Abstract
Background
Arachidonic acid (AA) is an omega-6 polyunsaturated fatty acid present in all mammalian cell membranes, and involved in the regulation of many cellular processes, including cell survival, angiogenesis, and mitogenesis. The dermal papilla, composed of specialized fibroblasts located in the bulb of the hair follicle, contributes to the control of hair growth and the hair cycle.
Objective
This study investigated the effect of AA on hair growth by using in vivo and in vitro models.
Methods
The effect of AA on human dermal papilla cells (hDPCs) and hair shaft elongation was evaluated by MTT assay and hair follicle organ culture, respectively. The expression of various growth and survival factors in hDPCs were investigated by western blot or immunohistochemistry. The ability of AA to induce and prolong anagen phase in C57BL/6 mice was analyzed.
Results
AA was found to enhance the viability of hDPCs and promote the expression of several factors responsible for hair growth, including fibroblast growth factor-7 (FGF-7) and FGF-10. Western blotting identified the role of AA in the phosphorylation of various transcription factors (ERK, CREB, and AKT) and increased expression of Bcl-2 in hDPCs. In addition, AA significantly promoted hair shaft elongation, with increased proliferation of matrix keratinocytes, during ex vivo hair follicle culture. It was also found to promote hair growth by induction and prolongation of anagen phase in telogen-stage C57BL/6 mice.
Arachidonic acid (AA) is an omega-6 polyunsaturated fatty acid (PUFA) present in an esterified form in all mammalian cell memranes , and is released for signaling purposes by the action of phospholipases12. Free AA is made available as a substrate for the intercellular biosynthesis of various eicosanoids, such as prostaglandins (PGs), thromboxanes, leukotrienes (LTs), and hydroxyeicosatetraenoic acids (HETEs), through the action of cyclooxygenases, lipoxygenases, and cytochrome P-450, respectively23. AA and its eicosanoid metabolites are involved in the regulation of many cellular processes, such as cell survival, angiogenesis, chemotaxis, mitogenesis, apoptosis, and migration4567.
The hair follicle (HF) is a mini-organ unique to mammals where a single HF can represent the entire neuroectodermal-mesodermal interaction system. In its lifetime, a HF undergoes cyclic transformations between phases of rapid growth (anagen), regression (catagen), and resting (telogen). The dermal papilla (DP), which is composed of specialized groups of fibroblasts located in the follicle's bulb, is important in this context for its role in controlling hair growth and cycling8910.
Animal and plant oils, which are rich in polyunsaturated fatty acids, are used in various countries as traditional remedies for skin irritations, rheumatic symptoms, and hair loss. In addition, several case reports have described clinical improvement in scalp dermatitis, alopecia, and hair depigmentation with topical application of safflower oil (which is rich in omega-6 polyunsaturated fatty acid, the linoleic-acid-precursor of AA). These conditions are established symptoms of essential fatty acid deficiency syndrome1112. Several recent studies have also demonstrated that hair growth can be promoted by using latanoprost and isopropyl unoprostone, which are analogues of PGF2α, which in turn is one of the major metabolite of AA13. However, the direct effects of AA and its eicosanoid metabolites on human dermal papilla cells (hDPCs) have not been reported.
We investigated the effects of AA on hair growth by analyzing its role in the proliferation of hDPCs and hair shaft elongation during human HF culture. We also studied the effect of AA on the expression of various growth and survival factors in hDPCs, and its ability to induce and prolong anagen in C57BL/6 mice.
