Abstract
Variation in human skin and hair color is the most notable aspect of human variability and several studies in evolution, genetics and developmental biology contributed to explain the mechanisms underlying human skin pigmentation, which is responsible for differences in skin color across the world's populations. Despite skin pigmentation is primarily related to melanocytes functionality, the surrounding keratinocytes and extracellular matrix proteins and fibroblasts in the underlying dermal compartment actively contribute to cutaneous homeostasis. Many autocrine/paracrine secreted factors and cell adhesion mechanisms involving both epidermal and dermal constituents determine constitutive skin pigmentation and, whenever deregulated, the occurrence of pigmentary disorders. In particular, an increased expression of such mediators and their specific receptors frequently lead to hyperpigmentary conditions, such as in melasma and in solar lentigo, whereas a defect in their expression/release is related to hypopigmented disorders, as seen in vitiligo. All these interactions underline the relevant role of pigmentation on human evolution and biology.
Understanding the fascinating history of pigmentation biology requires the integration of evolution, genetics, and developmental biology. In vertebrates, pigmentation varies widely from black and white stripes of zebra to muted browns in sparrows to bright colors of tropical fish. Thus, the pigmentation system has served as a model for a wide variety of studies providing some connections between genotype and phenotype for evolutionary important pigmentation traits. Skin color primarily depends on the functions of melanocytes, the cells specialized in the synthesis and distribution of the melanin pigment. In the epidermis, each melanocyte is connected through its dendrites with approximately 30~40 keratinocytes, establishing the so-called epidermal melanin unit1, and to fibroblasts in the underlying dermis. Within melanocytes, melanin synthesis occurs in specialized membrane-bound organelles termed melanosomes, characterized by four stages of maturation, along with they gradually become pigmented. Specialized melanogenic enzymes within melanosomes, tyrosinase and tyrosinase-related ones, direct the production of two melanin types: black-brown eumelanins, the major pigment found in dark skin and hair, and yellow/red pheomelanin, mainly observed in red hair and I/II skin phototypes2. The number of melanocytes is relatively comparable among different ethnicities34 and variations in skin color are mainly dependent on the type (the relative quantity of pheo and eumelanins) and the amount of melanin produced within melanosomes. Size, quantity, distribution and degradation of these structures in the surrounding keratinocytes are also determinants in ethnic color differences. In light skin, melanosomes are smaller and mainly at an early stage of maturation (stages I and II). They are transferred to keratinocytes as clusters and are degraded at the level of the spinous layer. In dark skin, these structures are larger, at stage IV and are singly transferred to the surrounding cells. Their degradation appears also slower than that in the light skin, being some melanins yet observed in the stratum corneum56. Moreover, the ratio between pheo and eumelanins appears higher in dark versus light skins (Fig. 1)7. Besides the underlying biological bases, variation in human skin and hair color is the most notable aspect of human variability and explaining which mechanisms support differences in skin color across the world's populations has been one of the central questions of human biology. The association of dark skin pigmentation with intense sunshine and heat was postulated by Aristotle into a "climatic theory" which related human features to the environment. Later, naturalists such as John Mitchell and Samuel Stanhope Smith related differences in skin pigmentation of world's people (from light toward the poles to dark near the equator) to the latitudinal gradient sunshine heat89. Today we can say more precisely that variations in skin color are adaptive and related to ultraviolet (UV) radiation levels, correlate with latitude and represent a solution to the physiological requirements of photoprotection and vitamin D synthesis10.
It is likely that the integument of early members of the hominid family was white or lightly pigmented and covered with dark hair. The evolution of a naked, darkly pigmented integument occurred to protect sweat glands from UV-induced injury thus ensuring the quality of somatic thermoregulation. The migration of hominids outside of the tropics placed pressure on skin pigmentation levels because of lower and more seasonal levels of UV. Simultaneously, many degrees of depigmentation evolved to allow UV-B-induced synthesis of vitamin D. Therefore, the range of pigmentation observed in modern humans evolved to favor photoprotection near the equator and to promote cutaneous UV-R-induced vitamin D synthesis closer to the poles.
Several studies in population genetics have allowed to explain the evolution of human pigmentation system. However, the genetics of pigmentation is complex: many genes, that have been identified for decades by using mouse coat color mutations (and more recently zebrafish), are responsible for the range of pigmentation phenotypes contributing to human skin pigment diversity seen both between and within human populations11. Moreover, variants of some of these genes or single-nucleotide polymorphisms (SNPs) close to them have been found to contribute to normal variation in pigmentary phenotype. Over the past 10 years, the development of high-throughput sequencing technology has allowed to identify associations between pigmentary characteristics and multiple loci across whole genomes. Such genome-wide scans have both confirmed a role for genes previously identified as candidates in human pigmentation and highlighted new loci, thus determining more than one hundred genes that regulate human pigmentary system either directly or indirectly. The expression of such genes is not necessarily confined to melanocytes1213.
Melanocortin 1 receptor (Mc1R), a G protein-coupled receptor that regulates the quantity and quality of melanins produced, was the first gene identified in humans that is involved in regulating mammalian skin and hair color14. It works by controlling the type of melanin being produced, and its activation causes the switch from phaeomelanin to eumelanin. Mc1R is preferentially expressed in melanocytes but not exclusively limited to them since low levels of Mc1R are also detected in keratinocytes, fibroblasts, and immune cells15. Several studies showed that Mc1R is highly polymorphic in Northern European populations, whereas is almost without any functionally significant variation in African populations demonstrating the action of selection to maintain dark pigmentation in high-UV-R environments1617.
The development and evolution of such a complex pigmentary system required a fine and integrated regulation of signalling pathways and cellular interactions. Several autocrine/paracrine secreted factors and cell adhesion mechanisms involving both epidermal and dermal constituents, regulate melanocyte homeostasis, determining the constitutive skin pigmentation and, whenever deregulated, the occurrence of pigmentary disorders. Some of these messengers are produced by both the epidermal and dermal cells, whereas others are distinctly synthesized by only one type of cell. UV exposure is the main environmental factor influencing skin pigmentation. Following UV irradiation, pigment redistribution in keratinocytes and skin is stimulated, melanogenesis is increased and the synthesis and secretion of many pro-pigmenting factors are up-regulated. All these factors act by binding to their specific receptors followed by activation of intracellular signalling pathways linked to melanocyte functionality. Among such growth factor/receptor signalling cascades, one of the main pathway is constituted by the pro-opiomelanocortin (POMC) derived peptides (melanocyte-stimulating hormone [MSH] and adrenocorticotropic hormone) from keratinocytes and their receptor Mc1R. Following UV exposure the transcription of POMC is stimulated by the induction of the tumor suppressor protein p53 in keratinocytes, thus promoting melanin synthesis in melanocytes via paracrine interactions18. As previously mentioned, Mc1R mutations, which are more frequently observed in light than in dark skin phototypes, may deregulate the cellular responses to α-MSH, resulting in decreased eumelanin production in both constitutive and UV-mediated pigmentation.
