Abstract
Background
Approximately 90%~99% of ultraviolet A (UVA) ray reaches the Earth's surface. The deeply penetrating UVA rays induce the formation of reactive oxygen species (ROS), which results in oxidative stress such as photoproducts, senescence, and cell death. Thus, UVA is considered a primary factor that promotes skin aging.
Objective
Researchers investigated whether pretreatment with ferulic acid protects human dermal fibroblasts (HDFs) against UVA-induced cell damages.
Methods
HDF proliferation was analyzed using the water-soluble tetrazolium salt assay. Cell cycle distribution and intracellular ROS levels were assessed by flow cytometric analysis. Senescence was evaluated using a senescence-associated β-galactosidase assay, while Gadd45α promoter activity was analyzed through a luciferase assay. The expression levels of superoxide dismutase 1 (SOD1), catalase (CAT), xeroderma pigmentosum complementation group A and C, matrix metalloproteinase 1 and 3, as well as p21 and p16 were measured using quantitative real-time polymerase chain reaction.
Results
Inhibition of proliferation and cell cycle arrest were detected in cells that were irradiated with UVA only. Pretreatment with ferulic acid significantly increased the proliferation and cell cycle progression in HDFs. Moreover, ferulic acid pretreatment produced antioxidant effects such as reduced DCF intensity, and affected SOD1 and CAT mRNA expression. These effects were also demonstrated in the analysis of cell senescence, promoter activity, expression of senescent markers, and DNA repair.
Solar radiation in the form of ultraviolet (UV) light is divided into three types; ultraviolet A (UVA, 320~400 nm), ultraviolet B (UVB, 280~320 nm), and ultraviolet C (UVC, 200~280 nm)1. However, terrestrial solar UV radiation is comprised mostly of UVA and partially of UVB because wavelengths shorter than 295 nm are blocked by the ozone layer in the stratosphere2. Although UVA photons are about 1,000 times less energetic than UVB, they can still penetrate the skin to cause skin aging in the dermis by regulating the extracellular matrix (ECM)345. Furthermore, UVA has been reported to produce reactive oxygen species (ROS) which cause oxidative stress, leading to cell death67. Therefore, UVA is one of the major factors of photoaging in skin.
As the largest organ of the human body, skin surrounds and protects our bodies from the external environment1348. Skin is composed of two layers, namely the epidermis and dermis layer, which is composed of connective tissue including fibroblasts, matrix proteins, and other substances. Studies have shown that human skin fibroblasts are the main components of the dermis and are more receptive to UVA exposure than keratinocytes, resulting in skin reconstruction9. This is one symptom of photoaging, which is caused by UVA photons and UVA-induced ROS in the fibroblasts1011. More interestingly, UVA has also been reported to stimulate fibroblasts to synthesize metalloproteinase 1 (MMP1), which degrades dermal collagen1012. Due to the diverse function of skin biology, fibroblasts have been studied mainly to understand the pathology following exposure to toxins, chemicals, and cosmetics. Therefore, it is necessary to find safe and effective natural products for human skin protection.
Ferulic acid (4-hydroxy-3-methoxycinnamic acid) is widely present in fruits, vegetables, and grains. According to previous studies, ferulic acid has antioxidant and anticancer properties131415. Ferulic acid has been shown to impart beneficial effects in diabetes, Alzheimer's disease, and cardiovascular disease by regulating antioxidant enzyme and caspase activities, COX-2, and hypertension161718. In the skin, ferulic acid has been shown to have a protective effect on UVB-induced erythema19. The current study is aimed at investigating how ferulic acid protects human dermal fibroblasts (HDFs) against UVA radiation.
Normal human dermal fibroblasts (nHDF; Lonza, Basel, Switzerland) were cultured in Dulbecco's modified Eagle medium (DMEM; Gibco/Life Technologies, Carlsbad, CA, USA) supplemented with 10% fetal bovine serum (FBS; Sigma-Aldrich, St. Louis, MO, USA) and 1% penicillin/streptomycin (Gibco/Life Technologies) at 37℃ in an atmosphere of 5% CO2. Ferulic acid was purchased from Sigma-Aldrich and dissolved in dimethyl sulfoxide.
The HDFs (1×106/well) were seeded into 6-well plates and cultured until 80%~90% confluent. Prior to irradiation, cells were washed twice with phosphate buffered saline (PBS). The cells were irradiated with UVA light (UVA lamp; UVP, Upland, CA, USA) in fresh PBS. The radiation intensity was monitored by a fiberoptic spectrometer system USB2000 (Ocean optics, Dunedin, FL, USA). Control cells were treated identically but were not exposed to UVA irradiation. After UVA radiation, fresh medium was added to the cells and the cells were incubated at 37℃ for 24 h.