The study design was reviewed and approved by the Institutional Review Board of Seoul National University Hospital (approval number C-1312-078-541), and included written informed consents from all human volunteers. All experimental procedures that used human materials were conducted in compliance with the ethical principles of the Helsinki Declaration. All animal experiments were performed in accordance with the national and institutional guidelines for animal care, at the animal facility of Seoul National University Hospital.
Lyophilized AA was procured from Cayman Chemicals (Ann Arbor, MI, USA), and dissolved in ethanol to create stock solutions of 100, 10, 5 mM, and 1 mM (1 mM=1 mmol/L). The stock solutions were stored at -20℃ until their use. For the animal experiments, 3% minoxidil (MNX) was obtained from Hyundai Pharm. Ind., Co., Ltd. (Cheonan, Korea).
Skin biopsy specimens (1.5×1.0 cm scalp tissue samples from the occipital region) were obtained from healthy male volunteers (age, 20~50 years) who did not have any current or prior scalp disease. The HFs were isolated under a dissecting stereomicroscope (Olympus, Tokyo, Japan), and the hDPCs were isolated from individual HFs that were determined to be in the anagen stage as described by a standardized technique in previous studies1415.
As previously described1516, The hDPCs were cultured at 37℃ (5% CO2) in Dulbecco's modified Eagle's medium (DMEM; Welgene, Daegu, Korea), supplemented with 10% fetal bovine serum (Welgene), 10 ng/ml of basic fibroblast growth factor (bFGF; R&D Systems, Minneapolis, MN, USA), and antibiotic/antimycotic 1X solution (100 mg/ml streptomycin and 100 U/ml penicillin).
Viability of the hDPCs was determined by using the MTT (3-[4,5-dimethylthiazol-2-yl]-2,5-diphenyltetrazolium bromide) assay. Briefly, hDPCs (1×104 cells/well) were seeded into 96-well plates, cultured for 24 hours in serum-free DMEM, and then treated for 24 hours with the vehicle (ethanol at a diluted to 1:1,000 in serum-free DMEM) or with AA (1~50 µM [final concentration], diluted to 1:1,000 in serum-free DMEM). Subsequently, 20 µl of MTT solution (5 mg/ml) was added to each well, and the plate was incubated for 3 hours at 37℃. The supernatant containing the MTT was removed, and the formazan crystals in the wells were dissolved by adding 200 µl of dimethyl sulfoxide. The samples were finally incubated for 30 minutes at room temperature before a plate reader enabled quantitative analysis for viable cells by optical density measurement at 570 nm.
Human scalp HFs were isolated and cultured as previously described17. Each HF was cut at the level of the sebaceous duct, and then cultured for 12 days at 37℃ (5% CO2) in Williams' E medium (Gibco-BRL laboratories, Grand Island, NY, USA), supplemented with 10 ng/ml hydrocortisone, 10 µg/ml insulin, 2 mM L-glutamine, and antibiotic/antimycotic 1X solution (penicillin and streptomycin, Gibco-BRL laboratories). AA was added to culture medium at final concentrations of 1, 2, 5 µM, or 10 µM. On every third day, the culture medium was changed and the elongation of the hair shaft in each HF was measured by using a stereomicroscope (Olympus). A total of 400 HFs from 5 different volunteers (80 HFs per volunteer) were analyzed for each growth condition (based on the concentration of AA solution).
Immunohistochemistry and immunofluorescence staining were performed with 5 µm paraffin sections of human HFs, as previously described18. The antibodies used were anti-FGF-7 and anti-FGF-10 (Santa Cruz Biotechnology, Santa Cruz, CA, USA). Anti-Ki-67 (DAKO, Carpentaria, CA, USA) was used as an indicator of cell proliferation, and DAPI (4',6-diamidino-2-phenylindole) mounting media kit (Vector Laboratories, Burlingame, CA, USA) was used to counterstain the nuclei.
