Abstract
Background
Kojic acid was used for decades in the cosmetic industry as an antimelanogenic agent. However, there are two major drawbacks of Kojic acid, one is cytotoxicity and second are instability on storage. These limitations led the scientist to synthesize the active Kojic acid peptides.
Objective
In the present study, we synthesize and investigate the effect of five Kojic acid peptides to overcome the limitation of Kojic acid.
Methods
The peptide was analyzed and purified by high-performance liquid chromatography and matrix-assisted laser desorption ionization time of flight mass spectroscopy. Further, the tyrosinase activities of the Kojic acid and Kojic acid peptides were compared. The toxicity was measured and the melanin content is recorded in B16F10 mouse melanoma cells.
Results
Maximum tyrosinase activity was measured by Kojic acid peptides. Therefore, Kojic acid peptides were subjected to melanin assay and cytotoxicity assay and finally the stability of the Kojic acid peptide was measured.
Conclusion
It was observed that this newly synthesized Kojic acid peptide is stable and potent to inhibit the tyrosinase activity and melanin content of B16F10 mouse melanoma cells without exhibiting cell toxicity. Together, these preliminary results suggest that a further exploration is being needed to establish Kojic acid peptide as antimelanogenic agent.
The consumption of skin care products and skin whitening agents generates billions of dollars. The world market for skin whitening is still developing and targeting all over the world1. To fulfill the demand of the cosmetic market, naturally occurring biologically active ingredient was exploited for decades in the cosmetic industry. However, from the heap of naturally stirring biologically active constituents, hunt for active ingredients in the natural skin whitening agent is difficult.
The knowledge of analytical sciences, biochemistry, and other disciplines has influenced the identification of new cosmetic ingredients. Recent approaches of skin whitening have provided the opportunity to choose more effective cosmetic ingredients. Conventionally, plant extract, hydrolytes, essential oil, and vitamins were used as a skin whitening agent. However, because of limitations in search of clinically proven safe and effective cosmetic formulation, now synthetic molecules are being designed tested and prefer over the traditional ones. In recent times, peptide emerges as a more effective cosmetic agent that contains a large number of individual molecules2.
Melanin is the pigment which provides the color to skin ranges widely from black to fair. Its prime function is to protect the skin from ultraviolet exposure. The step of melanin synthesis in melanocytes is mediated by several enzymes, of which tyrosinase is essential. Tyrosinase (EC 1.14.18.1) 2~7 (monophenol or o-diphenol oxygen oxidoreductase), also known as monophenol oxidase, monophenol monooxygenase, N-acetyl-6-hydroxytryptophan oxidase, phenolase, tyrosine-dopa oxidase and cresolase. It is a copper-containing enzyme, that is widespread in nature and the most exploited enzymes solely due to the key role in mammalian melanogenesis. The entire process of melanin synthesis requires a combination of enzymatically catalyzed and chemical reactions for the formation of dark macromolecule i.e., melanin. There is a skin whitening agent available in the cosmetic industry as a skin whitening agent which focus on modulation of the tyrosinase enzyme in melanin synthesis pathway3. These skins whitening agents like azelaic acid45, magnesium-L-ascorbyl-2-phosphate, electron-rich phenols6, hydroxyanisole, corticosteroids7, N-acetyl-4-S-cysteaminylphenol, resinoids89, arbutin (hydroquinone-beta-D-glucopyranoside), salicylhydroxamic acid, dioic acid, Kojic acid (KA), and hydroquinone are the most widely used in the cosmetic industry. They are recommended worldwide, but are associated with potential mutagenic and adverse effects including skin irritation, contact dermatitis, low stability toward oxygen, high toxicity and insufficient skin penetration ability and water, and exogenous ochronosis10. Because of side effect of hydroquinone, it is under strict control of the Food and Drug Administration and prohibited in European Union10. Despite of all the efforts made so far, development of high-performance tyrosinase inhibitors is much needed and the opportunity to find the effective tyrosinase inhibitor molecule is open.
