Abstract
Background
S100A8 is differentially expressed in various cell types and is associated with a number of malignant disorders. S100A8 may affect tumor biology. However, its role in cutaneous squamous cell carcinoma (SCC) is not well established.
Objective
This study aims to investigate the relationship between S100A8 and cutaneous SCC development.
Methods
We performed immunohistochemical staining to detect S100A8 expression in facial skin specimens of premalignant actinic keratosis (AK), malignant SCC, and normal tissues. In addition, we utilized postconfluence and high calcium-induced differentiation in a culture system model. Furthermore, we constructed a recombinant adenovirus expressing GFP-tagged S100A8 to investigate the role of S100A8 in SCC cell differentiation.
Results
S100A8 was significantly overexpressed in human cutaneous SCC compared to that in normal and AK tissues. S100A8 was gradually upregulated in SCC cells in a post-confluence-induced differentiation model. Overexpression of S100A8 in SCC cells induced by adenoviral transduction led to increased expression levels of differentiation markers, such as loricrin, involucrin, and filaggrin. S100A8 overexpression also increased loricrin and involucrin luciferase activity.
Cutaneous squamous cell carcinoma (SCC) is a type of non-melanoma skin cancer derived from suprabasal epidermal keratinocytes. It is the second most common cutaneous cancer among elderly persons, following basal cell carcinoma. In most cases, it evolves from precursor lesions, such as those of actinic keratosis (AK) and Bowen's disease. AK is the initial lesion in the majority of invasive cutaneous SCC cases123.
S100 proteins are a group of cytoplasmic Ca2+-binding proteins that are differentially expressed in various cell types. They are expressed in neutrophils, monocytes, and macrophages and are released from activated phagocytes45. S100A8 (calgranulin A or migration inhibitory factor-related protein 8 is a member of the S100 protein family and preferentially forms heteromeric calprotectin with S100A9. Although S100A8 and S100A9 form heteromeric protein complexes, distinct individual S100 protein functions have also been reported6.
Recent studies have shown that S100A8 protein is associated with various malignant disorders. Genomic abnormalities at the chromosomal region 1q21, a region in which most S100 genes are clustered, were frequently observed in various epithelial cancers, including lung, breast, colorectal, and liver cancer78910. Differential S100A8 expression has also been observed in many neoplastic diseases1112131415. Moreover, in tumors of glandular cell origin, S100A8/A9 expression is associated with poor cell differentiation161718. Nevertheless, little research has been done on S100A8 expression in cutaneous SCC and its relationship with cancer cell differentiation.
In the present study, we investigated differential S100A8 expression in premalignant AK and cutaneous SCC and evaluated the putative role of S100A8 in cultured SCC cells using an adenoviral gene delivery system. We demonstrated that S100A8 regulates cutaneous SCC differentiation and induces well-differentiated SCC formation in skin by modulating differentiation-related proteins.
Surgically resected or biopsy samples were collected from 8 normal skin, 8 AK, and 24 SCC samples from diagnosed patients treated at the Department of Dermatology of Chungnam National University Hospital (Daejeon, Korea).
Immunohistochemistry using an antibody against S100A8 (Santa Cruz Biotechnologies, CA, USA; sc-8112, goat polyclonal) was performed on 4-µm paraffin-embedded tissue sections. All slides were evaluated by one dermatologist and two dermatopathologists. High-power fields (×200) were randomly selected in each specimen. One-hundred tumor cells were counted in each field, and the average percentages of cytoplasmic-positive cells for each staining from the 3 HPFs were calculated for each sample. Expression levels were classified as grade 1 (<5% of tumor cells), grade 2 (6%~25%), grade 3 (26%~50%), or grade 4 (>50%). Staining of grade 3 and above was considered positive. The positive staining intensity of S100A8 in skin samples was analyzed using an image analyzer (ScanScope® system; DakoCytomation, Carpinteria, CA, USA).
To harvest tissues for immonoblotting, tissues were lysed in Pro-Prep solution (Intron, Daejeon, Korea) and homogenized with the TissueLyser (Qiagen, Hilden, Germany). To harvest cell lysates for immunoblotting, cells were lysed in Pro-Prep solution. Total protein was measured using a BCA protein assay kit (Thermo Scientific, Rockford, IL, USA). Samples (20~30 µg protein per lane) were run on sodium dodecyl sulfate-polyacrylamide gels, transferred onto nitrocellulose membranes, and incubated with the appropriate antibodies. Blots were then incubated with peroxidase-conjugated secondary antibodies and visualized by enhanced chemiluminescence (Intron). The following primary antibodies were used for western blots: S100A8, involucrin, and actin (Santa Cruz Biotechnologies), loricrin (Covance, Princeton, NJ, USA), and filaggrin (Abcam, Cambridge, UK).
SCC12 cells were maintained in Dulbecco's Modified Eagle medium (Welgene, Daegu, Korea) supplemented with 10% fetal bovine serum (Thermo Fisher Scientific, Waltham, MA, USA).