Total RNA was isolated from the hDPCs by using RNAiso Plus (Takara Bio Inc., Otsu, Japan) and was treated with DNase I (Roche Pharmaceuticals, Welwyn Garden City, UK) to remove the genomic DNA. Subsequently 1~2 µg of total RNA was used for the cDNA synthesis reaction, performed by using the First Strand cDNA Synthesis Kit (Fermentas, St. Leon-Rot, Germany) according to the manufacturer's instructions. Quantitative estimation of mRNA expression was performed by real-time polymerase chain reaction by using a 7500 Real-Time PCR System (Applied Biosystems, Foster City, CA, USA) with SYBR Premix Ex Taq (Takara Bio Inc.), according to the manufacturers' instructions. All experiments with SYBR Green were performed in triplicate and were independently repeated thrice.
The total protein from the hDPCs was extracted by using RIPA lysis buffer (Millipore, Billerica, MA, USA), according to the manufacturer's instructions. Proteins were separated by using 10% and 15% sodium dodecyl sulfate polyacrylamide gel electrophoresis, and were transferred to a polyvinylidene fluoride membrane (Amersham, Buckinghamshire, UK) by using a wet transfer system. The blotted membranes were incubated at 4℃ with the appropriate antibodies: anti-total extracellular signal-related kinase (ERK), anti-phosphorylated ERK, anti-total protein kinase B (Akt), anti-phosphorylated AKT, anti-B-cell lymphoma 2 (Bcl-2), anti-total cyclic AMP response element-binding protein (CREB), anti-phosphorylated CREB (Cell Signaling Technology, Beverly, MA, USA), and anti-β-actin (Santa Cruz Biotechnology). The membranes were then probed with anti-mouse, anti-rabbit, or anti-goat IgG-horseradish peroxidase conjugates (Santa Cruz Biotechnology) for 1 hour at room temperature. Antibody-antigen complexes were then detected by using the enhanced chemiluminescence system (Amersham Pharmacia Biotech, Little Chalfont, UK).
The methods that we used for the anagen induction assay has been described in previous studies1920. Briefly, 8-week-old female C57BL/6 mice (5 mice/group) that were in the telogen phase were selected. On their shaved backs, 200 µl of AA (2%), the vehicle (negative control), or 3% MNX (positive control) were topically applied every day for a 4-week period, as in the previously reported in vivo studies with PUFA2122. The skin over the back was observed every day and photographed every week. Skin biopsies were performed from the treated area on day 28 and assessed for anagen hairs by histological examination with hematoxylin and eosin staining.
The back skin of 8-week-old female C57BL/6 mice in the telogen phase was depilated by waxing, as described in the previously reported studies2324. All HFs in the depilated area entered the anagen VI stage after day 10. The mice were divided into three groups of five mice each. Each mouse received a topical application of either AA (2%), vehicle, or MNX (3%) on its back skin every day for the next 10 days. Biopsies were performed on day 21, and stained with hematoxylin and eosin. The hair cycle score was calculated by assigning the following arbitrary scores, as described in previous studies24: HFs in anagen VI, 100; HFs in early catagen (catagen I~III), 200; HFs in mid-catagen (catagen IV~V), 300; and HFs in late catagen (catagen VI~VIII), 400. Fifty HFs were identified for each mouse and graded on the biopsy specimens. The Image J program (National Institutes of Health, Bethesda, MD, USA) was used for quantitative analysis.
Statistical significance was determined by using Student's t-test. Paired t-test was used to compare the HF culture results. All tests were two-tailed, and differences with a p-value of <0.05 were considered statistically significant. The statistical analyses were done by using the IBM SPSS Statistics 21.0 software package (IBM Co., Armonk, NY, USA).