Along with α-MSH, melanocyte growth, differentiation, survival and activity are regulated by other keratinocyte-derived growth factors and cytokines including endothelin (ET)-1, basic fibroblast growth factor (bFGF), stem cell factor (SCF), granulocyte-macrophage colony-stimulating factor (GM-CSF), hepatocyte growth factor (HGF). As well as α-MSH, most of these messengers are up-regulated in response to UV: ET-1 binds to its specific receptor endothelin B receptor on melanocytes but it also enhances the expression of Mc1R19. Its effect results in the increase of both melanocyte proliferation and melanogenesis1920 and, as for POMC, ET-1 synthesis is induced by p5321. Upon specific stimuli, e.g. during inflammatory processes, several other mediators, including prostaglandins E2 and F2alpha, thromboxane B2, leukotriene (LT) C4 and LTD4 are released by the surrounding microenvironment and activate melanocytes increasing their dendricity and melanin production22. Adding complexity to the interplay between keratinocytes and melanocytes, a contribution of the eumelanin intermediate 5,6-dihydroxyindole-2-carboxylic acid, which goes beyond the supplying of pigments, has been shown: the diffusion of this chemical messenger from the producing melanocytes into keratinocytes may provide protection against environmental stimuli such as UV, through the increase of the antioxidant defence systems and differentiation in keratinocytes, thus contributing to the overall epidermis protection23.
Some studies highlight the role of beta defensins, small and hydrophobic members of highly conserved gene families exhibiting antimicrobial activity against a variety of gram-negative and gram-positive bacteria, yeast and viruses, in mediating also human pigmentation. More precisely, some results expand the functional role of beta defensins revealing the cross-talk between beta defensins and the melanocortin system. In fact, at least two human beta defensins, human β-defensin (hBD) 1 and hBD3 may modulate melanocortin receptor signaling in vivo, acting not only on Mc1R but also on Mc5R24. Abdel Malek's group defined the role of hBD3 on human pigmentation testing its effects on cultured human melanocytes. HBD3 inhibited α-MSH-induced, but not the forskolin-induced increase in cyclic adenosine monophosphate (cAMP) levels, thus suggesting that hBD3 acted by blocking the Mc1R25. Moreover, dysregulated expression of hBD3 in the skin may also impair DNA repair responses in UV-induced melanocytes and, consequently, improve melanoma risk26.
Parallel to the paracrine influences via keratinocytes, melanocyte functionality and skin pigmentation are regulated by the active role of both extracellular matrix (ECM) proteins and fibroblasts of the dermal compartment. In vitro studies demonstrated the mitogenic and melanogenic influences of ECM proteins such as fibronectin, collagen I and IV on normal human melanocytes and their effect in inhibiting cell vacuolization and death when melanocytes were growing in mitogen-deficient medium27. In addition, laminins and collagen IV are involved in the adhesion of melanocytes to the basement membrane via integrin interactions28. Dermal fibroblasts release many soluble factors able to modulate melanocyte activity: some of these factors are produced also by keratinocytes, such as SCF and HGF, others are specifically synthesized by fibroblasts. Most of these messengers favour melanin synthesis and melanocyte proliferation. Among them, neuregulin-1 is up-regulated in fibroblasts obtained from type VI skin respect to those from types I and III and it represents a melanogenic factor in the regulation of the constitutive pigmentation level of darker skin29. It has been demonstrated that fibroblasts from palmoplantar skin produce higher levels of the Wnt pathway antagonist dickkopf 1 (DKK1) respect to fibroblasts of the trunk. Differently from the growth factors described above, DKK1 exerts an inhibitory effect on melanocyte proliferation and pigment production. Moreover, this soluble factor acts also on keratinocytes, reducing the expression level of the proteinase-activated receptor-2, which is involved in melanosome transfer, thus decreasing also this latter process. These combined effects of DKK1 on both melanocytes and keratinocytes determine the physiological hypopigmentation observed in the epidermis of palms and soles30. The fibroblast growth factor family member keratinocyte growth factor/fibroblast growth factor 7 (KGF/FGF7) induces melanosome transfer, promoting the phagotytic process via activation and signalling of its specific receptor, keratinocyte growth factor receptor, directly in keratinocytes3132. Moreover, this growth factor stimulates the production and secretion of the promelanogenic mediator SCF in primary keratinocytes, creating an indirect paracrine network connecting keratinocytes and fibroblasts in the control of melanocyte functions33. The important role of KGF in promoting skin pigmentation is further demonstrated by additional studies showing the ability of KGF alone and in association with IL1alpha to increase melanin production and deposition in pigmented epidermal equivalents and in human skin explants34. Besides these autocrine and paracrine interactions, melanocyte functions are also influenced by hormonal factors distally produced, as assessed by the variation of pigmentation observed in the course of endocrine changes e.g.: (i) hyperpigmentation detected during pregnancy as a result of increased estrogen levels; (ii) hyperpigmentation of genital areas due to the action of reproductive hormones3536.
One basic function of the skin is the prevention of water loss from the body. This may represent the first physiological demand for protection, but it is equally true that the skin provides a protective barrier against invading microbial pathogens, while also serving as a habitat for a plethora of commensal bacteria. The stratum corneum provides the first line of defense against pathogenic and environmental assaults and, in fact, the barrier functions of the stratum corneum are interrelated, co-regulated and interdependent. The cohesive structure of the stratum corneum, coupled with its low water content and its acidic pH, allows the growth of the normal flora, while providing a formidable layer of the innate immune system that counteracts the invasion of pathogens37.
The recent skin microbiome analysis of monozygotic and dizygotic Korean twins has allowed to identify and discriminate genetic and environmental effects on the microbiome. In particular, the paper reported a strong association between the composition of skin microbiota and human genetic factors related to skin barrier function38.
The stratum corneum is a multilayered tissue composed of flattened and anucleate corneocytes, surrounded by many lamellae sheets, enriched in ceramides, cholesterol, and free fatty acids. The positioning of these highly hydrophobic lipids within the extracellular domains of the stratum corneum inhibits the outside movement of water39. However, the lamellar bodies secrete not only lipids but also proteolytic enzymes and antimicrobial peptides (AMPs) including corneodesmosin, hBD-2 and the cathelicidin product, LL-37 by which, after recognizing microbes via Toll-like receptors or NOD-like receptors, the stratum corneum initiates the defense response. Induction of AMPs can be further increased by cytokines released upon infection and inflammation such as interleukin (IL)-1, IL-17 and IL-224041. In addition, UV radiation has been identified as a potent inducer of AMPs expression in keratinocytes, thus explaining the absence of infection despite the inhibition of adaptive immunity upon UV radiation42. The hypothesis that epidermal pigmentation developed in response to UV-B induced stress to the barrier suggests that enhanced function of pigmented skin can be due to the lower pH of the outer epidermis, probably related to the permanence of melanosomes into the outer epidermis and to the conservation of genes associated with eumelanin synthesis and melanosome acidification in pigmented populations. Accordingly, natural selection would have favored mutations that increase and protect permeability barrier function and, in fact, pigmented skin displays superior barrier function43. On the other hand, the thicknesses of the stratum corneum is higher in not pigmented skin of patients affected by vitiligo, thus giving to the barrier a photoprotective role compensating for absent pigmentation44. Moreover, very recently, a dysbiosis in the diversity of microbial community structure in vitiligo lesional skin has been reported45.