Total RNA was isolated from cultured skin epithelial cells using the easy-BLUE™ RNA extraction kit (Intron). Two micrograms of total RNA was reverse transcribed with moloney-murine leukemia virus reverse transcriptase (ELPIS Biotech, Daejeon, Korea). Aliquots of reverse transcription mixture underwent polymerase chain reaction cycles with an S100A8 primer set. S100A8 cDNAs were subcloned into a pENTR/CMV-Flag vector with attL sites for site-specific recombination with a Gateway destination vector (Invitrogen, Carlsbad, CA, USA). Replication-incompetent adenoviruses were created using the ViraPower™ Adenoviral Expression System (Invitrogen), according to the manufacturer's instructions. Briefly, site-specific recombination between the entry vector and adenoviral destination vector was achieved using LR Clonase (Invitrogen). The resulting adenoviral expression vector was then transfected into 293A cells using Lipofectamine® 2000 (Invitrogen). Cells were grown until 80% of the cytopathic effect was seen and then harvested for recombinant adenovirus preparation.
SCC12 cells were grown at 50% confluency in 12-well culture plates and co-transduced with adenoviruses harboring an involucrin or loricrin promoter reporter cassette, in which about 3.7 kb of the involucrin promoter fragment and 2.0 kb of loricrin promoter fragment, respectively, were fused to luciferase gene and adenoviruses expressing GFP-tagged S100A8. After incubation for 6 hours, cells were replenished with fresh medium. Cells were further incubated for 3 days. Luciferase activities were determined using the Luciferase Assay System (Promega, Madison, WI, USA), according to the recommended protocol.
Each experiment was performed at least three times, with results expressed in each case as the mean±standard deviation. Significant differences were determined by Student's t-test. p-values <0.05 were considered significant. Statistical analysis was performed using software (PASW Statistics, version 18.0; IBM Corp, Armonk, NY, USA).
We performed immunohistochemical staining to detect S100A8 expression levels in facial premalignant AK, malignant SCC, and normal skin specimens. S100A8 was barely detected in normal facial skin, but S100A8 expression gradually increased in AK cells and increased further in malignant SCC cells (Table 1). As shown in Fig. 1A, S100A8 expression more significantly increased in SCC grades I and II than in grade III. Interestingly, S100A8 was most highly expressed in squamous eddies, which are well-differentiated regions in SCC tissues. The intensities of positively stained tumor cells were also quantified using an image analyzer. To confirm the S100A8 protein level in SCC, we obtained skin tissues from three patients with AK and SCC during surgery. As expected, S100A8 was weakly detected in AK tissue, and S100A8 expression significantly increased in SCC tissue compared to that in normal tissue (Fig. 1B). These results suggest that S100A8 markedly increases in SCC, especially in well-differentiated SCC.
Based on the finding that S100A8 is highly expressed in well-differentiated SCC grade I and II, especially around squamous eddies, we decided to investigate the relationship between S100A8 expression and cutaneous SCC cell differentiation (SCC12). To examine S100A8 expression during SCC12 cell differentiation, we utilized the well-established post-confluence-induced differentiation model with high calcium in a culture system model. SCC12 cell differentiation was confirmed by the increased expression levels of involucrin and loricrin, which are well-known markers of epidermal differentiation. S100A8 was not detected during the first few days, but its expression increased gradually in a differentiation-dependent manner, particularly 5 days after cell-cell contact and calcium treatment (Fig. 2). These data suggest that increased expression of S100A8 correlates with the degree of SCC12 cell differentiation, and S100A8 is expressed with increased tumor burden as cancer cells proliferate.
Because S100A8 expression was upregulated in a differentiation-dependent manner, we determined whether S100A8 modulates SCC12 cell differentiation. To investigate the effect of S100A8, we created a recombinant adenovirus expressing GFP-tagged S100A8. After adenoviral transduction into SCC12 cells, cells were cultured for 3 days, and S100A8 overexpression was detected by fluorescence imaging (Fig. 3A). To examine the effect of S100A8 on SCC12 cell differentiation, the expression levels of various differentiation markers were analyzed by western blotting. Involucrin, loricrin, and filaggrin protein levels were significantly induced by S100A8 overexpression (Fig. 3B). To confirm that S100A8 overexpression affects involucrin and loricrin gene transcription, we co-transduced SCC12 cells with S100A8 adenovirus and involucrin-luc or loricrin-luc reporter adenoviruses, in which 3.7 kb of the involucrin promoter and 2.0 kb of loricrin promoter fragments were fused to the luciferase gene, respectively. As expected, S100A8 induced involucrin and loricrin promoter activity (Fig. 3C). These results indicate that S100A8 influences SCC12 cell differentiation by increasing the expression of differentiation-related proteins, such as involucrin, loricrin, and filaggrin.
SCC is a non-melanoma skin cancer and the second most common cutaneous cancer found in elderly persons, following basal cell carcinoma. There has been a gradual increase in the non-melanoma skin cancer incidence worldwide due to ultraviolet radiation exposure, environmental carcinogens, and many other risk factors. Over one million cases are diagnosed in the United States each year, with approximately 200,000 being SCC1. Linear regression analyses have shown statistically significant increases in cutaneous SCC incidence over time for both sexes19. As its incidence increases, interest in prevention and treatment of cutaneous SCC is also increasing.