MTT assay was used to evaluate the effect of AA on survival of hDPCs. In this experiment, AA (1~5 µM) significantly enhanced viability of hDPCs compared to the vehicle-treated controls (Fig. 1A). However, the viability of hDPCs decreased at concentrations of AA greater than 10 µM.
In addition, hair shaft elongation by treating culturing human HFs with AA (1~10 µM) for 12 days was analyzed. In this experiment, AA, at concentrations of 1, 2 µM, and 5 µM significantly enhanced hair shaft elongation after 6, 9, and 12 days, compared to the vehicle-treated controls (Fig. 1B).
To analyze the proliferation of human HFs, immunofluorescence staining was performed for Ki-67 (a proliferation marker) after HFs were cultured with AA for 3 days. The number of cells among the follicular matrix keratinocytes that were positive for Ki-67 were counted and this number was normalized by using the number of DAPI positive cells. Treatment with AA (1~5 µM) significantly increased the number keratinocytes positive for Ki-67 (Fig. 1C and D).
After treatment with AA (1, 2 µM, and 5 µM) for 24 hours, the levels of mRNA expression for FGF-7 and FGF-10, which are known to stimulate hair growth252627, exhibited a marked increase in a dose-dependent manner. In addition, the expression of hepatocyte growth factor (HGF) mRNA, another potent inducer of hair growth2829, also significantly increased (Fig. 2A). The expression of insulin-like growth factor-1 and vascular endothelial growth factor mRNA were unaffected (data not presented).
Immunohistochemical staining for FGF-7 and FGF-10 was performed following treatment of HFs with AA for 3 days. The expression of FGF-7 increased in the DP cytoplasm of 1, 2 µM, and 5 µM solutions of AA. The expression of FGF-10 increased in DP treated with 2 µM and 5 µM solutions of AA (Fig. 2B).
In addition, Western blotting was performed to analyze the expression of various signaling molecules that are related to cell proliferation and survival, such as mitogen-activated protein kinase ERK, CREB, AKT, and Bcl-2233031. For Western blotting, hDPCs on passage 4~5 under subculture were used. In this experiment, AA treatment enhanced phosphorylation of ERK, CREB, and AKT, and increased the expression of Bcl-2 (Fig. 2C and D). These results indicate that AA affects intracellular signaling cascades and prevents cell death by increasing the phosphorylation of ERK and promoting cell survival by up-regulation of AKT phosphorylation and Bcl-2 expression.
To investigate effect of AA on hair growth, 8-week-old female C57BL/6 mice were treated with one among AA, MNX, or the vehicle. It should be noted here that the hair of C57BL/6 mice enters the telogen stage at postnatal week 7, which is the optimal time to examine anagen induction without endogenous hair growth32. In this experiment, both, 2% AA and 3% MNX were able to produce a significantly higher level of hair growth at day 28, compared to the controls (Fig. 3A and B). In addition, the change in the color of the back skin and hair re-growth percent, after four weeks, were significantly greater than controls when treated with 2% AA or 3% MNX (Fig. 3B and C). These results indicate that AA and MNX are potent anagen inducers.
The back skin mice were synchronized to the anagen stage by depilation, as all HFs in the depilated skin areas enter the anagen VI stage 10 days after depilation2432. At day 21, after 10 days of treatment (Fig. 4A), histological examination revealed an increase in the size, depth, and length of the HFs that were treated with 2% AA and 3% MNX (Fig. 4B). In addition, the hair cycle scoring revealed markedly prolonged anagen hair growth in the HFs that were treated with 2% AA. However, no significant difference in hair thickness was observed between the three groups (Fig. 4C).