A further corroboration that skin pigmentation is intricately regulated may be also supported by the link, recently demonstrated, between α-MSH and the peroxisome proliferator-activated receptor (PPAR)-γ in melanocytes46. Several lines of evidence indicate that cAMP is the main intracellular messenger responsible for the melanogenic actions of α-MSH47. Moreover, Mc1R signaling recruits c-KIT to activate ERK pathway independently on cAMP in normal human melanocytes and melanoma cells48. α-MSH-induced melanogenesis may also correlate with the canonical Wnt/β-catenin pathway activity49. More recently it has been demonstrated that α-MSH activates PPAR-γ and such activation depends on the induction of the phosphatidylinositol (PI(4,5)P2/PLCβ) signalling pathway. Therefore, the receptor Mc1R drives melanogenesis and proliferation through lipid mediators. In fact, α-MSH treatment failed to reduce cell proliferation rates after PPAR-γ inhibition. In agreement with these data, Kang and co-workers50 investigated in a previous study the expression and function of peroxisome PPARs, which belong to the superfamily of nuclear receptors that heterodimerize with the retinoic X receptor and initially known for their important role in fat metabolism and adipocyte differentiation, on melanocyte proliferation, differentiation and melanogenesis. Moreover, PPAR-γ induces differentiation and increases cell antioxidant defense in response to natural or pharmacological agonists both in melanocytes and melanoma cells51.
Increased production of one or more of pro-melanogenic factors often associated to an altered expression of their receptors have been detected in several hyperpigmentary disorders. Solar/senile lentigines (SLs) are hyperpigmented lesions mainly localized on photodamaged body sites, whose onset is commonly observed along with aging. The underlying mechanisms responsible for the occurence and maintainance of these spots are not yet completely defined. Molecules related to inflammatory and fatty acid metabolism are induced in SL, probably linked to chronical UV exposure. Deregulation in the expression of several pro-melanogenic factors has been demonstrated, involving both keratinocyte- and fibroblast-derived network. In lesional epidermis, ET-1 and its receptor ET-B, SCF and its receptor c-Kit signalling cascades are up-modulated, as well as the expression of α-MSH and Mc1R52. p53 has been shown to play an important role in the onset of these spots through its ability to induce the pro-pigmenting cytokines ET-1, SCF and POMC. In SL lesions, the expression of KGF and its receptor appears to be increased at early-mid stages, suggesting the involvement of this growth factor in the onset of the pigmented spots. Moreover, the treatment with KGF and KGF associated with IL-1alpha, induces hyperpigmented lesions in vivo3453. In SL the induction of pro-melanogenic growth factors signalling cascades has been highlighted also in the dermis, with high expression of KGF, SCF and HGF in lesional fibroblasts. The crucial contribution of the dermis and, specifically, of photoaged fibroblasts in the formation of SL hyperpigmentation are now increasingly strengthened by a number of in vitro models reported in the literature: (i) a higher expression of KGF, SCF and HGF using a model of stress-induced premature senescence in fibroblasts has been reported33; (ii) Salducci et al.54 showed the ability of conditioned medium produced by UV-exposed aged fibroblasts to induce the main SL features in pigmented reconstructed epidermis; (iii) Duval et al.55 demonstrated an increased epidermal pigmentation in a reconstructed skin model containing natural photo-aged fibroblasts. Very recently, an increased expression of KGF in SL has been reported also in the epidermal compartment, supporting the hypothesis that the transfer and accumulation of this fibroblast-derived growth factor into the epidermis may lead to the persistence of hyperpigmentation56. In the progression of this altered dermal-epidermal interplay, it has been suggested that an increased diffusion of heparin-binding growth factors such as FGFs, HGF, GM-CSF from dermis to epidermis and vice versa may be favoured by the reported heparanase-induced degradation of heparan sulfate at the basement membrane observed in solar lentigo57. Alterations in the melanogenic cytokine networks is present also in melasma, an hyperpigmented dysfunction primarily localized on sun-exposed sites, generally the face. Upregulation of genes related to melanogenesis e.g. tyrosinase, TYRP1, TYRP2 and MITF and an increased expression of α-MSH have been detected in lesional epidermis, whereas an over-expression of SCF has been observed in the dermis suggesting, also for this hyperpigmentary disorder, a deregulation of both epidermal and mesenchymal melanogenic cytokine networks leading to melanocyte hyperactivity5859. In addition, the basement membrane in lesional skin appears distrupted and disorganized, with the presence of the so called pendulous melanocytes. The expression of the metalloproteinase 2 is also increased due to the chronic UV exposure and such up-modulation is possibly responsible for the dysfunction of the basement membrane. These changes may, in turn, excessively activate the dermal-epidermal interactions, further underscoring the crucial role of the signalling network among melanocytes and other skin cell populations in the formation of melasma lesions6061. An increased number of blood vessels associated with an up-modulation of the vascular endothelial growth factor have been also detected, highlighting the presence of an altered vascularization in melasma lesions62. Lastly, likewise solar lentigo, accumulation of the melanogenic factor KGF in the lesional epidermis has been observed56. Very recently, an up-regulation of the secreted frizzled-ralated protein 2 (sFRP2) in the skin of melasma, solar lentigo and acutely UV-irradiated skin has been shown. This over-expression leads to increased melanogenesis through the activation of the β-catenin signalling and thus up-regulation of MITF and tyrosinase in melanocytes. Interestingly, a strong expression of sFRP2 was detected in lesional fibroblasts, further demonstrating the crucial role of the epithelial-mesenchymal interplay in the control of physiological and pathological skin pigmentation63. One more, an unbalance of fibroblast-derived growth factors able to stimulate melanocyte and induce pigmentation has been observed in other pigmentary disorders: (i) in the benign fibroblastic tumor dermatofibroma, the hyperpigmentation often observed in the above epidermis, has been attributed to the increased secretion of SCF and HGF by the fibroblastic tumour cells64; (ii) similarly, an heightened release of the same two growth factors by fibroblasts has been suggested to be involved in the epidermal hyperpigmentation of café-au lait macules of neurofibromatosis type 1 (NF1) patients65; (iii) An increased expression of the fibroblast-derived growth factors SCF, HGF and KGF in the dermis of two patients with a generalized, progressive dyschromatosis disorder66. Likewise, the hyperpigmentation sometimes observed in both basal cell carcinoma (BCC) and seborrhoeic keratosis (SK) has been linked to an accentuated expression of the keratinocyte-derived ET-16768 (Fig. 2). Taken together, all these studies show how intricate is the interplay among dermal and epidermal cells with melanocytes and how the variation of one or more of these interactions can result in a variety of different hyperpigmented lesions. On the other hand, changes in the synthesis and release of these messengers has been observed