KA [5-hydroxy-2-(hydroxymethy1)-y-pyrone], a fungal metabolite that has been used in many countries as skin whitening agent11. It worked through inhibition of tyrosinase activity and thereby reducing melanin contents11. Mixed inhibitory effect on the diphenolase activity and competitive inhibitory effect on monophenolase activity were observed by KA. However, due to limitation of the cytotoxicity and instability on storage, its use in cosmetics has been limited1213. Consequently, the researchers synthesized KA derivatives to enhance the properties by modifying the C-7 hydroxyl group141516.
The objective of this study is to synthesize KA-peptide using solid-phase parallel peptide synthesis using Fmoc-Rink amide aminomethyl-polystyrene resin. KA was activated by carbonyldiimidazole (CDI) and coupled to the N-terminal of the tripeptides on the resin. After removing the resin, KA-peptides were obtained with yield and purity. The tyrosinase activity was measured with compared to KA-PS and free KA. Further, cytotoxicity and melanin inhibition was performed to know the effects of the KA-PS on melanin synthesis and cell viability.
In this study, analytical grade chemicals and reagent grade solvents were used without further purification. Dulbecco's modified eagle's medium (DMEM; Gibco BRL, Eggenstein, Germany), dimethyl sulfoxide (DMSO), 3-(4,5-dimethylthiazol-2-yl)-2,5-diphenyltetrazolium bromide (MTT), 12-O-tetradecanoylphorbol- 13-acetate (TPA), were procured from Sigma Chemical Co. (St. Louis, MO, USA). Trypsin-ethylenediaminetetraacetic acid (EDTA) 0.5% solution (10×), phosphate buffered saline (PBS, pH 7.4) and, penicillin/ streptomycin (10,000 U/ml) were purchased from Invitrogen Corp. (Carlsbad, CA, USA). Fmoc-l-A.A-OH (Bead-Tech, Seoul, Korea) and Fmoc-A.A-Wang resin (Merck, Frankfurt, Darmstadt, Germany) were used. Hydroxybenzotriazole (HOBt) and diisoproylcarbodiimidazole (DIC) were obtained from GLS (Shanghai, China). KA was purchased from Wako (Tokyo, Japan) and 1,1-carbonyldiimidazole (CDI) was purchased from Acros (Belgium, NJ, USA). Diisopropylethylamine, N,N'-dimethylformamide (DMF), dichloromethane (DCM), piperidine and diethyl ether were purchased from Daejung (Siheung, Korea). 1,2-ethanedithiol (EDT), thioanisole was procured from Alfa Aesar (Lancaster, UK).
KA (5-hydroxy-2-(hydroxymethyl)-4#-pyran-4-one, 142.1 g, 1 mol) was dissolved in a solution of tetrahydrofurane (THF) and DMF in a proportion of 600 ml and 50 ml respectively and kept for stirring for 1 hour, at room temperature. CDI (146.0 g, 0.9 equivalent [eq.]) was dissolved in THF (200 ml) and added to the solution. The resulting yellow solid (3) were filtered, washed with THF or ether and dried in vacuum to give yellow solids (Fig. 1).
KA conjugated peptides were synthesized by the solid-phase method using Fmoc chemistry. The C-terminal amino acid residue pre-loaded wang resins swelled in DCM for 1 hour. Peptide chains were elongated in the consecutive cycles of deprotection and coupling. Deprotection was performed with 20% piperidine in the DMF for 20 minutes and 15 min respectively. HOBt/DIC chemistry; was used for chain elongation from 5 equivalents of protected amino acid derivatives. Subsequently, Fmoc group of the final amino acid residue was removed and compound 3 (3 eq.) and HOBt (3 eq.) were added to the resin-bound peptides (Fig. 2). The mixture was incubated for 2 hours and the resin was treated with a cleavage solution (reagent K; trifluoroacetic acid (TFA)/thioanisole/phenol/water/EDT in the ration of 82.5/5/5/5/2.5 (v/v)) for 2 hours at room temperature. Later on, the resin was filtered. Crude peptides in the filtrate were concentrated under high vacuum, and precipitated with pre-cold ether to yield KA conjugated peptides. The crude peptide was analyzed and purified by high-performance liquid chromatography (HPLC) on a Waters 600E system (Waters, Milford, MA, USA), using Phenomenex, C18, 5 µm column (300×22 mm). The solvent system which comprises of 0.1% TFA in H2O (solvent A) and 0.1% TFA in acetonitrile (solvent