Although ~4.0% of patients with cutaneous SCC develops nodal metastases and 1.5% die of the disease, the prognoses for cutaneous SCC patients are generally much more favorable than those for patients with SCC arising from internal organs, such as the lung and colon. This is because the majority of cutaneous SCC is indolent, well-to moderately-differentiated, and has a low malignant potential202122232425. The association between differentiation-related molecules and tumor aggressiveness was investigated by Watanabe et al.26 The expression levels of cytokeratins and involucrin in well-differentiated SCCs were similar to those in normal epidermis, but the expression levels of differentiation-specific cytokeratins and involucrin were diminished in immature tumor cells in proportion to SCC malignancy. These results suggest that changes in differentiation marker expression may be a marker for invasive ability and metastatic potential of cutaneous SCC.
Recently, new light has been shed on the role of S100A8, a member of the S100 protein family, in tumor biology27. Not only is it a tumor marker, but it may also function importantly in the development of various malignancies28. Differential S100A8 expression has been shown in diverse cancer types. Marked S100A8 upregulation is found in gastric cancer11, prostate cancer12, and colorectal cancer1314, whereas S100A8 is downregulated in other cancers, such as human esophageal SCC15. Choi et al.29 investigated S100A8 and S100A9 expression in gastric adenocarcinoma and suggested that S100A8 and S100A9 are negative regulators of lymph node metastasis and can be used as biomarkers for the prediction of lymph node metastasis in gastric adenocarcinoma.
To date, few studies have reported any relationship between S100A8 and cutaneous SCC. According to a previous study, high transcription of S100 proteins was detected in a mouse model of chemically induced skin cancer30. Furthermore, for cell differentiation, one study showed that S100A8 overexpression affects the HaCat keratinocyte differentiation31. However, no studies have investigated the relationship between S100A8 and cutaneous SCC cell differentiation.
In our study, we investigated the putative role of S100A8 in cutaneous SCC pathogenesis using an adenoviral gene delivery system. In facial normal, AK, and SCC tissues, S100A8 expression increased gradually from normal tissue to malignant SCC. Among SCC tissues, S100A8 expression was markedly higher in grades I and II than in grade III, which suggest that S100A8 is expressed mainly in well-differentiated SCCs.
Expression of S100A8 was not detected endogenously in SCC12 cells, but expression was increased post-confluence, suggesting that S100A8 is related to the degree of cutaneous SCC differentiation. When S100A8 was overexpressed in SCC12 cells using our adenoviral gene delivery system, SCC12 differentiation increased, with increased levels of the differentiation markers loricrin, involucrin, and filaggrin.
In summary, we confirmed that S100A8 is significantly overexpressed in cutaneous SCC. Moreover, our findings indicate for the first time that S100A8 induces cancer cell differentiation and eventually induces formation of well-differentiated SCC in the skin. This study also indicates a potential role for S100A8 in the formation of less aggressive or lower-risk cutaneous SCC, which has substantially lower rates of recurrence and metastases than do other internal organ-derived SCCs. Further study is needed to establish a more detailed understanding of the molecular mechanism of S100A8 in cutaneous SCC pathogenesis.
Figures and Tables
Fig. 1
S100A8 is highly expressed in cutaneous squamous cell carcinoma. (A) Immunohistochemical staining of S100A8 in normal, actinic keratosis (AK), and cutaneous squamous cell carcinoma (SCC) tissues. Positive staining intensity was calculated using an image analyzer. (B) S100A8 protein was detected by western blotting analysis. Lower panel: relative protein levels, which were calibrated to an internal control (actin). Gr: grade. *p<0.05 compared to AK.
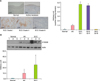
Fig. 2
Increased S100A8 expression in differentiated SCC12 cells. SCC12 cell differentiation was induced using a post-confluence-induced differentiation model or 8.0 mM calcium for 1, 3, or 5 days. S100A8 expression increased in a differentiation-dependent manner. Involucrin and loricrin were used as SCC12 differentiation markers, and actin was used as an internal control.
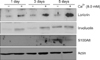
Fig. 3
Effect of S100A8 on SCC12 cell differentiation. (A) SCC12 cells were transduced with adenovirus expressing GFP-tagged S100A8 or GFP (control) and cultured for 3 days. Upper layer: bright field, lower layer: fluorescent field (×100). (B) Expression of differentiation-related proteins was assessed by western blotting analysis. S100A8 overexpression in SCC12 cells induced expression of involucrin, loricrin, and filaggrin. (C) An involucrin or loricrin promoter-luciferase reporter adenovirus was co-transduced with an adenovirus expressing GFP-tagged S100A8 or GFP (control). The cells were harvested after 3 days for a reporter assay. Involucrin and loricrin promoter activities are expressed as percentages of the control±standard deviation. *p<0.05 compared to control.
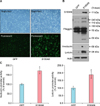
Table 1
S100A8 expression in normal, actinic keratosis, and squamous cell carcinoma facial skin specimens
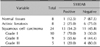
ACKNOWLEDGMENT
This research was supported by the Basic Science Research Program through the National Research Foundation of Korea (NRF) funded by the Ministry of Education, Science, and Technology (2012R1A1A1041389).
References
1. Grossman D, Leffel DJ. Squamous cell carcinoma. In : Goldsmith LA, Katz SI, Gilchrest BA, Paller AS, Leffell DJ, Wolff K, editors. Fitzpatrick's dermatology in general medicine. 8th ed. New York: McGraw-Hill;2012. p. 1283–1294.
2. Johnson TM, Rowe DE, Nelson BR, Swanson NA. Squamous cell carcinoma of the skin (excluding lip and oral mucosa). J Am Acad Dermatol. 1992; 26:467–484.