in hypopigmented disorders such as vitiligo. Despite significant studies contributed to a greater knowledge of vitiligo, understanding its pathogenesis is still a challenge. Vitiligo is characterized by the disappearance of functional melanocytes, and multiple mechanisms might contribute to this loss, including genetic defects, metabolic abnormalities, oxidative stress, generation of inflammatory mediators, cell detachment and autoimmune responses. Nevertheless, a growing amount of evidence in recent years argues for intrinsic metabolic defects in melanocytes leading to an intracellular oxidative stress that may act as the initial signal transduction sustaining cell degeneration69. Such metabolic impairment may be not restricted to melanocytes but also extended to dermal cells70. According to the idea that an increase of oxidative stress and impaired ability to effectively manage stressful stimuli are some of the hallmarks of vitiligo skin, melanocytes from non lesional areas show alterations of some pathways, including hyperactivation of mitogen-activated protein kinase (MAPK), cyclic AMP response element-binding protein (CREB), and p53 over-expression, leading to a condition of stress induced premature senescence-like phenotype which is characterized by the production of IL-6, matrix metalloproteinase 3, cyclooxygenase 2, and insulin- like growth factor-binding protein 3 (IGFBP3) and IGFBP771. Among the alterations reported in vitiligo skin, changes in the production of keratinocyte-derived factors have been described by several authors, showing either an up- or a down-modulation: Kitamura et al.72 described an increased expression of ET-1 and SCF transcripts associated with an higher SCF protein expression in lesional versus non-lesional vitiligo epidermis. The authors reported also a selective deficiency in the expression of SCF receptor c-KIT and MITF at the edge of the lesion, suggesting that the reduced function of the SCF/c-KIT signalling cascade may contribute to melanocyte alterations and loss. On the other hand, other groups demonstrated a reduced production of SCF by keratinocytes of the depigmented epidermis, possibly due to a higher vulnerability of these cells to apoptosis. Consequenlty, this reduced secretion of surviving factors may concur to melanocyte death7374. In lesional skin, a lower expression of GM-CSF, bFGF and ET-1, associated with a higher amount of the melanocyte inhibitor cytokines IL-6 and tumor necrosis factor-α have been also reported75 evidencing, altogether, the contribution of keratinocyte alterations in the defective homeostasis and loss of vitiligo melanocytes. Recently, an altered pattern of distribution of the adhesion molecule E-cadherin has been described in clinically normal pigmented skin, indicating the presence of melanocyte adhesiveness impairment that, in presence of stress, may facilitate and contribute to melanocyte loss76. In the dermis, a reduction in the expression of collagen IV and an up-regulation of the ECM protein tenascin have been described, possibly linked to the decreased adhesion of vitiligo melanocytes7778. As well, an higher expression of the melanocyte inhibitor fibroblast-derived factor DKK1 has been reported in lesional versus non lesional dermis79. A lower production and release of KGF by lesional fibroblasts, resulting in a reduction of melanosome transfer, has been recently described as an additional factor contributing to vitiligo hypopigmentation80 (Fig. 3). In the treatment of vitiligo, repigmentation frequently begins in the peri-follicular area. This likely arises from the reservoir of melanocyte stem cells (MelSCs) in the hair follicle bulge81. These quiescent MelSCs reside in the lower portion of the hair follicle as undifferentiated and not characterized by specific markers, but they are defined by their cellular shape and location within the hair follicle, their low proliferative rate and their low levels of KIT and MITF expression82. Quiescence, maintenance, activation and proliferation of MelSC are mediated by specific activities in the microenvironment that can influence the differentiation and regeneration of melanocytes. In response to wouding or UV irradiation, follicular MelSC migrate towards the basal layer of the epidermis and differentiate into functional epidermis melanocytes. This migration behavior of MelSC following environmental stimuli gives the opportunity to develop therapeutic approaches for treating skin hypopigmentation disorders by manipulating this stem cell population. For istance, narrow-band UV-B exposure has been used to treat patient with vitiligo, thus leading to follicular repigmentation of depigmented skin. The mechanicistic rationale underlying the success of this therapy is related to the proliferation, migration and differentiation of MelSC83.
Nevertheless, during vitiligo treatment some areas are refractory to repigmentation such as hands and feet84. The most relevant reason seems to be the lower melanocyte density, lower melanocyte stem cells reservoirs and lower baseline levels of epidermal stem cell requiring factors85. The maturation and function of hair follicle stem cells are regulated by dermal cells, including adipocytes, dermal papilla cells and fibroblasts, and it is plausible that metabolic impairment of dermal cells will affect stem cells. Moreover, the Herlyn group demonstrated that when dermal stem cells are embedded into dermis, a subpopulation migrates to the epidermis and differentiates into melanocytes86.
Understanding the development and evolution of pigmentary system requires the knowledge of cellular interactions and signaling pathways producing this system. The colour of human skin is determined by the amount and type of melanin pigment produced by melanocytes and transferred to keratinocytes. Nevertheless, the range of phenotypic differences in pigmentary traits it is not only attributed to melanocyte cells but it certainly encompasses other skin cell populations. Changes in these interactions are likely to explain some ethnic differences and, whenever deregulated, the occurrence of pigmentary disorders characterized by either hyper- or hypo-pigmentation.
Figures and Tables
Fig. 1
Skin types pigmentation. In lightly pigmented skin, melanosomes are smaller, mainly at early stages I and II of maturation and they are transferred to the surrounding keratinocytes as clusters in membrane bound organelles. In darkly pigmented skin, melanosomes are more abundant, larger, at stage IV and are singly transferred to the neighbouring cells. Their degradation appears also slower than that observed in light skin, as melanins is yet detected in the stratum corneum. Immunohistochemical analysis of the expression of MART-1 in a skin specimen. Nuclei are counterstained with haematoxylin (×200).
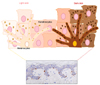
Fig. 2
Epithelial-mesenchymal melanogenic interplay and hyperpigmentary disorders: altered expression of growth factors/mediators and their receptors regulating melanocyte functionality has been shown in several hyperpigmentations, including solar/senile lentigo, melasma, dermatofibroma and seborrhoeic keratosis (H&E, ×100). KGF: keratinocyte growth factor, KGFR: keratinocyte growth factor receptor, SCF: stem cell factor, HGF: hepatocyte growth factor, ET-1: endothelin-1, α-MSH: alpha melanocyte-stimulating hormone, ETBR: endothelin B receptor, sFRP2: secreted frizzled-related protein 2, MMP2: metalloproteinase 2, VEGF: vascular endothelial growth factor.
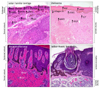
Fig. 3
Vitiligo as a model of deregulated epithelial-mesenchymal melanogenic interplay in hypopigmented disorders (H&E, ×100). KGF: keratinocyte growth factor, SCF: stem cell factor, ET-1: endothelin-1, GM-CSF: granulocyte macrophage colony-stimulating factor, TNF-α: tumor necrosis factor alpha, IL-1α: interleukin 1 alpha, bFGF: basic fibroblast growth factor, DKK1: dickkopf 1.
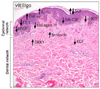
ACKNOWLEDGMENT
We thank Marco Zaccarini, Dermatopathology Unit, San Gallicano Dermatologic Institute for technical assistance.
References
1. Fitzpatrick TB, Breathnach AS. The epidermal melanin unit system. Dermatol Wochenschr. 1963; 147:481–489.
2. Yamaguchi Y, Hearing VJ. Physiological factors that regulate skin pigmentation. Biofactors. 2009; 35:193–199.