HDF cell toxicity due to ferulic acid was evaluated using a water-soluble tetrazolium salt (WST-1) assay (EZ-Cytox Cell Viability Assay kit; Itsbio, Seoul, Korea). HDF cells were seeded at a density of 3×103 cells into 96-well plates and incubated for 24 h. Then, the cells were incubated with ferulic acid (0~50 µM) for 24 h. A WST-1 solution was added to cultured cells, which were then incubated at 37℃ for 1 h. Cell viability was evaluated by measuring the absorbance at 450 nm using an iMark microplate reader (Bio-Rad, Hercules, CA, USA). In order to examine the protective effects of ferulic acid against UVA, nHDFs were pretreated with FA for 6 h, and then the cells were washed with PBS followed by UVA irradiation. After UVA irradiation, PBS was replaced with normal growth medium and incubated 24 h. After incubation, WST-1 assay was performed to analyze the UVA protection effect of FA on cell viability of nHDFs.
Cells were collected and fixed in cold 70% ethanol at 4℃ for 1 h. Then the fixed cells were stained by incubation with a propidium iodide (PI) staining solution (50 µg/ml PI, 0.5% Triton X-100 [both from Sigma-Aldrich] plus 100 µg/ml RNase) at 37℃ for 1 h. Changes in cell cycle were determined based on the intensity of fluorescent PI staining of 10,000 cells using the FL2-H channel of a FACSCalibur flow cytometer (BD Biosciences, San Jose, CA, USA)20.
The Gadd45α promoters were cloned into pGL3 to activate the firefly luciferase reporter vector (Promega, Madison, WI, USA). HDFs seeded into 96-well plates were cotransfected with pGL3-Gadd45α together with the pSV-β-galactosidase plasmid (Promega) using Lipofectamine 2000 reagent (Invitrogen/Life Technologies, Carlsbad, CA, USA). At 24 h post-transfection, cells were exposed to UVA radiation with or without ferulic acid treatment. At 24 h post-UVA radiation, cells were lysed using Passive lysis buffer (Promega). Luciferin was then added and the luciferase activity of each cell lysate was analyzed using a Veritas Luminometer (Turner Designs, Sunnyvale, CA, USA). The luciferase signal was normalized to β-galactosidase activity and is presented as a percentage of the control with standard deviation (SD). Results shown represent the average of three independent experiments.
Intracellular ROS scavenging assays were performed by measuring the fluorescence intensity of the 2',7'-dichlorofluroescein diacetate (DCF-DA) probe, which was proportional to the amount of ROS produced. Cells pre-treated with and without ferulic acid were irradiated with UVA prior to harvest. The cells were then mixed with DCF-DA solution and incubated at 37℃ for 1 h. Fluorescence intensity was measured using a BD FACSCalibur flow cytometer (BD Biosciences).
β-galactosidase expression was used as a marker for senescence in HDFs. Expression levels were determined using the senescence-associated-β-galactosidase staining kit (Biovision, Milpitas, CA, USA) according to the manufacturer's protocol. HDFs were seeded at a density of 1×106 cells/well in 60-mm cell culture plates and incubated until cells reached 90% confluence. Then cells were pretreated with 10 or 20 µM ferulic acid for 6 h before irradiation with 10 J/cm2 UVA. After UVA radiation, the cells were washed with fresh media and incubated for another 24 h. To evaluate cellular senescence, the cells were washed in PBS prior to incubation in 0.5 ml fixing solution (4% formaldehyde, 0.5% glutaraldehyde in PBS buffer, pH 7.2) for 1 h. Fixed cells were then incubated in a staining solution mixture (staining solution [470 µl], staining supplement [5 µl], 20 mg/ml X-Gal in dimethylformamide [25 µl]) for 24 h at 37℃. Finally, 70% glycerol (1 ml) was added to the cells and images were captured using an Olympus IX51 microscope (Olympus, Tokyo, Japan).
Total RNA was isolated using the TRIzol reagent (Life Technologies) according to the manufacturer's protocol. RNA purity and concentration were evaluated using the MaestroNano® microspectrophotometer (Maestrogen, Las Vegas, NV, USA). All cDNAs for sensitive and specific miRNA detection were synthesized using the miScript II RT kit (Qiagen, Hilden, Germany) according to the manufacturer's protocol. To evaluate the expression of p21 (forward primer: 5'-GTCCAGCGACCTTCCTCATCCA-3', reverse primer: 5'-CCATAGCCTACTGCCACCATC-3'), p16 (forward primer: 5'-CCCAACGCCCCGAACT-3', reverse primer: 5'-GCAGAAGAGCTGCTACGTGAA-3'), SOD1 (forward primer: 5'-GGGAGATGGCCCAACTACTG-3', reverse primer: 5'-CCAGTTGACATGCAACCGTT-3'), MMP1 (forward primer: 5'-TCTGACGTTGATCCCAGAGAGCAG-3', reverse primer: 5'-CAGGGTGACACCAGTGACTGCAC-3'), MMP3 (forward primer: 5'-ATTCCATGGAGCCAGGCTTTC-3', reverse primer: 5'-CATTTGGGTCAAACTCCAACTGTG-3'), xeroderma pigmentosum complementation group A (XPA, forward primer: 5'-CCAGGACCTGTTATGGAAT TTGA-3', reverse primer: 5'-GCTTCTTGACTACCCCAAA CTTC-3'), xeroderma pigmentosum complementation group C (XPC, forward primer: 5'-AGCAGCTTCCCACC TGTTC-3', reverse primer: 5'-GTGGGTGCCCCTCTAGTG-3'), and CAT (forward primer: 5'-TGGAGCTGGTAACCCA GTAGG-3', reverse primer: 5'-CCTTTGCCTTGGAGTATT TGGTA-3'), quantitative real-time polymerase chain reaction (RT-PCR) was performed using EvaGreen dye (Solis BioDyne, Tartu, Estonia) and Line-Gene K software (BioER, Hangzhou, China). The CT value for each gene was normalized to that of β-actin (forward primer: 5'-GGATTCCTATGTGGGCG ACGA-3', reverse primer: 5'-CGCTCGGTGAGGATCTTCA TG-3'). The 2-ΔΔCt method was used to calculate the relative expression level of each gene21.
