Abstract
Methods
Rats were immobilized for 24 hours and blood samples, and skin biopsies were taken. The effect of stress-serum on the in vitro proliferation of rat and human dermal papilla cells (hDPCs), as well as serum cortisol and corticotropin-releasing hormone levels, were measured. Mast cell staining was performed on the biopsied tissue. In addition, Western blot and quantitative real time polymerase chain reaction were used to assess mast cell tryptase and cytokine expression, respectively in rat skin biopsies.
Results
Stress-serum treatment reduced significantly the number of viable hDPCs and arrested the cell cycle in the G1 phase, compared to serum from unrestrained rats (p<0.05, respectively). Moreover, restrained rats had significantly higher levels of cortisol in serum than unrestrained rats (p<0.01). Acute stress serum increased mast cell numbers and mast cell tryptase expression, as well as inducing interleukin (IL)-6 and IL-1β up-regulation.
Stress has long been suspected as one of the causes of hair loss but sometimes the evidence supporting an association has been weak1. However, stress has been reported to play an important role in the pathophysiology of alopecia2345. Arck et al.6 reported for the first time that stress leads to the premature onset of catagen in mice, an effect which appears to be the result of substance P (SP) stimulation of mast cell degranulation in the context of the brain-hair follicle axis. Mast cells are regarded as the main cell type involved in stress-induced neurogenic inflammation78.
In addition to the SP-mast cell pathway, stress is known to activate the hypothalamic pituitary adrenal (HPA) axis, being the hair follicle one of the various targets of HPA axis activity910. Mouse bulge stem cells express glucocorticoid receptors, and are sensitive to the anti-proliferative effect of glucocorticoids which induce premature onset of catagen and hair growth inhibition11. We previously reported that glucocorticoids inhibit human hair growth by inhibiting both dermal papilla cells (DPCs) proliferation and growth factor expression on these cells12. In this study, we investigated the effect of acute stress on hair growth in vitro on rat and human cells, and in vivo using rat skin biopsies in the context of both, the SP-mast cell pathway and HPA axis activity.
All experiments involving rats were performed in accordance with the regulations and the approval of the Institutional Animal Care and Use Committee (IACUC) of the Seoul National University Hospital (IRB no. H-1102-055-351, IACUC no. 15-0023). Twenty male Sprague-Dawley rats were assigned to the experimental or control groups (ten animals per group). In the experimental group, immobilization was exerted for 24 hours using an individual rodent restrainer device (Model 544-RR; Plas Labs Inc., Lansing, MI, USA) (Fig. 1)13, which allowed rodent immobilization in a non-traumatic manner. Animals from the control group, receiving no stress, were isolated for the same time, but not immobilized. At the end of the isolation or immobilization, all animals were anaesthetized with sodium pentobarbital (50 mg/kg, intraperitoneal injection), and 4~5 ml of blood were immediately collected via intracardiac puncture, followed by cardiac perfusion with a saline solution (NaCl 0.9%, 200~250 ml per rat). Blood samples were centrifuged at 3,800g for 10 minutes, and the serum obtained was stored at −70℃.
The methods used for isolating and culturing human DPCs (hDPCs) have been described previously14. Dr. Wendy Filsell (Unilever Research, Sharnbrook, UK) kindly provided the rat vibrissae DPC lines. Cell line culture and DPC proliferation assays were performed as described previously1516. Cell viability was determined using an MTT [3-(4,5-dimethylthiazol-2-yl)-2,5-diphenyltetrazolium bromide] assay. All samples were run in triplicate in each independent experiment.
After a 72-hour serum treatment, hDPCs were fixed with 70% ethanol and incubated for 1 hour at 4℃. Cells were stained with propidium iodide (50 mg/ml) and supplemented with RNase A (Sigma-Aldrich, Steinheim, Germany) for 30 min at 4℃ prior to flow cytometry analysis. All samples were independently analyzed and repeated three times.
Cortisol levels and corticotropin-releasing hormone (CRH) in serum samples were measured using the commercial Cortisol EIA kit (Cayman Chemical Company, Ann Arbor, MI, USA), and the CRH Enzyme-linked Immunosorbent Assay Kit (USCN Life Science Inc., Wuhan, China), respectively, following the manufacturer's protocols.
Skin sections were deparaffinized and incubated in a 1% Alcian blue solution for 30 min. After rinsing, the sections were counterstained in nuclear fast red solution for 3 min, followed by washing. The sections were then dehydrated with 95% absolute alcohol and cleared with a xylene substitute prior to mounting. Alcian blue-positive mast cells were counted in different fields at ×100 magnification and the mean cell number per skin section was calculated.
For immunofluorescence staining, 4 µm sections were obtained from frozen skin sections and fixed with acetone at −20℃ for 15 min. Sections were blocked with blocking solution for 30 min (Golden Bridge International Labs, Mukilteo, WA, USA) and incubated overnight with an anti-mast cell tryptase antibody (Epitomics, Burlingame, CA, USA) in a 4℃ humidified chamber. The sections were then incubated with an Alexa Fluor 488-labeled goat anti-rabbit secondary antibody (Invitrogen, Tokyo, Japan), for 1 hour at room temperature. The slides were analyzed using a confocal laser-scanning microscope (Carl Zeiss MicroImaging, Inc., Thornwood, NY, USA).
Skin samples were immediately placed into liquid nitrogen and stored at −70℃ until they were required for experiments. Quantitative real time polymerase chain reaction (qPCR) was performed as described previously using GAPDH, interleukin (IL)-1β, IL-6, IL-13, tumor necrosis factor (TNF)-α primers for rat genes17. Western blot analysis was performed as described previously using anti-β-actin, anti-mast cell tryptase monoclonal antibodies18. Samples from three independent experiments run in triplicate were analyzed.
The Mann-Whitney test was used for the hormone level assay, mast cell counts, and qPCR. The Wilcoxon Signed Rank test was used for MTT assay and flow cytometry. Results are presented as means±standard error in graphs. All quoted p-values are two-tailed, and significance was accepted at values <0.05. Data were analyzed with GraphPad Prism 6 (GraphPad Software, Inc., San Diego, CA, USA).
The viability of rat DPCs treated with 25% serum from stressed rats (stress serum) was lower than that of DPCs treated with 25% serum from control rats (control serum) after a 24, 48, 72-hour incubation. This effect was more apparent at 72 hours when the number of viable rat DPCs decreased significantly in stress serum-treated cells compared with those in control serum-treated cells (p<0.05, Fig. 2A). The stress serum had similar effects on hDPCs (Fig. 2B). After a 48 and 72-hour incubation, the number of viable hDPCs was significantly lesser in stress serum-treated than in control serum-treated cells (p<0.05, respectively).