Epithelial-mesenchymal interactions play an important role in the organogenesis of many tissues, including HFs. The DP contains a population of mesenchymal cells that regulate hair matrix keratinocytes via various molecular signals, including growth factors33. In the present study, we found that AA increased the expression of FGF-7, FGF-10, and HGF. Both FGF-7 and FGF-10 are paracrine factors that stimulate cell proliferation and survival, and their expression peaks during anagen252634. HGF is a paracrine factor secreted by mesenchymal-derived cells and acts on neighboring epithelial or endothelial cells to regulate the function of various organs, as demonstrated in in vitro studies of human HFs and mouse vibrissae2829. We were able to confirm this activity in our ex vivo culture of HFs, as 3 days of culture with AA increased the proliferation of human hair matrix keratinocytes and the expression of FGF-7 and FGF-10. Therefore, our results suggest that, in cultured hDPCs and ex vivo cultured HFs, treatment with AA affects the signal transduction cascades that mediate cell proliferation via expression of FGF-7, FGF-10, and HGF.
There is a possible direct stimulatory effect of AA on matrix keratinocytes or epithelial cells of outer root sheath (ORS). Several studies have investigated the effect of AA and its metabolites on skin keratinocytes and found that subtypes of PGE receptors, EP2 and EP3, affect the rate of keratinocyte proliferation35. It was also demonstrated that ORS keratinocytes expressed PGE receptors (EP2, EP4), thromboxane A2 receptor (TP) and to a lesser extend PGF2α receptor (FP) and PGD2 receptor (DP2) receptors in anagen human HFs36.
In the Western blot analysis of hDPCs, we observed that AA treatment lead to phosphorylation of various transcription factors (ERK, CREB, and AKT) and increased the expression of Bcl-2; these factors are known to promote survival and prevent cell death233738. Therefore, it appears that AA triggers multiple intracellular signal transduction pathways and affects a variety of cellular functions. We also confirmed that AA treatment significantly affected anagen induction, prolongation, and hair growth in C57BL/6 mice. This may indicate that the topical use of AA promotes hair growth.
In our study, it is unclear how exogenously applied AA exerts the observed effects. Some studies showed that the AA is directly converted by cyclooxygenase, P-450, and lipoxygenase enzymes to its metabolites, which in turn play a role in the regulation of growth and motility in various cell types and the effects of AA depend on the concentration of AA applied and the cell type3940.
PG and its analogues, which are major metabolites of AA, demonstrated modulation of hair growth on their application. PGE2 analogues have been investigated as agents against radiation or doxorubicin induced alopecia in a murine model of hair injury. Both systemic and topical application of a PGE2 analogue resulted in a significant degree of protection against radiation or doxorubicin induced alopecia414243. In another study, application of viprostol, another PGE2 analogue, led to human scalp hair growth supporting the argument that E series PGs may have an effect on hair growth, although to a lesser extent than the F series PGs44. Recent studies have demonstrated promotion of hair growth with the use of latanoprost and isopropyl unoprostone, which are analogues of PGF2α—another major metabolite of AA13. However, the underlying mechanism of latanoprost-associated hair growth is not clearly understood.
In conclusion, our results demonstrate that AA treatment increases the proliferation of hDPCs, promotes the elongation of human hair shafts, and promotes hair growth in a mouse model. These results may be related to the ability of AA to increase the expression of proliferation factors in HFs. Therefore, AA appears to be a potential agent for promoting hair growth.
Figures and Tables
Fig. 1