Analysis was performed using SPSS for Windows ver. 21.0 (SPSS Inc., Chicago, IL, USA). All results are presented as the mean percentage±SD of three independent experiments. Differences with a p-value less than 0.05, as determined by the Student's t-test, were considered statistically significant.
Before evaluating the protective effects of ferulic acid in UVA-induced cell death and inhibition of proliferation, we examined the cytotoxicity of ferulic acid in HDFs. The cells were treated with ferulic acid (0~50 µM) for 24 h, and viability was determined using the WST-1 assay (Fig. 1A). Cell viability was greater than 90% at ferulic acid concentrations below 50 µM concentration. To determine whether ferulic acid affects the viability of HDFs undergoing UVA-induced cellular stress, cultured cells were irradiated with various intensities of UVA (0~20 J/cm2) and incubated for 24 h. As shown in Fig. 1B, HDF viability decreased drastically after UVA radiation. However, pretreatment with 20 µM ferulic acid exhibited a protective effect up to 10 J/cm2 (Fig. 1B). This protective effect of ferulic acid on UVA-induced cell death was dose-dependent at 10 J/cm2 UVA intensity (Fig. 1C).
We investigated the effects of ferulic acid on cell cycle progression via PI staining followed by flow cytometric analysis. Our data demonstrate that treatment with 10 J/cm2 of UVA led to G1-phase arrest, and that pretreatment with ferulic acid protected cell cycle progression in a dose-dependent manner. Cells treated with UVA only exhibited significantly increased G1-phase cells (up to 67.9%) relative to untreated cells. This increase in G1-phase cells was reduced by pretreatment with ferulic acid at the indicated concentrations (Fig. 2A). Next, we examined Gadd45α expression using a promoter luciferase activity assay. Gadd45α, a stress-inducible gene, has been implicated in cell cycle arrest, apoptosis, and DNA repair2223. HDFs treated with UVA only showed increased luciferase activity (up to 4.7-fold) compared with untreated cells. However, cells pretreated with ferulic acid for 6 h before UVA radiation exhibited significantly decreased Gadd45α luciferase activity in a dose-dependent manner (Fig. 2B). p21 has been reported to be a key regulator of G1- and G2-phase arrest, as well as aging and photoaging2425. In addition, p21 is involved in Gadd45α as cell cycle regulation2226. Therefore, we examined the relative expression of p21 mRNA in ferulic acid-pretreated HDFs irradiated with UVA using quantitative RT-PCR. As shown in Fig. 2C, cells only irradiated with UVA (10 J/cm2) displayed a greater than 5-fold increase in p21 mRNA expression relative to non-irradiated cells. However, cells pretreated 10 and 20 µM ferulic acid before UVA radiation showed a 4.8 (±0.5)- and 2.7 (±0.2)-fold increase, respectively. We also assessed the expression of DNA repair genes in HDFs following UVA radiation and ferulic acid pretreatment. XPA and XPC are core nucleotide excision repair (NER) factors expressed during UV-induced DNA lesion repair27. Studies have also reported that photolyase enzymes such as XPA and XPC can repair UV-induced DNA lesions efficiently28293031. Our data demonstrate that UVA irradiation reduced the expression of both XPA and XPC, whereas cells pretreated with ferulic acid showed significantly increased expression of both genes (Fig. 2D). These results demonstrate that 10 J/cm2 UVA radiation resulted in G1-phase cell cycle arrest and DNA damage in HDFs, but that pretreatment with ferulic acid inhibited UVA-induced cell cycle arrest and DNA damage by regulating gene expression associated with cell cycle progression and DNA repair in a dose-dependent manner.
