Abstract
Background
Fractional laser resurfacing treatment has been extensively investigated and is widely used. However, the mechanism underlying its effects is poorly understood because of the ethical and cosmetic problems of obtaining skin biopsies required to study the changes after laser treatment.
Objective
To evaluate the usefulness of human skin explants for the investigation of fractional photothermolysis.
Methods
Full-thickness discarded skin was treated in 4 ways: no treatment (control), fractional carbon dioxide laser, fractional Er:YAG laser, and fractional 1,550-nm erbium-doped fiber laser. Both treated and non-treated skin samples were cultured ex vivo at the air-medium interface for 7 days. Frozen tissue was sectioned and stained with hematoxylin & eosin for histologic examination and nitro blue tetrazolium chloride for viability testing.
Results
Skin explants cultured for up to 3 days exhibited histologic changes similar to those observed in in vivo studies, including microscopic treatment zones surrounded by a thermal coagulation zone, re-epithelialization, and formation of microscopic epidermal necrotic debris. However, the explant structure lost its original form within 7 days of culture. The viability of skin explants was maintained for 3 days of culture but was also lost within 7 days.
Although fractional laser resurfacing treatment (FR) is widely used in dermatology clinics, data on the mechanisms underlying the resultant tissue changes in human skin are sparse. Investigating the molecular changes in the laser wound-healing process requires multiple serial skin biopsies to obtain tissue samples at various times. However, this has serious ethical and cosmetic problems, hindering investigations.
To overcome these problems, various skin models have been developed and used as substitutes for human skin. An in vivo animal model using pig skin has been used in some previous studies1,2, but porcine skin differs from human skin histologically and physiologically, including thickness, vascularity, and pH3. In addition, recent increases in the awareness of animal welfare and legal issues have raised the need for alternatives to animal models4. Accordingly, two-dimensional monocellular or co-culture systems are relatively inexpensive and uncomplicated methods but do not include a sufficient number of cell types and reproduce the three-dimensional histologic structure of skin. Meanwhile, reconstructed skin models have the basic three-dimensional structure of skin but do not usually include skin appendages and all of the cell types found in skin5. The skin explant model, which utilizes ex vivo skin organ culture, is designed to overcome these problems. Explants are small samples of tissue obtained during surgical procedures that are cultured with adequate media under various conditions5,6. Although tissue explants cannot completely reproduce in vivo conditions because of a lack of intact nervous and vascular tissues, they are useful nonetheless. Tumor explants have been used for anti-cancer drug screening7. Skin explants have been used to investigate normal growth and differentiation as well as study the effects of pharmacologic agents that modulate skin growth and differentiation in vivo8. Skin explants have recently been utilized in various areas in dermatologic research, including immunology, microbiology, and dermal sensation9,10,11.
Skin explants allow data from human tissue to be obtained while avoiding unnecessary and invasive skin biopsies. Therefore, they have great potential in dermatologic research, particularly for studies focusing on the extracellular matrix, the three-dimensional structure of skin, transdermal drug delivery, and interactions between different types of cutaneous cells5. Accordingly, the present study evaluated the usefulness of skin explants in the investigation of fractional photothermolysis.
Full-thickness discarded skin was obtained from four women 30~50 years old after abdominoplasty at the Asan Medical Center. Informed patient consent and institutional approval were obtained. The whole-skin samples were treated in 4 ways: no treatment (control), fractional nonablative laser treatment (NAFR) with a 1,550-nm erbium-doped fiber laser, fractional Er:YAG laser treatment (Er:YAG FR), or fractional carbon dioxide laser treatment (CO2 FR). NAFR was conducted with Fraxel SR 1500 laser (Reliant Technologies, Mountain View, CA, USA) set at 20 mJ per microscopic thermal zone (MTZ). Er:YAG FR was performed using an AVVIO laser (WONTECH Co., Ltd., Daejeon, Korea) with a pulse energy of 27 mJ per microbeam. A COFRAX laser (AMT Engineering, Seongnam, Korea) was used for CO2 FR, with a pulse energy of 91 mJ per MTZ. The energy levels were determined on the basis of normal parameters in clinical settings and the availability of previous human in vivo histologic data12,13,14,15.
The skin samples were sterilized in 70% (v/v) ethanol, and subcutaneous fat was removed. The samples were then cut into pieces using a 5-mm punch. The specimens of each treatment group were cultured in 2 ways (Fig. 1): (A) incubated in RPMI 1640 medium (Cellgro, Manassas, VA, USA) containing 10% type AB Human Serum (GemCell, West Sacramento, CA, USA), 100 U/ml penicillin, and streptomycin sulfate 100 µg/ml (H10 media); oriented with the apical epithelial surface up; and maintained at the air-medium interface (Fig. 1A)16; and (B) oriented with the apical epithelial surface up in 24-well Transwell permeable supports (6.5-mm wells with 8.0 µm pore polycarbonate membrane inserts, Transwell CLS3422; Costar/Corning Corp., Corning, NY, USA). In the method (B), agarose (3%) in RPMI 1640 medium was heated in a microwave and placed around the tissue to seal its edges as described previously17. The polarized tissue explants were cultured in the same media as the method (A) with 0.2 ml medium added in the insert and 1 ml medium/well in 24-well plates (Fig. 1B). The tissues were incubated in a 5% CO2 atmosphere at 37℃ for 7 days; the culture medium was changed every other day. On days 0, 1, 3, and day 7, tissue from each culture was frozen, sectioned, and stained with hematoxylin & eosin for histologic examination. To evaluate tissue viability, nitro blue tetrazolium chloride staining was also performed as described previously18.