B) was used in a linear gradient from 4% to 14% B for 15 min with a flow rate 5 ml/min. The elution was monitored at 230 nm. The purity of the synthesized peptide was checked on another Shim-pack column, C18, 5 µm column (250×4.6 mm). The solvent system 0.1% TFA in H2O (solvent A), 0.1% TFA in acetonitrile (solvent B) was used in a linear gradient from 5% to 65% B in 30 minutes while monitoring at 230 nm. The peptide was further characterized by matrix-assisted laser desorption ionization time of flight mass spectroscopy (MALDI-TOF-MS) (Voyager-DE STR; Applied Biosystems, Foster city, CA, USA), using α-cyano-4-hydroxycinnamic acid as a matrix.
Highly pigmented B16F10 mouse melanoma cell and Melan-a cell line, a highly pigmented, immortal, non-tumorigenic mouse melanocyte cell line was used for the present study. The cells were generously provided by Prof. Dorothy C. Bennett (St. George's Hospital Medical School, London, UK). Culture procedures were carried out as described previously17. In brief, B16F10 murine melanoma cells were cultured in DMEM supplemented with 10% (V/V) fetal bovine serum, 1% (V/V) penicillin /streptomycin (100 units/ml) at 37℃ in a humidified atmosphere in a 5% CO2. The Melan-a murine melanocytes were cultured in Roswell Park Memorial Institute (RPMI) 1640 Medium supplemented with 10% (v/v) fetal bovine serum, 1% (v/v) penicillin/streptomycin (100 units/ml) and 200 nM TPA at 37℃ in a humidified atmosphere with 10% CO2. The subculture of the cells was carried out at 4th day of incubation by a passage number 20 was achieved.
Mushroom tyrosinase (EC 1.14.18.1) used for the bioassay was purchased from Sigma Chemical Co.. In vitro tyrosinase assay was assessed according to Kubo and Kinst-Hori18 with little modification. Briefly, In a 96 well plate system, mushroom tyrosinase was performed in triplicates through a mixture preparation. The mixture was prepared by adding 40 µl of phosphate buffer, 100 µl of the sample (KA-PS) and the positive control (KA). Further 20 µl of tyrosinase enzyme (1,100 units/ml:0.516 mg/ml phosphate buffer 0.1 M), L-dopa (5 mM) was added in the 40 µl volume and the mixture was incubated for ten minutes at 37℃. The optical density of dopachrome formation was measured using the spectrophotometric enzyme-linked immunosorbent assay reader at an optical density of 475 nm (The inhibitory percentage of tyrosinase was calculated as follows: Inhibition=[(ΔAcontrol-ΔAsample)/ΔAcontrol]×100). The IC50, defined as the concentration of KA-peptides required to inhibit tyrosinase activity by 50%, was determined for each sample. All experiments were performed at least three times, with similar consequences.
The viability of cells treated with respective KA-PS compounds was determined using an MTT assay. Briefly, 3×104 B16F10 cells were seeded and adhered in 96-well plates. After 24 hours, DMEM was removed and 200 µl of various concentrations of fresh DMEM and different KA-PS solution were added, and the cells were incubated for 48 hours at 37℃ in 5% CO2 incubator. After incubation, the medium was removed, and 100 µl of MTT in PBS solution (0.5 mg/ml) was added and incubated for 2 hours. Then blue crystalline precipitates were dissolved in 200 µl of 100% DMSO and viability was assessed by measuring absorbance at 540 nm using a molecular device "Spectra Max 340 microplate reader"19. Untreated normal cells were used as a control group.
Highly pigmented B16F10 mouse melanoma cells were seeded into the 12-well plate, incubated for 24 hours, and then triplicate cultures were fed with fresh media and incubated for a further 48 hours. Then cells were detached by trypsin/EDTA solution and harvested. The harvested cells were washed twice with PBS for 5 minutes at 5,000 rpm, suspended in 100 µl of extraction buffer (1 N NaOH, 10% DMSO), heated at 80℃ for 1 hour, and transferred to 96-well plate. Melanin content was determined by absorbance at 405 nm in ELISA microplate reader1920. Normal untreated cells were used as a control.
All experiments were performed at least in triplicates and expressed as the means±standard deviation, and were analyzed using the unpaired t-test. A p-value of less than 0.05 was considered to be statistically significant compared with the control. SigmaPlot (Systat Software Inc., San Jose, CA, USA) was used to analyze the data.
