemispheric transfer? A review. Behav Brain Res. 2011; 223:211–221.


30. Kassubek J, Unrath A, Huppertz HJ, Lulé D, Ethofer T, Sperfeld AD, et al. Global brain atrophy and corticospinal tract alterations in ALS, as investigated by voxel-based morphometry of 3-D MRI. Amyotroph Lateral Scler Other Motor Neuron Disord. 2005; 6:213–220.


31. Grosskreutz J, Kaufmann J, Frädrich J, Dengler R, Heinze HJ, Peschel T. Widespread sensorimotor and frontal cortical atrophy in Amyotrophic Lateral Sclerosis. BMC Neurol. 2006; 6:17.


32. Ellis CM, Suckling J, Amaro E Jr, Bullmore ET, Simmons A, Williams SC, et al. Volumetric analysis reveals corticospinal tract degeneration and extramotor involvement in ALS. Neurology. 2001; 57:1571–1578.


33. Agosta F, Pagani E, Rocca MA, Caputo D, Perini M, Salvi F, et al. Voxel-based morphometry study of brain volumetry and diffusivity in amyotrophic lateral sclerosis patients with mild disability. Hum Brain Mapp. 2007; 28:1430–1438.


34. Bartels C, Mertens N, Hofer S, Merboldt KD, Dietrich J, Frahm J, et al. Callosal dysfunction in amyotrophic lateral sclerosis correlates with diffusion tensor imaging of the central motor system. Neuromuscul Disord. 2008; 18:398–407.


35. Douaud G, Filippini N, Knight S, Talbot K, Turner MR. Integration of structural and functional magnetic resonance imaging in amyotrophic lateral sclerosis. Brain. 2011; 134(Pt 12):3470–3479.


36. Brettschneider J, Del Tredici K, Toledo JB, Robinson JL, Irwin DJ, Grossman M, et al. Stages of pTDP-43 pathology in amyotrophic lateral sclerosis. Ann Neurol. 2013; 74:20–38.


37. Eisen A. Amyotrophic lateral sclerosis-Evolutionary and other perspectives. Muscle Nerve. 2009; 40:297–304.


38. Eisen A, Turner MR, Lemon R. Tools and talk: an evolutionary perspective on the functional deficits associated with amyotrophic lateral sclerosis. Muscle Nerve. 2014; 49:469–477.


39. Eisen A, Kuwabara S. The split hand syndrome in amyotrophic lateral sclerosis. J Neurol Neurosurg Psychiatry. 2012; 83:399–403.


40. Geser F, Martinez-Lage M, Kwong LK, Lee VM, Trojanowski JQ. Amyotrophic lateral sclerosis, frontotemporal dementia and beyond: the TDP-43 diseases. J Neurol. 2009; 256:1205–1214.


41. Witgert M, Salamone AR, Strutt AM, Jawaid A, Massman PJ, Bradshaw M, et al. Frontal-lobe mediated behavioral dysfunction in amyotrophic lateral sclerosis. Eur J Neurol. 2010; 17:103–110.


42. Phukan J, Elamin M, Bede P, Jordan N, Gallagher L, Byrne S, et al. The syndrome of cognitive impairment in amyotrophic lateral sclerosis: a population-based study. J Neurol Neurosurg Psychiatry. 2012; 83:102–108.


43. Phukan J, Pender NP, Hardiman O. Cognitive impairment in amyotrophic lateral sclerosis. Lancet Neurol. 2007; 6:994–1003.


44. Meier SL, Charleston AJ, Tippett LJ. Cognitive and behavioural deficits associated with the orbitomedial prefrontal cortex in amyotrophic lateral sclerosis. Brain. 2010; 133:3444–3457.