3. Staricco RJ, Pinkus H. Quantitative and qualitative data on the pigment cells of adult human epidermis. J Invest Dermatol. 1957; 28:33–45.


4. Alaluf S, Barrett K, Blount M, Carter N. Ethnic variation in tyrosinase and TYRP1 expression in photoexposed and photoprotected human skin. Pigment Cell Res. 2003; 16:35–42.


5. Alaluf S, Atkins D, Barrett K, Blount M, Carter N, Heath A. Ethnic variation in melanin content and composition in photoexposed and photoprotected human skin. Pigment Cell Res. 2002; 15:112–118.


6. Rawlings AV. Ethnic skin types: are there differences in skin structure and function? Int J Cosmet Sci. 2006; 28:79–93.


7. Kadekaro AL, Kavanagh RJ, Wakamatsu K, Ito S, Pipitone MA, Abdel-Malek ZA. Cutaneous photobiology. The melanocyte vs the sun: who will win the final round? Pigment Cell Res. 2003; 16:434–447.


8. Smith SS, Jordan WD. An essay on the causes of the variety of complexion and figure in the human species. Cambridge: Belknap Press of Harvard University Press;1965.
9. Mitchell J, Collinson P. An essay upon the causes of the different colours of people in different climates. Phil Trans. 1744; 43:102–150.
12. Sulem P, Gudbjartsson DF, Stacey SN, Helgason A, Rafnar T, Magnusson KP, et al. Genetic determinants of hair, eye and skin pigmentation in Europeans. Nat Genet. 2007; 39:1443–1452.