UVA induces intracellular ROS production that results in oxidative stress and DNA damage in cells323334. Thus, we evaluated the ROS scavenging effects of ferulic acid by analyzing DCF-DA fluorescent intensity. As shown in Fig. 3A, formation of intracellular ROS increased following radiation with 10 J/cm2 UVA. The increased intracellular ROS production was decreased by pretreatment with 10 and 20 µM of ferulic acid. Interestingly, treatment with N-acetylcysteine (NAC), a ROS scavenger used as a positive control, showed no significant scavenging effects at 20 µM, which was the same concentration of ferulic acid used. Furthermore, we investigated the antioxidant effects of ferulic acid by relative gene expression analysis using qRT-PCR. Superoxide dismutases (SODs) and catalase (CAT) have been reported as the main enzymes involved in antioxidant defense against oxidative stress35. In this experiment, cells, irradiated UVA alone, showed a significant alteration in both SOD1 and CAT mRNA expression. Alterative SOD1 mRNA expression was increased and indicated 0.4 (±0.08)- and 0.8 (±0.04)- fold changes, by pretreatment with 10 and 20 µM of ferulic acid (Fig. 3B). The CAT mRNA expression was also increased by pretreatment with 10 and 20 µM of ferulic acid, respectively (Fig. 3C). These results verify that Ferulic acid pretreatment resulted in partial restoration of SOD1 and CAT mRNA level in a dose-dependent manner.
UVA is widely accepted as a trigger of photoaging by regulating ECM components such as MMPs and procollagens3637. Therefore, in this study, we examined the relative expression of p16 mRNA in ferulic acid-pretreated HDFs irradiated with UVA using quantitative real-time PCR. p16 gene expression levels also changed under these conditions. As shown in Fig. 4A, relative p16 mRNA expression was increased in UVA-irradiated cells (4.3±0.7 fold) and was reduced by ferulic acid in a dose-dependent manner. According to previous reports, UVA radiation can stimulate gene expression related with cell cycle arrest3839. p16 are also known as cellular senescence markers40, leading us to examine cellular senescence using a senescence-associated-β-galactosidase staining kit (BioVision). Pretreatment of cells with ferulic acid (10 and 20 µM) before UVA radiation resulted in significantly reduced cellular senescence (38.2% and 19.6%, respectively) compared with cells treated with UVA radiation only (61.2%, Fig. 4B). Further investigation showed that pretreatment with ferulic acid regulated MMP1 and MMP3 gene expression in HDFs. As shown in Fig. 4C, UVA irradiation alone increased MMP1 and MMP3 mRNA expression, whereas pretreatment of ferulic acid prior to UVA radiation reduced expression. These results demonstrate the effects of ferulic acid in UVA-induced cellular senescence and reprogramming of ECM components.
In this study, we investigated the protective effects of ferulic acid on UVA-induced proliferation, oxidation, senescence, and DNA damage in HDFs. Phenolic compounds in the plant are some of the most common water-soluble antioxidant compounds. Ferulic acid, one phenolic compound derived from cereal grains and vegetables, has been widely studied in biological aspects. Previous studies have demonstrated in skin biology that ferulic acid protects UVB damages41.
Dermal fibroblasts, which mainly comprise the dermis layer of the skin, are established to contribute to the generation of connective tissues4243. During skin aging, MMPs, which are generated from dermal fibroblasts, can degrade all components in the ECM, including collagen, elastin, laminin, and proteoglycans44.
Results from our study indicate that pretreatment with ferulic acid decreases cell cycle arrest and DNA damage in HDFs. We verified that ferulic acid reduces Gadd45α promoter activity, cell cycle arrest, and expression of DNA damage markers in a dose-dependent manner. Following analysis of Gadd45α promoter activity, we investigate gene expression of key regulator of cell cycle progression, p21. After then, we verified the effects of ferulic acid on NER by assessing XPA and XPC expression. Interestingly, expression of both XPA and XPC was significantly increased by pretreatment with ferulic acid. These findings suggest the protective effects of ferulic acid on UVA-induced cell cycle arrest and DNA damage in HDFs. Also, further studies are helpful to confirm the Gadd45α-dependent UVA protective effect of Ferulic acid in Gadd45α siRNA-mediated knockdown nHDFs.
Consistent with previous studies1415, we demonstrated the antioxidant effects of ferulic acid against UVA radiation by analyzing intracellular ROS levels and relative expression of SOD1 and CAT. In this study, ferulic acid shows properties about regulation of antioxidant gene expression and ROS scavenging effects. A previous study suggested that formation of intracellular ROS is the main cause of senescence and skin aging. Thus, we examined senescence marker expression and the frequency of senescent cells. Our data show that UVA radiation increased the expression of senescence marker, namely p16, and that marker is significantly decreased in ferulic acid-treated cells along with the frequency of senescent cells. These results suggest the inhibitory effects of ferulic acid against UVA-induced cellular senescence in dermal fibroblasts. Furthermore, UVA has been established as a factor that induces a decrease in type I collagen and inhibition of pro-collagen synthesis in dermal fibroblasts454647. Our results for MMP1 and MMP3 mRNA expression strongly suggest that ferulic acid not only regulates cellular senescence, but also reconstructs the ECM.