The stress induced by the 24-hour restraint increased significantly the number of mast cells in the rat perifollicular tissue. The average number of mast cells in a ×100 magnification field was significantly higher in the stress group than in the control group (n=5 per group, p<0.05, Fig. 3A, B). An increase in the skin tryptase levels in the stress group was revealed by Western blot analysis (n=3 per group, Fig. 3C). Mast cell tryptase expression was higher in the stress group than in the control group, as shown by tryptase immunofluorescence staining (n=3 of each group, Fig. 3D). Tryptase mean fluorescence intensity was 205.3%±50.9% in the stress group compared with that in the control group.
qPCR showed that IL-6 and IL-1β mRNA expression levels were significantly higher in the stress group than in the control group (p<0.05, respectively). Although IL-13 and TNF-α expression levels were also higher, these increases were not statistically significant (Fig. 4).
Liu et al.19 reported that chronic stress caused by restraint inhibits hair growth via SP in mice. However, whether acute stress causes a similar inhibitory effect on hair growth remains uncertain. Based on results from our previous study showing that dexamethasone inhibits human hair growth, we hypothesized whether increased cortisol levels found in stress serum would also inhibit hair growth12. Our results confirmed that cortisol levels increased approximately two-fold after a 24-hour restraint compared with unrestrained rats. As CRH levels remained unchanged, we speculate that increased cortisol levels exerted a negative feedback on the HPA axis, leading to CRH suppression20.
The important role of mast cells in stress-induced hair loss is well known. Our results confirmed that mast cells were activated after acute stress, as demonstrated previously8. In addition, the significant increase in the total number of perifollicular mast cells we observed, may be the result of increased recruitment of mast cell progenitors in the circulation caused by restraint-induced acute stress. Mast cells originating from hematopoietic stem cells, can infiltrate the inflamed tissue within 24 hours2122. As glucocorticoid suppresses mast cell activation, such an increase in mast cell activation and numbers appears paradoxical in view of the increased cortisol levels that we found. In fact, glucocorticoids are widely used to treat allergic diseases in which mast cells are involved23. However, a suggested explanation is that acute stress may induce CRH secretion, and CRH is known to induce mast cell degranulation and increase mast cells numbers24. Although our results did not show an increase in CRH levels after a 24-hour restraint, it is possible that their levels raised earlier, triggering the cortisol negative feedback on the HPA axis. An alternative explanation involves the SP-mast cell pathway. As SP action upon mast cells is opposed to that of glucocorticoids, an increased in mast cell activation and infiltration might suggest that SP exerts a more powerful effect on mast cells than glucocorticoids, as a result of acute stress events.
SP induces the release of mast cell cytokines with inflammatory, anti-proliferative, and pro-apoptotic function which have an effect on the hair follicle epithelium25. We observed a significantly increased expression of IL-6 and IL-1β mRNA in the skin of the stressed rats. IL-6 is an important proinflammatory mediator produced by mast cells, which inhibits hair shaft elongation and suppresses proliferation of matrix cells in cultured human hair follicles2627. IL-1β is also known to inhibit hair follicle growth, and is highy expressed in patients with active alopecia areata28. Interestingly, both inflammatory cytokines, IL-6 and IL-1β are suppressed by cortisol29. However, in our study, cortisol did not fully suppress both cytokines after rats were exposed to acute stress.
Stress responses stimulate the SP-mast cell pathway as well as cortisol production via the HPA axis. The SP-mast cell pathway leads to various inflammatory reactions, and the cortisol, produced as a result of HPA axis activity, modulates such inflammation. Although the body's homeostatic mechanisms play an essential role in balancing the effects of different pathways, the SP-mast cell and HPA axis appear to exert similar effects on hair growth inhibition. Furthermore, the SP-mast cell pathway is known to play a role in the inhibition of hair follicle epithelial proliferation, and we found that serum, which contains increased cortisol after acute stress, inhibited mesenchyma-derived DPCs proliferation. These results suggest that in addition to the SP-mast cell pathway, acute stress can affect hair growth via elevated serum cortisol levels. This study has limitation that did not investigate other stress related factors besides cortisol in the serum after acute stress. Therefore it can be questioned whether cortisol was the most critical component in the serum to inhibit DPCs proliferation. Further studies to clarify more the link between acute stress and alopecia are needed. In conclusion, as well as conventional medical treatments, physicians should consider stress-reducing strategies as part of a comprehensive approach when treating patients with alopecia.
Figures and Tables
Fig. 2
(A) Effect of stress serum on cell viability of rat dermal papilla cells (DPCs), and (B) human DPCs (hDPCs). (C) Percentage of hDPCs in G1 phase, and (D) in S phase after the treatment with stress serum. (E) Cortisol levels, and (F) corticotropin-releasing hormone (CRH) levels of stress serum. *p<0.05, and **p<0.01, compared with the control serum.
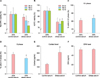
Fig. 3
(A) Mast cells show a purple color after Alcian blue staining in rat perifollicular tissue (×400). (B) The mean number of mast cells at ×100 magnification. *p<0.05. (C) Relative protein levels were compared by Western blot analysis. (D) Restraint-induced stress increased the expression of mast cell tryptase in the stress group compared with that of the control group.
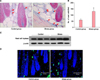
ACKNOWLEDGMENT
We thank Wendy Filsell (Unilever Research, Colworth House, Sharnbrook, Bedford MK44 1LQ, UK) for the kind gift of the vibrissa dermal papilla rat cell line (DPLTtsa 6). This research was supported by a grant of the Korea Health Technology R&D Project through the Korea Health Industry Development Institute (KHIDI), funded by the Ministry of Health & Welfare, Republic of Korea (grant number: HI13C1853) and a research agreement with AmorePacific Corporation, Republic of Korea.
References
1. Hadshiew IM, Foitzik K, Arck PC, Paus R. Burden of hair loss: stress and the underestimated psychosocial impact of telogen effluvium and androgenetic alopecia. J Invest Dermatol. 2004; 123:455–457.