The dimensions of microscopic wounds were compared with the data measured in previous human in vivo studies by using Student t-test. R version 2.15.3 (The R Foundation for Statistical Computing, Vienna, Austria) was used for all statistical analyses, and the level of significance was set at p<0.05.
The histologic appearance and structure of untreated frozen tissues were similar to those of human skin (Fig. 2, 3). Until 3 days of ex vivo culture, the epidermis of skin explants showed acceptable maintenance of intact structures. However, after 7 days of culture, the histologic features of the epidermis showed marked spongiosis-like changes and overt detachment of the spinous layer. In the dermis, there were various degrees of degeneration of collagen fibers after 0, 1, 3, and 7 days. There were no significant differences in the degenerative changes of the epidermis and dermis between the skin explants cultured by methods (A) and (B). In addition, nitro blue tetrazolium chloride staining showed that the skin explants were viable until day 3, but viability was lost after 7 days of culture (Fig. 4, 5). Skin explant viability did not differ between culture methods.
The explants that received NAFR exhibited multiple columns of denaturation in the epidermis and dermis, disruption of the dermo-epidermal junction, and subepidermal clefting within the MTZ. The stratum corneum and surrounding tissue appeared intact. The mean±standard deviation depth and width of MTZs were 611±121 and 170±26 µm, respectively, which are comparable to the results measured in a human in vivo study (both p>0.05)12. The skin explants treated with Er:YAG FR exhibited a microscopic ablation zone with a minimal coagulation zone. The depth and width of ablation zones were 71±30 and 314±47 µm, respectively, which are similar to the corresponding results provided by the manufacturer (80 and 350 µm, respectively; both p>0.05). The histologic examination of frozen tissues treated with CO2 FR also revealed MTZs surrounded by a thermal coagulation zone. The depth of ablation zones and thickness of coagulated zones were 316±43 and 55±11 µm, respectively, which approximated the expected values from a human in vivo study (286 and 44 µm, respectively; both p> 0.05)13.
Three days after NAFR, the cultured skin explants exhibited epidermal re-epithelialization and necrotic debris in the treated area reminiscent of microscopic epidermal necrotic debris (MEND) formed in vivo after NAFR. The Er:YAG FR-treated skin explants also exhibited re-epithelialization and MEND-like materials on day 3. In contrast, the re-epithelialization of CO2 FR-treated skin explants became prominent 7 days after treatment.
In all explants treated with NAFR, Er:YAG FR, or CO2 FR, the re-epithelialized epidermis was thinner than the surrounding untreated epidermis. There was also no evident invagination of epithelial cells in laser-treated zones. In addition, no complete extrusion of MEND or collagen remodeling was observed in any explant until day 7. The overall histologic features and viability of skin explants treated with each laser did not differ significantly between culture methods.
Skin explants can be maintained entirely immersed in medium for short-term culture. For long-term culture, the explant is usually exposed at the air-liquid interface. Air exposure better maintains the structural integrity of the skin and allows complete epidermal differentiation5. Direct contact with the liquid medium might also promote tissue edema and maceration, resulting in epidermal spongiosis and dermo-epidermal separation. Several techniques are used for culture at the air-liquid interface. Trowell et al. placed explants on lens paper with the epidermal side upward at the air-liquid interface; the lens paper was placed on a stainless steel grid bathed in the culture medium, and the explants were nourished by diffusion through the lens paper5. However, this method cannot fix the explant position. Meanwhile, Companjen et al.6 used a Transwell filter with a 2-mm hole into which the biopsy tissues were inserted. In this method, the explant position can be fixed, but the level of the liquid media must be carefully adjusted. Collins et al.17 cultured human cervical tissue explants in Transwell chambers in which the tissue was surrounded with 3% agarose. This helps fix the explant position and prevent direct contact between the tissue and liquid media. However, the Collins method, which was adapted for culture method (B) in the present study, did not result in better maintenance of the structural integrity of the skin. This may be due to the relatively small size of the explants exposed a larger surface area to the environment, which causes tissue damage. Thus, for longer maintenance, it may be helpful to culture larger pieces of tissue at the air-medium interface using 6-well plates with cell strainers as described previously19,20.
In the present study, 10% human serum was added to RPMI 1640 media. High concentrations of extracellular Ca2+ (e.g., 1.4 mM) are important for maintaining the structural cohesion of the tissue through stimulation of extracellular matrix production and epidermal differentiation5. Although the addition of serum to a culture medium is not recommended because of the problem of reproducibility, it might supply some growth factors along with extracellular Ca2+ and thus improve outcomes. The optimum temperature for skin explant culture is not well studied5. Although some authors recommend temperatures from 31℃~32℃, which correspond to average skin surface temperatures, 37℃ is regarded as the standard for tissue and cell culture5,21.