3. Feldman SR, Fleischer AB Jr. Progression of actinic keratosis to squamous cell carcinoma revisited: clinical and treatment implications. Cutis. 2011; 87:201–207.
4. Ryckman C, Vandal K, Rouleau P, Talbot M, Tessier PA. Proinflammatory activities of S100: proteins S100A8, S100A9, and S100A8/A9 induce neutrophil chemotaxis and adhesion. J Immunol. 2003; 170:3233–3242.


5. Roth J, Vogl T, Sorg C, Sunderkötter C. Phagocyte-specific S100 proteins: a novel group of proinflammatory molecules. Trends Immunol. 2003; 24:155–158.


6. Kerkhoff C, Voss A, Scholzen TE, Averill MM, Zänker KS, Bornfeldt KE. Novel insights into the role of S100A8/A9 in skin biology. Exp Dermatol. 2012; 21:822–826.


7. Petersen S, Aninat-Meyer M, Schlüns K, Gellert K, Dietel M, Petersen I. Chromosomal alterations in the clonal evolution to the metastatic stage of squamous cell carcinomas of the lung. Br J Cancer. 2000; 82:65–73.


8. Jee KJ, Gong G, Ahn SH, Park JM, Knuutila S. Gain in 1q is a common abnormality in phyllodes tumours of the breast. Anal Cell Pathol. 2003; 25:89–93.


9. Knösel T, Petersen S, Schwabe H, Schlüns K, Stein U, Schlag PM, et al. Incidence of chromosomal imbalances in advanced colorectal carcinomas and their metastases. Virchows Arch. 2002; 440:187–194.


10. Sy SM, Wong N, Lai PB, To KF, Johnson PJ. Regional over-representations on chromosomes 1q, 3q and 7q in the progression of hepatitis B virus-related hepatocellular carcinoma. Mod Pathol. 2005; 18:686–692.


11. Yong HY, Moon A. Roles of calcium-binding proteins, S100A8 and S100A9, in invasive phenotype of human gastric cancer cells. Arch Pharm Res. 2007; 30:75–81.