2. Manolache L, Petrescu-Seceleanu D, Benea V. Alopecia areata and relationship with stressful events in children. J Eur Acad Dermatol Venereol. 2009; 23:107–109.


3. Chu SY, Chen YJ, Tseng WC, Lin MW, Chen TJ, Hwang CY, et al. Psychiatric comorbidities in patients with alopecia areata in Taiwan: a case-control study. Br J Dermatol. 2012; 166:525–531.


4. Manolache L, Benea V. Stress in patients with alopecia areata and vitiligo. J Eur Acad Dermatol Venereol. 2007; 21:921–928.


5. Atefi N, Soltani-Arabshahi R, Afkham-Ebrahimi A. Stressful life events and diffuse unexplained hair loss in women: a case-control study. Dermatology. 2006; 213:44–45.


6. Arck PC, Handjiski B, Hagen E, Joachim R, Klapp BF, Paus R. Indications for a 'brain-hair follicle axis (BHA)': inhibition of keratinocyte proliferation and up-regulation of keratinocyte apoptosis in telogen hair follicles by stress and substance P. FASEB J. 2001; 15:2536–2538.


7. Arck PC, Slominski A, Theoharides TC, Peters EM, Paus R. Neuroimmunology of stress: skin takes center stage. J Invest Dermatol. 2006; 126:1697–1704.