13. Han J, Kraft P, Nan H, Guo Q, Chen C, Qureshi A, et al. A genome-wide association study identifies novel alleles associated with hair color and skin pigmentation. PLoS Genet. 2008; 4:e1000074.


14. Rees JL. The melanocortin 1 receptor (MC1R): more than just red hair. Pigment Cell Res. 2000; 13:135–140.


15. Böhm M, Luger TA, Tobin DJ, García-Borrón JC. Melanocortin receptor ligands: new horizons for skin biology and clinical dermatology. J Invest Dermatol. 2006; 126:1966–1975.


16. Smith R, Healy E, Siddiqui S, Flanagan N, Steijlen PM, Rosdahl I, et al. Melanocortin 1 receptor variants in an Irish population. J Invest Dermatol. 1998; 111:119–122.


17. John PR, Makova K, Li WH, Jenkins T, Ramsay M. DNA polymorphism and selection at the melanocortin-1 receptor gene in normally pigmented southern African individuals. Ann N Y Acad Sci. 2003; 994:299–306.


18. Cui R, Widlund HR, Feige E, Lin JY, Wilensky DL, Igras VE, et al. Central role of p53 in the suntan response and pathologic hyperpigmentation. Cell. 2007; 128:853–864.


19. Tada A, Suzuki I, Im S, Davis MB, Cornelius J, Babcock G, et al. Endothelin-1 is a paracrine growth factor that modulates melanogenesis of human melanocytes and participates in their responses to ultraviolet radiation. Cell Growth Differ. 1998; 9:575–584.
20. Imokawa G. Autocrine and paracrine regulation of melanocytes in human skin and in pigmentary disorders. Pigment Cell Res. 2004; 17:96–110.


21. Murase D, Hachiya A, Amano Y, Ohuchi A, Kitahara T, Takema Y. The essential role of p53 in hyperpigmentation of the skin via regulation of paracrine melanogenic cytokine receptor signaling. J Biol Chem. 2009; 284:4343–4353.


22. Tomita Y, Maeda K, Tagami H. Melanocyte-stimulating properties of arachidonic acid metabolites: possible role in postinflammatory pigmentation. Pigment Cell Res. 1992; 5:357–361.


23. Kovacs D, Flori E, Maresca V, Ottaviani M, Aspite N, Dell'Anna ML, et al. The eumelanin intermediate 5,6-dihydroxyindole-2-carboxylic acid is a messenger in the cross-talk among epidermal cells. J Invest Dermatol. 2012; 132:1196–1205.


24. Candille SI, Kaelin CB, Cattanach BM, Yu B, Thompson DA, Nix MA, et al. A -defensin mutation causes black coat color in domestic dogs. Science. 2007; 318:1418–1423.


25. Swope VB, Jameson JA, McFarland KL, Supp DM, Miller WE, McGraw DW, et al. Defining MC1R regulation in human melanocytes by its agonist α-melanocortin and antagonists agouti signaling protein and β-defensin 3. J Invest Dermatol. 2012; 132:2255–2262.


26. Jarrett SG, Wolf Horrell EM, Boulanger MC, D'Orazio JA. Defining the contribution of MC1R physiological ligands to ATR phosphorylation at Ser435, a predictor of DNA repair in melanocytes. J Invest Dermatol. 2015; 135:3086–3095.


27. Hedley S, Gawkrodger DJ, Weetman AP, MacNeil S. Investigation of the influence of extracellular matrix proteins on normal human melanocyte morphology and melanogenic activity. Br J Dermatol. 1996; 135:888–897.


28. Hara M, Yaar M, Tang A, Eller MS, Reenstra W, Gilchrest BA. Role of integrins in melanocyte attachment and dendricity. J Cell Sci. 1994; 107:2739–2748.


29. Choi W, Wolber R, Gerwat W, Mann T, Batzer J, Smuda C, et al. The fibroblast-derived paracrine factor neuregulin-1 has a novel role in regulating the constitutive color and melanocyte function in human skin. J Cell Sci. 2010; 123:3102–3111.


30. Yamaguchi Y, Morita A, Maeda A, Hearing VJ. Regulation of skin pigmentation and thickness by Dickkopf 1 (DKK1). J Investig Dermatol Symp Proc. 2009; 14:73–75.


31. Cardinali G, Ceccarelli S, Kovacs D, Aspite N, Lotti LV, Torrisi MR, et al. Keratinocyte growth factor promotes melanosome transfer to keratinocytes. J Invest Dermatol. 2005; 125:1190–1199.


32. Cardinali G, Bolasco G, Aspite N, Lucania G, Lotti LV, Torrisi MR, et al. Melanosome transfer promoted by keratinocyte growth factor in light and dark skin-derived keratinocytes. J Invest Dermatol. 2008; 128:558–567.


33. Kovacs D, Cardinali G, Aspite N, Cota C, Luzi F, Bellei B, et al. Role of fibroblast-derived growth factors in regulating hyperpigmentation of solar lentigo. Br J Dermatol. 2010; 163:1020–1027.


34. Chen N, Hu Y, Li WH, Eisinger M, Seiberg M, Lin CB. The role of keratinocyte growth factor in melanogenesis: a possible mechanism for the initiation of solar lentigines. Exp Dermatol. 2010; 19:865–872.


35. Snell RS. The pigmentary changes occurring in the breast skin during pregnancy and following estrogen treatment. J Invest Dermatol. 1964; 43:181–186.


36. Wilson MJ, Spaziani E. The melanogenic response to testosterone in scrotal epidermis: effects on tyrosinase activity and protein synthesis. Acta Endocrinol (Copenh). 1976; 81:435–448.


38. Si J, Lee S, Park JM, Sung J, Ko G. Genetic associations and shared environmental effects on the skin microbiome of Korean twins. BMC Genomics. 2015; 16:992–1002.


39. Elias PM, Menon GK. Structural and lipid biochemical correlates of the epidermal permeability barrier. Adv Lipid Res. 1991; 24:1–26.