The CO2 FR induced noncontiguous columns of thermal injury in the dermis, resulting in the formation of MTZs surrounded by uninjured tissue. The low incidence of side effects with this treatment may be attributable to the spatial separation of areas of thermal damage characteristic of FR, resulting in rapid healing and a shorter duration of post-operative erythema. CO2 FR induces excellent skin collagen remodeling with low risks of scarring and post-inflammatory hyperpigmentation, and result in rapid patient recovery22,23. This is likely related not only to the ablation of epidermal and superficial dermal tissue and subsequent healing, but also to thermal energy diffusion, a process known as "residual thermal damage24,25." Brief exposure to high energy results in tissue ablation that is sufficiently rapid to limit extracutaneous dermal injury26. Dermal heating results in the dissolution of hydrogen bonds within the triple-helical structure of collagen fibrils and subsequent generation of a random coil configuration; this process is known as denaturation. Initial collagen shrinkage is primarily due to collagen denaturation occurring in the coagulation zone27. Once sufficient numbers of collagen fibrils are affected, immediate collagen shrinkage occurs28,29,30. This is thought to provide a more compact template for new collagen deposition in response to wound formation. This neocollagenesis is thought to partially account for long-term improvement in skin tightness and rhytides. The extent of neocollagenesis, which is indicative of clinical efficacy, is proportional to the degree of thermal damage31,32.
The precise action mechanisms of the instruments used in the present study and the laser-tissue interaction process in humans have not been sufficiently investigated, mainly because such studies require human skin biopsies, which pose cosmetic and ethical problems. Although experiments using animal skin including pig skin may produce results similar to those of human studies, not all results can be extrapolated to humans. In addition, the early molecular changes seem to be important in the development of FR effects. Therefore, the present study evaluated the usefulness of a human skin explant model. Skin explants cannot usually be maintained for a prolonged period as indicated by the degenerative histologic changes observed in this study. However, in the early stages, the skin explant model may reflect both the morphologic and functional aspects of the human in vivo reaction to FR.
In vivo human studies indicate CO2 FR produces an MTZ surrounded by a thermal coagulation zone immediately after treatment. On day 1 after treatment, re-epithelialization is completed and MEND formation is observed. After 2~5 days, the epithelial cells proliferate and invaginate into the coagulation zones. The complete extrusion of MEND and collagen remodeling cannot be observed within 7 days13,33. Er:YAG FR also produces microscopic ablation zones but much smaller coagulation zones. Re-epithelialization is completed within 12 hours. After 2 weeks, collagen remodeling can be observed34,35,36. NAFR produces denatured columns of epidermis and dermis, and disrupts the dermo-epidermal junction. However, the stratum corneum remains intact. Re-epithelialization is completed within 1 day. MEND is observed after 1 day and is nearly completely extruded by 1 week37. In the present study, cultured skin explants exhibited similar findings with respect to MTZs or microscopic ablation zones, coagulation zones, MEND formation, and re-epithelialization. However, the time required for re-epithelialization was longer and the thickness of the newly formed epidermis was thinner than those observed in vivo. In addition, MEND formation was less prominent, and there was no invagination of epithelial cells in the skin explants. Hence, these findings suggest the proliferation and differentiation of epidermal cells in skin explants may be inferior to those in vivo. In addition, fractional laser therapy induces inflammatory cell infiltration, which was not observed in the skin explants on histologic examination. However, the overall features of the wound-related changes were similar to those occurring in vivo, particularly in the early phase.
In summary, the human skin explant model described herein may be a useful method for investigating the immediate or early changes after fractional photothermolysis while avoiding the ethical problems of using human skin and differences in the responses between human and experimental animals. However, this model does not seem useful for evaluating long-term changes, because explants were not sustained for more than 3 days. In addition to the short survival of explants, the degenerative changes of dermal collagen make it difficult to observe the dermal rejuvenation process, which underlies the main effect of fractional lasers.
Figures and Tables
Fig. 1
Ex vivo organ culture of skin explants. Method (A): culture method in which the sample is incubated in medium with the apical epithelial surface up at the air-medium interface. Method (B): culture method using Transwell chambers and microporous inserts filled with 3% agarose.
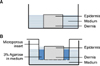
Fig. 2
Histologic appearance of human skin explants cultured using culture method (A) (H&E, ×100). NAFR: fractional nonablative laser treatment, Er:YAG FR: fractional Er:YAG laser treatment, CO2 FR: fractional carbon dioxide laser treatment.
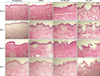
Fig. 3
Histologic appearance of human skin explants cultured using culture method (B) (H&E, ×100). NAFR: fractional nonablative laser treatment, Er:YAG FR: fractional Er:YAG laser treatment, CO2 FR: fractional carbon dioxide laser treatment.
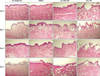
ACKNOWLEDGMENT
This research was supported by a Basic Science Research Program through the NRF of Korea (grant number: 2011-0009121) and partially supported by a grant from the Korea Health Technology R&D Project through the Korea Health Industry Development Institute (KHIDI) funded by the Ministry of Health & Welfare (grant number: HI12C1765).
References
1. Skovbølling Haak C, Illes M, Paasch U, Hædersdal M. Histological evaluation of vertical laser channels from ablative fractional resurfacing: an ex vivo pig skin model. Lasers Med Sci. 2011; 26:465–471.