In conclusion, ferulic acid was found to regulate antioxidation, senescence, and DNA damage mechanisms through Gadd45α signaling pathways. Ferulic acid downregulates MMP1 and MMP3 gene expression, thereby demonstrating the protective effects of ferulic acid on the dermis layer through ECM reconstruction. These findings indicate that ferulic acid can reverse the effects of aging in the skin by modulating its physiological structure, suggesting that ferulic acid is a potentially useful component for various cosmetic uses.
Figures and Tables
Fig. 1
Effects of ferulic acid on human dermal fibroblast (HDF) cell proliferation. HDF cells (3×103 cells/well) were seeded into 96-well plates and treated with the indicated concentrations of ferulic acid for 24 h. Proliferation was measured using the water-soluble tetrazolium salt assay. (A) Cell viability following ferulic acid treatment in HDFs. (B) Protective effects of treatment with 20 µM ferulic acid against cytotoxicity induced by varying intensities of ultraviolet A (UVA) radiation in HDFs. Cells were pretreated with ferulic acid for 6 h prior to UVA radiation. (C) Protective effects of ferulic acid treatment against 10 J/cm2 UVA radiation. Cells were treated with the indicated concentration of ferulic acid for 6 h before UVA radiation. *p<0.05 by Student's t-test.
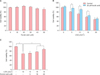
Fig. 2
Effects of ferulic acid on ultraviolet A (UVA)-induced cell cycle arrest and DNA repair in human dermal fibroblasts (HDFs). (A) UVA-induced cell cycle arrest was reduced by ferulic acid. Cell cycle progression was evaluated by propidium iodide staining followed by flow cytometric analysis. (B) UVA-induced upregulation of Gadd45α promoter activity was decreased by ferulic acid. The Gadd45α promoter regions were cloned into the pGL3 luciferase reporter vector and then transfected into HDFs. Luciferase intensity was normalized against β-galactosidase activity. (C) Relative gene expression of p21 in human dermal fibroblasts (HDFs). HDFs were irradiated with ultraviolet A (UVA) followed by ferulic acid. (D) Ferulic acid enhanced mRNA expression of nucleotide excision repair (NER) factors, xeroderma pigmentosum complementation group A (XPA) and xeroderma pigmentosum complementation group C (XPC). Pretreatment with ferulic acid promoted NER-associated genes expression. Gene expression was normalized against β-actin and the 2−ΔΔCt method was used to calculate the relative expression level. Results are expressed as the mean±standard deviation in triplicate. *p<0.05 by Student's t-test.
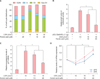
Fig. 3
Effects of ferulic acid on ultraviolet A (UVA)-induced oxidative stress in human dermal fibroblasts (HDFs). (A) Ferulic acid scavenged UVA-induced upregulation of intracellular reactive oxygen species (ROS) production. The 2',7'-dichlorofluroescein diacetate (DCF-DA) probe was used to investigate intracellular ROS levels and 20 µM N-acetylcysteine (NAC) was used as a positive control for ROS scavenger. (B, C) Ferulic acid enhanced antioxidant expression of superoxide dismutase 1 (SOD1) and catalase (CAT). Relative gene expression of antioxidant, SOD1 and CAT, in ferulic acid pretreated cells. Gene expression was normalized against β-actin and the 2−ΔΔCt method was used to calculate the relative expression level. Results are expressed as the mean±standard deviation in triplicate. *p<0.05 by Student's t-test.
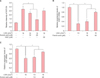
Fig. 4
Effects of ferulic acid on cellular senescence and extracellular matrix reconstruction. (A) Relative gene expression of p16, decreased with ferulic acid treatement prior to ultraviolet A (UVA) radiation. (B) Ferulic acid prohibited senescence in human dermal fibroblasts (HDFs) irradiated with UVA. The senescent cell population was analyzed using the senescence-associated β-galactosidase staining kit. (C) Relative gene expression of metalloproteinase 1 (MMP1) and MMP3 in HDFs. HDFs were irradiated with UVA followed by ferulic acid. Gene expression of MMP1 and MMP3 was reduced by feruilc acid pretreatment. Results are expressed as the mean±standard deviation in triplicate. *p<0.05 by Student's t-test.
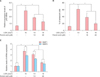
ACKNOWLEDGMENT
This study was supported by grants from the Ministry of Science, ICT and Future Planning (grant no. 2013R1A1A 1012205 to S. Bae and 20110028646 to S. An) of Republic of Korea.
References
1. Lee JJ, An S, Kim KB, Heo J, Cho DH, Oh HM, et al. Extract of ettlia sp. YC001 exerts photoprotective effects against UVB irradiation in normal human dermal fibroblasts. J Microbiol Biotechnol. 2016; 26:775–783.