By using the fomc chemistry and with the help of activated KA, KA-peptides were synthesized and the yield and purity of KA-peptides were KA-peptide (95.2%, 27.5%), KA-ECG (98.5%, 36.1%), KA-KECG (99.3%, 42.0%), KA-PKEK (99.5%, 32.9%) and KA-CDPGYIGSR (KA-CR9) (98.0%, 22.4%) respectively shown in Table 1.
Mushroom tyrosinase has been used for prescreening hypopigmentation agents because it is commercially available. The mushroom tyrosinase assay was performed in order to know the inhibitory activity of KA-peptides on tyrosinase and were observed that KA-CR-9 and KA-PS inhibit 81% and 82% tyrosinase activity respectively at the concentration of 40 µM (Fig. 3). Fig. 4, 5 show the dose-dependent inhibition of mushroom tyrosinase activity at different concentration (5, 10, 20, 40, and 80 µM) of KA-CR-9 and KA-PS respectively.
Fig. 6A shows the inhibition of melanin by KA-PS in B16F10 mouse melanoma cell line and inhibition of melanin content was observed in α-melanocyte-stimulating hormone (αMSH) induced Melan-a mouse cell (Fig. 6B). At the concentration of 5 mM, the melanin content was inhibited by 42% in the B16F10 melanoma cell. Dose-dependent down-regulation was observed in Melan-a mouse cell.
KA was activated by CDI for coupling of KA to the N-terminal of the peptides via urethane bond yielding KA-imidazole 3 (Fig. 1). The coupling, decoupling reaction was carried out at room temperature to restrict the decomposition of the desired product or from the oxidation of the product. The minimum quantity of DMF was added to the reaction as a cosolvent to improve the solubility of the KA in THF. With the subsequent reaction, the desired product precipitates and finally the yield was 77% (Fig. 1). Using solid-phase Fmoc chemistry, the resin bound peptides were synthesized separately (Fig. 2). The reaction of coupling between activated KA and the resin was time-consuming. Thus, to reduce the time and increase the purity of the final product the general catalyst HOBt was used (Fig. 2). HOBt has proven to be the most effective catalyst for the coupling of the KA-imidazole to the resin-bound tripeptide2. HOBt donates the proton to the leaving group, imidazole and thus increasing the rate of reaction2.
Using solid phase parallel peptide synthesis method, we synthesized five compounds and all the compounds were purified by pre-cold ether precipitation under the high vacuum condition. The compounds synthesized were mentioned in Table 1. Purity and the correctness of the molecular weight value of peptide were analyzed by HPLC and MALDI-TOF-MS. Further, invitro tyrosinase assay was performed to know the effect on tyrosinase. It was observed that the KA-PS and KA-CR9 show the maximum inhibitory effect of tyrosinase activity of 82%. 84%, respectively. The KA-PS, being the low molecular molecule, it was chosen for the further experiment. Therefore, with the help of HPLC crude KA-PS was purified and the purity was more than 95% (S1).
Subsequently, the KA-PS was subjected to the αMSH (10 nM) induced melanin assay and cell viability assay. The KA-PS inhibits the melanin content of B16F10 cells melan-a cell and no cytotoxicity was observed (Fig. 6). Further, the stability of the KA-PS was analyzed by dissolving KA-PS in DMSO at 37℃ for 48 hours. Later on, melanin assay and cytotoxicity showed that KA-PS retains same activity (Data Not shown). Thus, this newly synthesized synthetic peptide (KA-PS) may be a promising ingredient in the cosmetic industry. It can be exploited as a new potent tyrosinase inhibitor, which reduces the melanin content of B16F10 mouse melanoma cell (Fig. 6A). Dose-dependent down-regulation of melanin content was observed in Melan-a mouse cell (Fig. 6B). KA-PS can be used as a new depigmenting agent in the cosmetic industry. However, before KA-PS is used in the cosmetic industry, it must progress through a series of in vivo trials to determine if it is safe and effective in intact skin system. It must be taken into consideration that sometimes the absorbability of the compound differs between cell culture and natural skin2122.
Figures and Tables
Fig. 1
Synthesis of activated Kojic acid by using 1,1-carbonyldiimidazole (CDI) at room temperature.