8. Arck PC, Handjiski B, Kuhlmei A, Peters EM, Knackstedt M, Peter A, et al. Mast cell deficient and neurokinin-1 receptor knockout mice are protected from stress-induced hair growth inhibition. J Mol Med (Berl). 2005; 83:386–396.


9. Theoharides TC, Spanos C, Pang X, Alferes L, Ligris K, Letourneau R, et al. Stress-induced intracranial mast cell degranulation: a corticotropin-releasing hormone-mediated effect. Endocrinology. 1995; 136:5745–5750.


10. Esposito P, Chandler N, Kandere K, Basu S, Jacobson S, Connolly R, et al. Corticotropin-releasing hormone and brain mast cells regulate blood-brain-barrier permeability induced by acute stress. J Pharmacol Exp Ther. 2002; 303:1061–1066.


11. Chebotaev DV, Yemelyanov AY, Lavker RM, Budunova IV. Epithelial cells in the hair follicle bulge do not contribute to epidermal regeneration after glucocorticoid-induced cutaneous atrophy. J Invest Dermatol. 2007; 127:2749–2758.


12. Choi SJ, Cho AR, Jo SJ, Hwang ST, Kim KH, Kwon OS. Effects of glucocorticoid on human dermal papilla cells in vitro. J Steroid Biochem Mol Biol. 2013; 135:24–29.


13. Méndez-Cuesta LA, Márquez-Valadez B, Pérez-De La Cruz V, Escobar-Briones C, Galván-Arzate S, Alvarez-Ruiz Y, et al. Diazepam blocks striatal lipid peroxidation and improves stereotyped activity in a rat model of acute stress. Basic Clin Pharmacol Toxicol. 2011; 109:350–356.


14. Shin H, Cho AR, Kim DY, Munkhbayer S, Choi SJ, Jang S, et al. Enhancement of human hair Growth using ecklonia cava polyphenols. Ann Dermatol. 2016; 28:15–21.


15. Shin H, Yoo HG, Inui S, Itami S, Kim IG, Cho AR, et al. Induction of transforming growth factor-beta 1 by androgen is mediated by reactive oxygen species in hair follicle dermal papilla cells. BMB Rep. 2013; 46:460–464.


16. Yoon SY, Yoon JS, Jo SJ, Shin CY, Shin JY, Kim JI, et al. A role of placental growth factor in hair growth. J Dermatol Sci. 2014; 74:125–134.


17. Jo SJ, Choi SJ, Yoon SY, Lee JY, Park WS, Park PJ, et al. Valproic acid promotes human hair growth in in vitro culture model. J Dermatol Sci. 2013; 72:16–24.


18. Kwon OS, Pyo HK, Oh YJ, Han JH, Lee SR, Chung JH, et al. Promotive effect of minoxidil combined with all-trans retinoic acid (tretinoin) on human hair growth in vitro. J Korean Med Sci. 2007; 22:283–289.


19. Liu N, Wang LH, Guo LL, Wang GQ, Zhou XP, Jiang Y, et al. Chronic restraint stress inhibits hair growth via substance P mediated by reactive oxygen species in mice. PLoS One. 2013; 8:e61574.


20. Ghisleni G, Capiotti KM, Da Silva RS, Oses JP, Piato ÂL, Soares V, et al. The role of CRH in behavioral responses to acute restraint stress in zebrafish. Prog Neuropsychopharmacol Biol Psychiatry. 2012; 36:176–182.


22. Prignano F, Lotti T, Spallanzani A, Berti S, de Giorgi V, Moretti S. Sequential effects of photodynamic treatment of basal cell carcinoma. J Cutan Pathol. 2009; 36:409–416.


23. Yoshikawa H, Tasaka K. Suppression of mast cell activation by glucocorticoid. Arch Immunol Ther Exp (Warsz). 2000; 48:487–495.


24. Ito N, Sugawara K, Bodó E, Takigawa M, van Beek N, Ito T, et al. Corticotropin-releasing hormone stimulates the in situ generation of mast cells from precursors in the human hair follicle mesenchyme. J Invest Dermatol. 2010; 130:995–1004.


25. Peters EM, Arck PC, Paus R. Hair growth inhibition by psychoemotional stress: a mouse model for neural mechanisms in hair growth control. Exp Dermatol. 2006; 15:1–13.


26. Ilves T, Harvima IT. Decrease in chymase activity is associated with increase in IL-6 expression in mast cells in atopic dermatitis. Acta Derm Venereol. 2015; 95:411–416.


27. Kwack MH, Ahn JS, Kim MK, Kim JC, Sung YK. Dihydrotestosterone-inducible IL-6 inhibits elongation of human hair shafts by suppressing matrix cell proliferation and promotes regression of hair follicles in mice. J Invest Dermatol. 2012; 132:43–49.