40. Guilloteau K, Paris I, Pedretti N, Boniface K, Juchaux F, Huguier V, et al. Skin inflammation induced by the synergistic action of IL-17A, IL-22, oncostatin M, IL-1{alpha}, and TNF-{alpha} recapitulates some features of psoriasis. J Immunol. 2010; 184:5263–5270.


41. Liang SC, Tan XY, Luxenberg DP, Karim R, Dunussi-Joannopoulos K, Collins M, et al. Interleukin (IL)-22 and IL-17 are coexpressed by Th17 cells and cooperatively enhance expression of antimicrobial peptides. J Exp Med. 2006; 203:2271–2279.


42. Gläser R, Navid F, Schuller W, Jantschitsch C, Harder J, Schröder JM, et al. UV-B radiation induces the expression of antimicrobial peptides in human keratinocytes in vitro and in vivo. J Allergy Clin Immunol. 2009; 123:1117–1123.


43. Elias PM, Menon G, Wetzel BK, Williams J. Barrier requirements as the evolutionary "driver" of epidermal pigmentation in humans. Am J Hum Biol. 2010; 22:526–537.


44. Jung SE, Kang HY, Lee ES, Kim YC. Changes of epidermal thickness in vitiligo. Am J Dermatopathol. 2015; 37:289–292.


45. Ganju P, Nagpal S, Mohammed MH, Nishal Kumar P, Pandey R, Natarajan VT, et al. Microbial community profiling shows dysbiosis in the lesional skin of Vitiligo subjects. Sci Rep. 2016; 6:18761.


46. Maresca V, Flori E, Camera E, Bellei B, Aspite N, Ludovici M, et al. Linking αMSH with PPARγ in B16-F10 melanoma. Pigment Cell Melanoma Res. 2013; 26:113–127.


47. Buscà R, Ballotti R. Cyclic AMP a key messenger in the regulation of skin pigmentation. Pigment Cell Res. 2000; 13:60–69.


48. Herraiz C, Journé F, Abdel-Malek Z, Ghanem G, Jiménez-Cervantes C, García-Borrón JC. Signaling from the human melanocortin 1 receptor to ERK1 and ERK2 mitogen-activated protein kinases involves transactivation of cKIT. Mol Endocrinol. 2011; 25:138–156.


49. Bellei B, Pitisci A, Izzo E, Picardo M. Inhibition of melanogenesis by the pyridinyl imidazole class of compounds: possible involvement of the Wnt/β-catenin signaling pathway. PLoS One. 2012; 7:e33021.


50. Kang HY, Chung E, Lee M, Cho Y, Kang WH. Expression and function of peroxisome proliferator-activated receptors in human melanocytes. Br J Dermatol. 2004; 150:462–468.


51. Flori E, Mastrofrancesco A, Kovacs D, Ramot Y, Briganti S, Bellei B, et al. 2,4,6-Octatrienoic acid is a novel promoter of melanogenesis and antioxidant defence in normal human melanocytes via PPAR-γ activation. Pigment Cell Melanoma Res. 2011; 24:618–630.


52. Aoki H, Moro O, Tagami H, Kishimoto J. Gene expression profiling analysis of solar lentigo in relation to immunohistochemical characteristics. Br J Dermatol. 2007; 156:1214–1223.


53. Lin CB, Hu Y, Rossetti D, Chen N, David C, Slominski A, et al. Immuno-histochemical evaluation of solar lentigines: The association of KGF/KGFR and other factors with lesion development. J Dermatol Sci. 2010; 59:91–97.


54. Salducci M, André N, Guéré C, Martin M, Fitoussi R, Vié K, et al. Factors secreted by irradiated aged fibroblasts induce solar lentigo in pigmented reconstructed epidermis. Pigment Cell Melanoma Res. 2014; 27:502–504.


55. Duval C, Cohen C, Chagnoleau C, Flouret V, Bourreau E, Bernerd F. Key regulatory role of dermal fibroblasts in pigmentation as demonstrated using a reconstructed skin model: impact of photo-aging. PLoS One. 2014; 9:e114182.


56. Hasegawa K, Fujiwara R, Sato K, Shin J, Kim SJ, Kim M, et al. Possible involvement of keratinocyte growth factor in the persistence of hyperpigmentation in both human facial solar lentigines and melasma. Ann Dermatol. 2015; 27:626–629.


57. Iriyama S, Ono T, Aoki H, Amano S. Hyperpigmentation in human solar lentigo is promoted by heparanase-induced loss of heparan sulfate chains at the dermal-epidermal junction. J Dermatol Sci. 2011; 64:223–228.


58. Miot LD, Miot HA, Polettini J, Silva MG, Marques ME. Morphologic changes and the expression of alpha-melanocyte stimulating hormone and melanocortin-1 receptor in melasma lesions: a comparative study. Am J Dermatopathol. 2010; 32:676–682.


59. Kang HY, Hwang JS, Lee JY, Ahn JH, Kim JY, Lee ES, et al. The dermal stem cell factor and c-kit are overexpressed in melasma. Br J Dermatol. 2006; 154:1094–1099.


60. Lee DJ, Park KC, Ortonne JP, Kang HY. Pendulous melanocytes: a characteristic feature of melasma and how it may occur. Br J Dermatol. 2012; 166:684–686.


61. Torres-Álvarez B, Mesa-Garza IG, Castanedo-Cázares JP, Fuentes-Ahumada C, Oros-Ovalle C, Navarrete-Solis J, et al. Histochemical and immunohistochemical study in melasma: evidence of damage in the basal membrane. Am J Dermatopathol. 2011; 33:291–295.


62. Kim EH, Kim YC, Lee ES, Kang HY. The vascular characteristics of melasma. J Dermatol Sci. 2007; 46:111–116.


63. Kim M, Han JH, Kim JH, Park TJ, Kang HY. Secreted frizzled-related protein 2 (sFRP2) functions as a melanogenic stimulator; the role of sFRP2 in UV-induced hyperpigmentary disorders. J Invest Dermatol. 2016; 136:236–244.