Fig. 2
Synthesis of Kojic acid peptides (KA-peptides). Reagents and conditions; a) Treat with 2% 1,8-diazabicyclo[5.4.0]undec-7-ene in N,N'-dimethylformamide (DMF) for 10 min; b) Mixed Fomc-A.A2-OH (5.0 equivalent [eq.]), hydroxybenzotriazole (HOBt) (5.0 eq.), and diisopropylcarbodiimide (5.0 eq.) with resin for 2 hours; c) repeat a) and b) procedure; d) React with KA-imidazole (3.0 eq.), HOBt (3.0 eq.) in DMF for overnight; e) React with reagent K. [trifluoroacetic acid/thioanisole/phenol/water/ethanedithiol (82.5/5/5/5/2.5(v/v)] for 1 hour, and treat with pre-cooled diethylether.
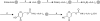
Fig. 3
Screening of newly synthesized Kojic acid peptides (KA-PKEK, KA-ECG, KA-CR9, KA-KECG, and KA-PS) through the invitro tyrosinase assay. Data are mean±standard deviation. **p<0.001; t-test.
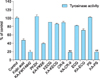
Fig. 4
Showing the dose dependent decrease in the mushroom tyrosinase activity by KA-CR-9. Data are mean±standard deviation. *p<0.05, **p<0.001, ***p<0.0001; t-test.
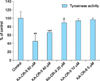
Fig. 5
Showing the dose dependent inhibition of the mushroom tyrosinase activity by KA-PS. Data are mean±standard deviation. *p<0.05, **p<0.001, ***p<0.0001; t-test.
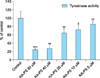
Fig. 6
B16F10 melanoma cell and melan-a cells were cultured in the presence of Roswell Park Memorial Institute media supplemented with 10 fetal bovine serum. Arbutin and KA-PS acid inhibited melanin content of α-melanocyte-stimulating hormone (10 nM) induced B16F10 melanoma cells (A) and melan-a cells (B). Melanin content and cell viability (% of control) in both B16F10 cells (A) and melan-a melanocytes (B) are shown. Data are mean±standard deviation. *p<0.05, **p<0.001; t-test.
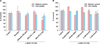
ACKNOWLEDGMENT
This study was supported by a grant of the Korea Industrial Complex Corp. (KICOX, No. 1415136429).
References
1. Jones G. Beauty imagined: a history of the global beauty industry. Oxford and New York: Oxford University Press;2010.
2. Noh JM, Kwak SY, Kim DH, Lee YS. Kojic acid-tripeptide amide as a new tyrosinase inhibitor. Biopolymers. 2007; 88:300–307.