The effect of arachidonic acid (AA) on viability of cultured human dermal papilla cells (hDPCs). (A) Treatment of hDPCs with AA (1~5 µM) resulted in significantly increased cell viability, as measured by the MTT assay. (B) AA-enhanced hair shaft elongation in ex vivo follicle culture. Human hair follicles (HFs) were treated with the vehicle or AA (1, 2, 5, 10 µM) (n=5); AA significantly enhanced hair shaft elongation. (C, D) The proliferation of matrix keratinocytes increases in AA-treated HFs. Human HFs were cultured with the vehicle or AA (1, 2 µM, or 5 µM) for 3 days, and then subjected to immunofluorescence staining, to examine proliferation in the hair matrix keratinocytes, with Ki-67 (proliferation, green fluorescence), and 4',6-diamidino-2-phenylindole (DAPI, blue fluorescence) to counterstain the nuclei (×200). Data are expressed as mean±standard error. CTL: control. *p≤0.05, **p≤0.01, ***p≤0.01, versus the control group.
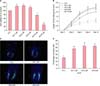
Fig. 2
Arachidonic acid (AA) increases the expression of growth and survival factor genes in human dermal papillary cells (hDPCs). (A) The mRNA for fibroblast growth factors (FGF-7 and -10) and hepatocyte growth factor (HGF) is elevated in hDPCs that were treated with AA (1, 2 µM, or 5 µM) for 24 hours. (B) Treatment with AA increases the expression of FGF-7 and FGF-10 in the cytoplasm of the dermal papilla (DP) and inner root sheath (IRS) on day 3 of its application, compared to the vehicle control (×100). (C and D) Human DPCs were lysed to analyze the total protein content of the lysates via Western blotting by using primary antibodies for total-p42/44 ERK, phospho-p42/44 ERK, total-AKT, phospho-AKT, total-CREB, phospho-CREB, Bcl-2, and β-actin. ERK: extracellular signal-regulated kinases, AKT: protein kinase B, CREB: cAMP response element-binding protein, CTL, control. Data are expressed as mean±standard error. *p≤0.05, **p≤0.01, ***p≤0.01, versus the control group.
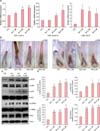
Fig. 3
Enhanced anagen induction in response to arachidonic acid (AA) treatment in 8-week-old female C57BL/6 mice. (A) After their backs were shaved, five mice in each group, were treated either with the vehicle, topical 3% minoxidil (MNX) (positive control), or 2% AA for 4 weeks. (B) Weekly representation of the amount of black colored skin. (C) Weekly representation of the amount of hair-bearing skin. Data are expressed as mean±standard error. CTL: control, D0: first day after the back was shaved, W: week.*p≤0.05, ***p≤0.001, versus the control group.
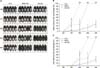
Fig. 4
Arachidonic acid (AA) prolongs the anagen hair cycle. (A) The experiment design. Ten days after depilation by waxing, the back skin of C57BL/6 mice was treated with either with the vehicle, topical 3% minoxidil (MNX), or 2% AA. (B) On day 21 after depilation, biopsied skin samples were fixed in paraffin, sectioned and stained with hematoxylin and eosin (×40). (C) Calculating the hair cycle scores. For each mouse (5 mice per group), 50 hair follicles (HFs) from each section were analyzed and graded as follows: anagen VI, 100; early catagen, 200; mid catagen, 300; late catagen, 400. The score indicates the mean hair cycle stage for all HFs in a group. Data are expressed as mean±standard error. CTL: control. *p≤0.05, ***p≤0.001 versus the control group.
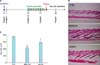
ACKNOWLEDGMENT
This study was supported by a grant (Grant No. HN10C0009) from the Korean Health Technology R&D Project, Ministry of Health & Welfare, Republic of Korea and by a grant (No. 04-2015-0530) from Seoul National University Hospital Research Fund.
References
1. Paine E, Palmantier R, Akiyama SK, Olden K, Roberts JD. Arachidonic acid activates mitogen-activated protein (MAP) kinase-activated protein kinase 2 and mediates adhesion of a human breast carcinoma cell line to collagen type IV through a p38 MAP kinase-dependent pathway. J Biol Chem. 2000; 275:11284–11290.