2. Faurschou A. Role of tumor necrosis factor-α in the regulation of keratinocyte cell cycle and DNA repair after ultraviolet-B radiation. Dan Med Bull. 2010; 57:B4179.
3. Bae S, Kim K, Cha HJ, Choi Y, Shin SH, An IS, et al. Low-dose γ-irradiation induces dual radio-adaptive responses depending on the post-irradiation time by altering microRNA expression profiles in normal human dermal fibroblasts. Int J Mol Med. 2015; 35:227–237.


4. Bae S, An IS, An S. Development of a high-throughput screening system for identification of novel reagents regulating DNA damage in human dermal fibroblasts. Acta Pharm. 2015; 65:331–341.


5. Mac-Mary S, Sainthillier JM, Jeudy A, Sladen C, Williams C, Bell M, et al. Assessment of cumulative exposure to UVA through the study of asymmetrical facial skin aging. Clin Interv Aging. 2010; 5:277–284.
6. Saito Y, Tsuruma K, Ichihara K, Shimazawa M, Hara H. Brazilian green propolis water extract up-regulates the early expression level of HO-1 and accelerates Nrf2 after UVA irradiation. BMC Complement Altern Med. 2015; 15:421.


7. Bossi O, Gartsbein M, Leitges M, Kuroki T, Grossman S, Tennenbaum T. UV irradiation increases ROS production via PKCdelta signaling in primary murine fibroblasts. J Cell Biochem. 2008; 105:194–207.