3. Slominski A, Tobin DJ, Shibahara S, Wortsman J. Melanin pigmentation in mammalian skin and its hormonal regulation. Physiol Rev. 2004; 84:1155–1228.


4. Breathnach AC, Nazzaro-Porro M, Passi S, Zina G. Azelaic acid therapy in disorders of pigmentation. Clin Dermatol. 1989; 7:106–119.


5. Verallo-Rowell VM, Verallo V, Graupe K, Lopez-Villafuerte L, Garcia-Lopez M. Double-blind comparison of azelaic acid and hydroquinone in the treatment of melasma. Acta Derm Venereol Suppl (Stockh). 1989; 143:58–61.
6. Jimbow K. N-acetyl-4-S-cysteaminylphenol as a new type of depigmenting agent for the melanoderma of patients with melasma. Arch Dermatol. 1991; 127:1528–1534.


7. Neering H. Treatment of melasma (chloasma) by local application of a steroid cream. Dermatologica. 1975; 151:349–353.


8. Griffiths CE, Finkel LJ, Ditre CM, Hamilton TA, Ellis CN, Voorhees JJ. Topical tretinoin (retinoic acid) improves melasma. A vehicle-controlled, clinical trial. Br J Dermatol. 1993; 129:415–421.


9. Kimbrough-Green CK, Griffiths CE, Finkel LJ, Hamilton TA, Bulengo-Ransby SM, Ellis CN, et al. Topical retinoic acid (tretinoin) for melasma in black patients. A vehicle-controlled clinical trial. Arch Dermatol. 1994; 130:727–733.


10. Lima LL, Lima RM, da Silva AF, do Carmo AM, da Silva AD, Raposo NR. Azastilbene analogs as tyrosinase inhibitors: new molecules with depigmenting potential. ScientificWorldJournal. 2013; 2013:274643.


11. Saghaie L, Pourfarzam M, Fassihi A, Sartippour B. Synthesis and tyrosinase inhibitory properties of some novel derivatives of kojic acid. Res Pharm Sci. 2013; 8:233–242.
12. Schurink M, van Berkel WJ, Wichers HJ, Boeriu CG. Novel peptides with tyrosinase inhibitory activity. Peptides. 2007; 28:485–495.


13. Chen JS, Wei C, Marshall MR. Inhibition mechanism of kojic acid on polyphenol oxidase. J Agric Food Chem. 1991; 39:1897–1901.


14. Kobayashi Y, Kayahara H, Tadasa K, Nakamura T, Tanaka H. Synthesis of amino acid derivatives of kojic acid and their tyrosinase inhibitory activity. Biosci Biotech Biochem. 1995; 59:1745–1746.


15. Kadokawa J, Nishikura T, Muraoka R, Tagaya H, Fukuoka N. Synthesis of kojic acid derivatives containing phenolic hydroxy groups. Commun. 2003; 33:1081–1086.


16. Kitano T, Tada H, Nishimura T, Teramukai S, Kanai M, Nishimura T, et al. Prevalence and incidence of anemia in Japanese cancer patients receiving outpatient chemotherapy. Int J Hematol. 2007; 86:37–41.


17. Manga P, Orlow SJ. Inverse correlation between pink-eyed dilution protein expression and induction of melanogenesis by bafilomycin A1. Pigment Cell Res. 2001; 14:362–367.


18. Kubo I, Kinst-Hori I. Tyrosinase inhibitory activity of the olive oil flavor compounds. J Agric Food Chem. 1999; 47:4574–4578.


19. Luo LH, Kim HJ, Nguyen DH, Lee HB, Lee NH, Kim EK. Depigmentation of melanocytes by (2Z,8Z)-matricaria acid methyl ester isolated from Erigeron breviscapus. Biol Pharm Bull. 2009; 32:1091–1094.


20. Park S, Morya VK, Nguyen DH, Singh BK, Lee HB, Kim EK. Unrevealing the role of P-protein on melanosome biology and structure, using siRNA-mediated down regulation of OCA2. Mol Cell Biochem. 2015; 403:61–71.


SUPPLEMENTARY MATERIALS
Supplementary data can be found via http://anndermatol.org/src/sm/ad-28-555-s001.pdf.