2. Clària J. Regulation of cell proliferation and apoptosis by bioactive lipid mediators. Recent Pat Anticancer Drug Discov. 2006; 1:369–382.


3. Cabral M, Martín-Venegas R, Moreno JJ. Role of arachidonic acid metabolites on the control of non-differentiated intestinal epithelial cell growth. Int J Biochem Cell Biol. 2013; 45:1620–1628.


4. Bogatcheva NV, Sergeeva MG, Dudek SM, Verin AD. Arachidonic acid cascade in endothelial pathobiology. Microvasc Res. 2005; 69:107–127.


5. Tragni E, Caruso D, Porta S, Fumagalli R, Galli G, Galli CL. Arachidonic acid metabolism in HEL/30 murine epidermal cell line. Arch Dermatol Res. 1988; 280:437–442.


6. Wen ZH, Su YC, Lai PL, Zhang Y, Xu YF, Zhao A, et al. Critical role of arachidonic acid-activated mTOR signaling in breast carcinogenesis and angiogenesis. Oncogene. 2013; 32:160–170.


7. Nishizawa Y, Nishii K, Kishimoto S, Matsumoto K, Sato B. Regulatory role of arachidonic acid-derived metabolites for proliferation of transformed murine Leydig cell in serum-free culture condition. Anticancer Res. 1990; 10:317–322.
8. Paus R, Foitzik K. In search of the "hair cycle clock": a guided tour. Differentiation. 2004; 72:489–511.


11. Skolnik P, Eaglstein WH, Ziboh VA. Human essential fatty acid deficiency: treatment by topical application of linoleic acid. Arch Dermatol. 1977; 113:939–941.


12. Janssen CI, Kiliaan AJ. Long-chain polyunsaturated fatty acids (LCPUFA) from genesis to senescence: the influence of LCPUFA on neural development, aging, and neurodegeneration. Prog Lipid Res. 2014; 53:1–17.


13. Sasaki S, Hozumi Y, Kondo S. Influence of prostaglandin F2alpha and its analogues on hair regrowth and follicular melanogenesis in a murine model. Exp Dermatol. 2005; 14:323–328.


14. Kwon OS, Pyo HK, Oh YJ, Han JH, Lee SR, Chung JH, et al. Promotive effect of minoxidil combined with all-trans retinoic acid (tretinoin) on human hair growth in vitro. J Korean Med Sci. 2007; 22:283–289.


15. Messenger AG. The culture of dermal papilla cells from human hair follicles. Br J Dermatol. 1984; 110:685–689.


16. Choi SJ, Cho AR, Jo SJ, Hwang ST, Kim KH, Kwon OS. Effects of glucocorticoid on human dermal papilla cells in vitro. J Steroid Biochem Mol Biol. 2013; 135:24–29.


18. Yoon SY, Kim KT, Jo SJ, Cho AR, Jeon SI, Choi HD, et al. Induction of hair growth by insulin-like growth factor-1 in 1,763 MHz radiofrequency-irradiated hair follicle cells. PLoS One. 2011; 6:e28474.


19. Paus R, Stenn KS, Link RE. The induction of anagen hair growth in telogen mouse skin by cyclosporine A administration. Lab Invest. 1989; 60:365–369.
20. Jo SJ, Choi SJ, Yoon SY, Lee JY, Park WS, Park PJ, et al. Valproic acid promotes human hair growth in in vitro culture model. J Dermatol Sci. 2013; 72:16–24.


21. Kim HH, Cho S, Lee S, Kim KH, Cho KH, Eun HC, et al. Photoprotective and anti-skin-aging effects of eicosapentaenoic acid in human skin in vivo. J Lipid Res. 2006; 47:921–930.


22. Jin XJ, Kim EJ, Oh IK, Kim YK, Park CH, Chung JH. Prevention of UV-induced skin damages by 11,14,17-eicosatrienoic acid in hairless mice in vivo. J Korean Med Sci. 2010; 25:930–937.


23. Yoon SY, Yoon JS, Jo SJ, Shin CY, Shin JY, Kim JI, et al. A role of placental growth factor in hair growth. J Dermatol Sci. 2014; 74:125–134.


24. Kwack MH, Kang BM, Kim MK, Kim JC, Sung YK. Minoxidil activates β-catenin pathway in human dermal papilla cells: a possible explanation for its anagen prolongation effect. J Dermatol Sci. 2011; 62:154–159.


25. du Cros DL. Fibroblast growth factor and epidermal growth factor in hair development. J Invest Dermatol. 1993; 101:1 Suppl. 106S–113S.


26. Marchese C, Felici A, Visco V, Lucania G, Igarashi M, Picardo M, et al. Fibroblast growth factor 10 induces proliferation and differentiation of human primary cultured keratinocytes. J Invest Dermatol. 2001; 116:623–628.