64. Shishido E, Kadono S, Manaka I, Kawashima M, Imokawa G. The mechanism of epidermal hyperpigmentation in dermatofibroma is associated with stem cell factor and hepatocyte growth factor expression. J Invest Dermatol. 2001; 117:627–633.


65. Okazaki M, Yoshimura K, Suzuki Y, Uchida G, Kitano Y, Harii K, et al. The mechanism of epidermal hyperpigmentation in café-au-lait macules of neurofibromatosis type 1 (von Recklinghausen's disease) may be associated with dermal fibroblast-derived stem cell factor and hepatocyte growth factor. Br J Dermatol. 2003; 148:689–697.


66. Cardinali G, Kovacs D, Giglio MD, Cota C, Aspite N, Amantea A, et al. A kindred with familial progressive hyperpigmentation-like disorder: implication of fibroblast-derived growth factors in pigmentation. Eur J Dermatol. 2009; 19:469–473.


67. Lan CC, Wu CS, Cheng CM, Yu CL, Chen GS, Yu HS. Pigmentation in basal cell carcinoma involves enhanced endothelin-1 expression. Exp Dermatol. 2005; 14:528–534.


68. Teraki E, Tajima S, Manaka I, Kawashima M, Miyagishi M, Imokawa G. Role of endothelin-1 in hyperpigmentation in seborrhoeic keratosis. Br J Dermatol. 1996; 135:918–923.


69. Picardo M, Dell'Anna ML, Ezzedine K, Hamzavi I, Harris JE, Parsad D, et al. Vitiligo. Nat Rev Dis Primers. 2015; 1:15011.


70. XXII International Pigment Cell Conference (IPCC). "Bringing Colors to Life: Advances in Pigment Cell Research and Translation into Clinical Practice" Organised by the Asian Society for Pigment Cell Research (ASPCR), in partnership with the Dermatological Society of Singapore (DSS). Pigment Cell Melanoma Res. 2014; 27:849–1001.
71. Bellei B, Pitisci A, Ottaviani M, Ludovici M, Cota C, Luzi F, et al. Vitiligo: a possible model of degenerative diseases. PLoS One. 2013; 8:e59782.


72. Kitamura R, Tsukamoto K, Harada K, Shimizu A, Shimada S, Kobayashi T, et al. Mechanisms underlying the dysfunction of melanocytes in vitiligo epidermis: role of SCF/KIT protein interactions and the downstream effector, MITF-M. J Pathol. 2004; 202:463–475.


73. Moretti S, Fabbri P, Baroni G, Berti S, Bani D, Berti E, et al. Keratinocyte dysfunction in vitiligo epidermis: cytokine microenvironment and correlation to keratinocyte apoptosis. Histol Histopathol. 2009; 24:849–857.
74. Lee AY, Kim NH, Choi WI, Youm YH. Less keratinocyte-derived factors related to more keratinocyte apoptosis in depigmented than normally pigmented suction-blistered epidermis may cause passive melanocyte death in vitiligo. J Invest Dermatol. 2005; 124:976–983.


75. Moretti S, Spallanzani A, Amato L, Hautmann G, Gallerani I, Fabiani M, et al. New insights into the pathogenesis of vitiligo: imbalance of epidermal cytokines at sites of lesions. Pigment Cell Res. 2002; 15:87–92.


76. Wagner RY, Luciani F, Cario-André M, Rubod A, Petit V, Benzekri L, et al. Altered e-cadherin levels and distribution in melanocytes precede clinical manifestations of vitiligo. J Invest Dermatol. 2015; 135:1810–1819.


77. Le Poole IC, van den Wijngaard RM, Westerhof W, Das PK. Tenascin is overexpressed in vitiligo lesional skin and inhibits melanocyte adhesion. Br J Dermatol. 1997; 137:171–178.


78. Ricard AS, Pain C, Daubos A, Ezzedine K, Lamrissi-Garcia I, Bibeyran A, et al. Study of CCN3 (NOV) and DDR1 in normal melanocytes and vitiligo skin. Exp Dermatol. 2012; 21:411–416.


79. Oh SH, Kim JY, Kim MR, Do JE, Shin JY, Hann SK. DKK1 is highly expressed in the dermis of vitiligo lesion: is there association between DKK1 and vitiligo. J Dermatol Sci. 2012; 66:163–165.


80. Purpura V, Persechino F, Belleudi F, Scrofani C, Raffa S, Persechino S, et al. Decreased expression of KGF/FGF7 and its receptor in pathological hypopigmentation. J Cell Mol Med. 2014; 18:2553–2557.


81. Nishimura EK. Melanocyte stem cells: a melanocyte reservoir in hair follicles for hair and skin pigmentation. Pigment Cell Melanoma Res. 2011; 24:401–410.


82. Ueno M, Aoto T, Mohri Y, Yokozeki H, Nishimura EK. Coupling of the radiosensitivity of melanocyte stem cells to their dormancy during the hair cycle. Pigment Cell Melanoma Res. 2014; 27:540–551.


83. Goldstein NB, Koster MI, Hoaglin LG, Spoelstra NS, Kechris KJ, Robinson SE, et al. Narrow band ultraviolet B treatment for human vitiligo is associated with proliferation, migration, and differentiation of melanocyte precursors. J Invest Dermatol. 2015; 135:2068–2076.


84. Taïeb A, Picardo M. VETF Members. The definition and assessment of vitiligo: a consensus report of the Vitiligo European Task Force. Pigment Cell Res. 2007; 20:27–35.


85. Esmat SM, El-Tawdy AM, Hafez GA, Zeid OA, Abdel Halim DM, Saleh MA, et al. Acral lesions of vitiligo: why are they resistant to photochemotherapy? J Eur Acad Dermatol Venereol. 2012; 26:1097–1104.


86. Li L, Fukunaga-Kalabis M, Yu H, Xu X, Kong J, Lee JT, et al. Human dermal stem cells differentiate into functional epidermal melanocytes. J Cell Sci. 2010; 123:853–860.