8. Sjerobabski-Masnec I, Situm M. Skin aging. Acta Clin Croat. 2010; 49:515–518.
9. Zhou BR, Yin HB, Xu Y, Wu D, Zhang ZH, Yin ZQ, et al. Baicalin protects human skin fibroblasts from ultraviolet A radiation-induced oxidative damage and apoptosis. Free Radic Res. 2012; 46:1458–1471.


10. Lan CC, Ho PY, Wu CS, Yang RC, Yu HS. LED 590 nm photomodulation reduces UVA-induced metalloproteinase-1 expression via upregulation of antioxidant enzyme catalase. J Dermatol Sci. 2015; 78:125–132.


11. Vile GF, Tyrrell RM. Oxidative stress resulting from ultraviolet A irradiation of human skin fibroblasts leads to a heme oxygenase-dependent increase in ferritin. J Biol Chem. 1993; 268:14678–14681.


12. Varani J, Spearman D, Perone P, Fligiel SE, Datta SC, Wang ZQ, et al. Inhibition of type I procollagen synthesis by damaged collagen in photoaged skin and by collagenase-degraded collagen in vitro. Am J Pathol. 2001; 158:931–942.


13. Janicke B, Hegardt C, Krogh M, Onning G, Akesson B, Cirenajwis HM, et al. The antiproliferative effect of dietary fiber phenolic compounds ferulic acid and p-coumaric acid on the cell cycle of Caco-2 cells. Nutr Cancer. 2011; 63:611–622.


14. Xu X, Xiao H, Zhao J, Zhao T. Cardioprotective effect of sodium ferulate in diabetic rats. Int J Med Sci. 2012; 9:291–300.


15. Roy S, Metya SK, Sannigrahi S, Rahaman N, Ahmed F. Treatment with ferulic acid to rats with streptozotocin-induced diabetes: effects on oxidative stress, pro-inflammatory cytokines, and apoptosis in the pancreatic β cell. Endocrine. 2013; 44:369–379.


16. Sgarbossa A, Giacomazza D, di Carlo M. Ferulic acid: a hope for Alzheimer's disease therapy from plants. Nutrients. 2015; 7:5764–5782.


17. Ardiansyah , Ohsaki Y, Shirakawa H, Koseki T, Komai M. Novel effects of a single administration of ferulic acid on the regulation of blood pressure and the hepatic lipid metabolic profile in stroke-prone spontaneously hypertensive rats. J Agric Food Chem. 2008; 56:2825–2830.


18. Jung EH, Kim SR, Hwang IK, Ha TY. Hypoglycemic effects of a phenolic acid fraction of rice bran and ferulic acid in C57BL/KsJ-db/db mice. J Agric Food Chem. 2007; 55:9800–9804.


19. Saija A, Tomaino A, Trombetta D, De Pasquale A, Uccella N, Barbuzzi T, et al. In vitro and in vivo evaluation of caffeic and ferulic acids as topical photoprotective agents. Int J Pharm. 2000; 199:39–47.


20. Lee MJ, Cha HJ, Lim KM, Lee OK, Bae S, Kim CH, et al. Analysis of the microRNA expression profile of normal human dermal papilla cells treated with 5α-dihydrotestosterone. Mol Med Rep. 2015; 12:1205–1212.


21. Livak KJ, Schmittgen TD. Analysis of relative gene expression data using real-time quantitative PCR and the 2(-Delta Delta C(T)) Method. Methods. 2001; 25:402–408.


22. Hildesheim J, Bulavin DV, Anver MR, Alvord WG, Hollander MC, Vardanian L, et al. Gadd45a protects against UV irradiation-induced skin tumors, and promotes apoptosis and stress signaling via MAPK and p53. Cancer Res. 2002; 62:7305–7315.
23. Fornace AJ Jr, Nebert DW, Hollander MC, Luethy JD, Papathanasiou M, Fargnoli J, et al. Mammalian genes coordinately regulated by growth arrest signals and DNA-damaging agents. Mol Cell Biol. 1989; 9:4196–4203.


24. Zhou BR, Xu Y, Wu D, Permatasari F, Gao YY, Luo D. Ginsenoside Rg1 protects human fibroblasts against psoralen- and UVA-induced premature senescence through a telomeric mechanism. Arch Dermatol Res. 2012; 304:223–228.


25. Chen A, Huang X, Xue Z, Cao D, Huang K, Chen J, et al. The role of p21 in apoptosis, proliferation, cell cycle arrest, and antioxidant activity in UVB-irradiated human HaCaT keratinocytes. Med Sci Monit Basic Res. 2015; 21:86–95.