27. Danilenko DM, Ring BD, Yanagihara D, Benson W, Wiemann B, Starnes CO, et al. Keratinocyte growth factor is an important endogenous mediator of hair follicle growth, development, and differentiation. Normalization of the nu/nu follicular differentiation defect and amelioration of chemotherapy-induced alopecia. Am J Pathol. 1995; 147:145–154.
28. Shimaoka S, Tsuboi R, Jindo T, Imai R, Takamori K, Rubin JS, et al. Hepatocyte growth factor/scatter factor expressed in follicular papilla cells stimulates human hair growth in vitro. J Cell Physiol. 1995; 165:333–338.


29. Jindo T, Tsuboi R, Imai R, Takamori K, Rubin JS, Ogawa H. Hepatocyte growth factor/scatter factor stimulates hair growth of mouse vibrissae in organ culture. J Invest Dermatol. 1994; 103:306–309.


30. Hicklin DJ, Ellis LM. Role of the vascular endothelial growth factor pathway in tumor growth and angiogenesis. J Clin Oncol. 2005; 23:1011–1027.


31. Hwang KA, Hwang YL, Lee MH, Kim NR, Roh SS, Lee Y, et al. Adenosine stimulates growth of dermal papilla and lengthens the anagen phase by increasing the cysteine level via fibroblast growth factors 2 and 7 in an organ culture of mouse vibrissae hair follicles. Int J Mol Med. 2012; 29:195–201.


32. Müller-Röver S, Handjiski B, van der Veen C, Eichmüller S, Foitzik K, McKay IA, et al. A comprehensive guide for the accurate classification of murine hair follicles in distinct hair cycle stages. J Invest Dermatol. 2001; 117:3–15.


33. Botchkarev VA, Kishimoto J. Molecular control of epithelialmesenchymal interactions during hair follicle cycling. J Investig Dermatol Symp Proc. 2003; 8:46–55.


34. Kawano M, Komi-Kuramochi A, Asada M, Suzuki M, Oki J, Jiang J, et al. Comprehensive analysis of FGF and FGFR expression in skin: FGF18 is highly expressed in hair follicles and capable of inducing anagen from telogen stage hair follicles. J Invest Dermatol. 2005; 124:877–885.


35. Konger RL, Malaviya R, Pentland AP. Growth regulation of primary human keratinocytes by prostaglandin E receptor EP2 and EP3 subtypes. Biochim Biophys Acta. 1998; 1401:221–234.


36. Colombe L, Michelet JF, Bernard BA. Prostanoid receptors in anagen human hair follicles. Exp Dermatol. 2008; 17:63–72.


37. Iino M, Ehama R, Nakazawa Y, Iwabuchi T, Ogo M, Tajima M, et al. Adenosine stimulates fibroblast growth factor-7 gene expression via adenosine A2b receptor signaling in dermal papilla cells. J Invest Dermatol. 2007; 127:1318–1325.


38. Ahn SY, Pi LQ, Hwang ST, Lee WS. Effect of IGF-I on hair growth is related to the anti-apoptotic effect of IGF-I and up-regulation of PDGF-A and PDGF-B. Ann Dermatol. 2012; 24:26–31.


39. Brash AR, Ingram CD. Lipoxygenase metabolism of endogenous and exogenous arachidonate in leukocytes: GC-MS analyses of incubations in H2(18)O buffers. Prostaglandins Leukot Med. 1986; 23:149–154.


40. Sautebin L, Caruso D, Galli G, Paoletti R. Preferential utilization of endogenous arachidonate by cyclo-oxygenase in incubations of human platelets. FEBS Lett. 1983; 157:173–178.


41. Geng L, Hanson WR, Malkinson FD. Topical or systemic 16, 16 dm prostaglandin E2 or WR-2721 (WR-1065) protects mice from alopecia after fractionated irradiation. Int J Radiat Biol. 1992; 61:533–537.


42. Hanson WR, Pelka AE, Nelson AK, Malkinson FD. Subcutaneous or topical administration of 16,16 dimethyl prostaglandin E2 protects from radiation-induced alopecia in mice. Int J Radiat Oncol Biol Phys. 1992; 23:333–337.