12. Hermani A, De Servi B, Medunjanin S, Tessier PA, Mayer D. S100A8 and S100A9 activate MAP kinase and NF-kappaB signaling pathways and trigger translocation of RAGE in human prostate cancer cells. Exp Cell Res. 2006; 312:184–197.


13. Stulík J, Koupilova K, Osterreicher J, Knízek J, Macela A, Bures J, et al. Protein abundance alterations in matched sets of macroscopically normal colon mucosa and colorectal carcinoma. Electrophoresis. 1999; 20:3638–3646.


14. Stulík J, Osterreicher J, Koupilová K, Knízek , Macela A, Bures J, et al. The analysis of S100A9 and S100A8 expression in matched sets of macroscopically normal colon mucosa and colorectal carcinoma: the S100A9 and S100A8 positive cells underlie and invade tumor mass. Electrophoresis. 1999; 20:1047–1054.


15. Luo A, Kong J, Hu G, Liew CC, Xiong M, Wang X, et al. Discovery of Ca2+-relevant and differentiation-associated genes downregulated in esophageal squamous cell carcinoma using cDNA microarray. Oncogene. 2004; 23:1291–1299.


16. Ito Y, Arai K, Nozawa R, Yoshida H, Hirokawa M, Fukushima M, et al. S100A8 and S100A9 expression is a crucial factor for dedifferentiation in thyroid carcinoma. Anticancer Res. 2009; 29:4157–4161.
17. Arai K, Takano S, Teratani T, Ito Y, Yamada T, Nozawa R. S100A8 and S100A9 overexpression is associated with poor pathological parameters in invasive ductal carcinoma of the breast. Curr Cancer Drug Targets. 2008; 8:243–252.


18. Duan L, Wu R, Ye L, Wang H, Yang X, Zhang Y, et al. S100A8 and S100A9 are associated with colorectal carcinoma progression and contribute to colorectal carcinoma cell survival and migration via Wnt/β-catenin pathway. PLoS One. 2013; 8:e62092.


19. Karia PS, Han J, Schmults CD. Cutaneous squamous cell carcinoma: estimated incidence of disease, nodal metastasis, and deaths from disease in the United States, 2012. J Am Acad Dermatol. 2013; 68:957–966.


20. Brantsch KD, Meisner C, Schönfisch B, Trilling B, Wehner-Caroli J, Röcken M, et al. Analysis of risk factors determining prognosis of cutaneous squamous-cell carcinoma: a prospective study. Lancet Oncol. 2008; 9:713–720.


21. Mourouzis C, Boynton A, Grant J, Umar T, Wilson A, Macpheson D, et al. Cutaneous head and neck SCCs and risk of nodal metastasis - UK experience. J Craniomaxillofac Surg. 2009; 37:443–447.


22. Cassarino DS, Derienzo DP, Barr RJ. Cutaneous squamous cell carcinoma: a comprehensive clinicopathologic classification--part two. J Cutan Pathol. 2006; 33:261–279.


23. Sereno M, Esteban IR, Zambrana F, Merino M, Gómez-Raposo C, López-Gómez M, et al. Squamous-cell carcinoma of the lungs: is it really so different? Crit Rev Oncol Hematol. 2012; 84:327–339.


24. Ambe P, Shadouh S, Granetzny S, Köhler L. Squamous cell carcinoma of the colon. A rare histological entity. Chirurg. 2011; 82:1116–1119.
25. Dyson T, Draganov PV. Squamous cell cancer of the rectum. World J Gastroenterol. 2009; 15:4380–4386.


26. Watanabe S, Ichikawa E, Takahashi H, Otsuka F. Changes of cytokeratin and involucrin expression in squamous cell carcinomas of the skin during progression to malignancy. Br J Dermatol. 1995; 132:730–739.


27. Ghavami S, Chitayat S, Hashemi M, Eshraghi M, Chazin WJ, Halayko AJ, et al. S100A8/A9: a Janus-faced molecule in cancer therapy and tumorgenesis. Eur J Pharmacol. 2009; 625:73–83.


28. Srikrishna G. S100A8 and S100A9: new insights into their roles in malignancy. J Innate Immun. 2012; 4:31–40.


29. Choi JH, Shin NR, Moon HJ, Kwon CH, Kim GH, Song GA, et al. Identification of S100A8 and S100A9 as negative regulators for lymph node metastasis of gastric adenocarcinoma. Histol Histopathol. 2012; 27:1439–1448.