26. Kearsey JM, Coates PJ, Prescott AR, Warbrick E, Hall PA. Gadd45 is a nuclear cell cycle regulated protein which interacts with p21Cip1. Oncogene. 1995; 11:1675–1683.
27. Park JM, Choi JY, Yi JM, Chung JW, Leem SH, Koh SS, et al. NDR1 modulates the UV-induced DNA-damage checkpoint and nucleotide excision repair. Biochem Biophys Res Commun. 2015; 461:543–548.


28. Lo HL, Nakajima S, Ma L, Walter B, Yasui A, Ethell DW, et al. Differential biologic effects of CPD and 6-4PP UV-induced DNA damage on the induction of apoptosis and cell-cycle arrest. BMC Cancer. 2005; 5:135.


29. Chiganças V, Batista LF, Brumatti G, Amarante-Mendes GP, Yasui A, Menck CF. Photorepair of RNA polymerase arrest and apoptosis after ultraviolet irradiation in normal and XPB deficient rodent cells. Cell Death Differ. 2002; 9:1099–1107.


30. Chiganças V, Miyaji EN, Muotri AR, de Fátima, Amarante-Mendes GP, Yasui A, et al. Photorepair prevents ultraviolet-induced apoptosis in human cells expressing the marsupial photolyase gene. Cancer Res. 2000; 60:2458–2463.
31. You YH, Lee DH, Yoon JH, Nakajima S, Yasui A, Pfeifer GP. Cyclobutane pyrimidine dimers are responsible for the vast majority of mutations induced by UVB irradiation in mammalian cells. J Biol Chem. 2001; 276:44688–44694.


32. Jaszewska E, Soin M, Filipek A, Naruszewicz M. UVA-induced ROS generation inhibition by Oenothera paradoxa defatted seeds extract and subsequent cell death in human dermal fibroblasts. J Photochem Photobiol B. 2013; 126:42–46.


33. Aroun A, Zhong JL, Tyrrell RM, Pourzand C. Iron, oxidative stress and the example of solar ultraviolet A radiation. Photochem Photobiol Sci. 2012; 11:118–134.


34. Maverakis E, Miyamura Y, Bowen MP, Correa G, Ono Y, Goodarzi H. Light, including ultraviolet. J Autoimmun. 2010; 34:J247–J257.


35. Saify K, Saadat I, Saadat M. Down-regulation of antioxidant genes in human SH-SY5Y cells after treatment with morphine. Life Sci. 2016; 144:26–29.


36. Kohl E, Steinbauer J, Landthaler M, Szeimies RM. Skin ageing. J Eur Acad Dermatol Venereol. 2011; 25:873–884.


37. Krutmann J. The role of UVA rays in skin aging. Eur J Dermatol. 2001; 11:170–171.
38. Lee KS, Cha HJ, Lee GT, Lee KK, Hong JT, Ahn KJ, et al. Troxerutin induces protective effects against ultraviolet B radiation through the alteration of microRNA expression in human HaCaT keratinocyte cells. Int J Mol Med. 2014; 33:934–942.


39. Assefa Z, Van Laethem A, Garmyn M, Agostinis P. Ultraviolet radiation-induced apoptosis in keratinocytes: on the role of cytosolic factors. Biochim Biophys Acta. 2005; 1755:90–106.


40. Rayess H, Wang MB, Srivatsan ES. Cellular senescence and tumor suppressor gene p16. Int J Cancer. 2012; 130:1715–1725.


41. Mancuso C, Santangelo R. Ferulic acid: pharmacological and toxicological aspects. Food Chem Toxicol. 2014; 65:185–195.


42. Quan T, Qin Z, Xu Y, He T, Kang S, Voorhees JJ, et al. Ultraviolet irradiation induces CYR61/CCN1, a mediator of collagen homeostasis, through activation of transcription factor AP-1 in human skin fibroblasts. J Invest Dermatol. 2010; 130:1697–1706.


43. Yang Y, Li S. Dandelion extracts protect human skin fibroblasts from UVB damage and cellular senescence. Oxid Med Cell Longev. 2015; 2015:619560.


44. Cho S, Won CH, Lee DH, Lee MJ, Lee S, So SH, et al. Red ginseng root extract mixed with Torilus fructus and Corni fructus improves facial wrinkles and increases type I procollagen synthesis in human skin: a randomized, double-blind, placebo-controlled study. J Med Food. 2009; 12:1252–1259.


45. Kim JA, Ahn BN, Kong CS, Kim SK. The chromene sargachromanol E inhibits ultraviolet A-induced ageing of skin in human dermal fibroblasts. Br J Dermatol. 2013; 168:968–976.