2. Walgrave S, Zelickson B, Childs J, Altshuler G, Erofeev A, Yaroslavsky I, et al. Pilot investigation of the correlation between histological and clinical effects of infrared fractional resurfacing lasers. Dermatol Surg. 2008; 34:1443–1453.


3. Swindle MM. Porcine integumentary system models: Part 1-Dermal toxicology [Internet]. Columbia: Sinclair Research Center Inc.;2008. updated 2008 Dec 16. cited 2013 Sep 25. Available at: http://www.sinclairbioresources.com/Downloads/TechnicalBulletins/Porcine%20Integumentary%20System%20Model-Part%201.pdf
.
4. Lee GH, Choe BI, Kim JS, Hart LA, Han JS. The current status of animal use and alternatives in Korean veterinary medical schools. Altern Lab Anim. 2010; 38:221–230.


5. Lebonvallet N, Jeanmaire C, Danoux L, Sibille P, Pauly G, Misery L. The evolution and use of skin explants: potential and limitations for dermatological research. Eur J Dermatol. 2010; 20:671–684.
6. Companjen AR, van der Wel LI, Wei L, Laman JD, Prens EP. A modified ex vivo skin organ culture system for functional studies. Arch Dermatol Res. 2001; 293:184–190.


7. Joshi K, Demir H, Yamada R, Miyazaki T, Ray-Chaudhury A, Nakano I. Method for novel anti-cancer drug development using tumor explants of surgical specimens. J Vis Exp. 2011; (53):


8. Varani J, Fligiel SE, Schuger L, Perone P, Inman D, Griffiths CE, et al. Effects of all-trans retinoic acid and Ca++ on human skin in organ culture. Am J Pathol. 1993; 142:189–198.
9. Eaton LH, Chularojanamontri L, Ali FR, Theodorakopoulou E, Dearman RJ, Kimber I, et al. Guttate psoriasis is associated with an intermediate phenotype of impaired Langerhans cell migration. Br J Dermatol. 2014; 171:409–411.


10. Lebonvallet N, Pennec JP, Le Gall-Ianotto C, Chéret J, Jeanmaire C, Carré JL, et al. Activation of primary sensory neurons by the topical application of capsaicin on the epidermis of a re-innervated organotypic human skin model. Exp Dermatol. 2014; 23:73–75.


11. Phillips PL, Yang Q, Schultz GS. The effect of negative pressure wound therapy with periodic instillation using antimicrobial solutions on Pseudomonas aeruginosa biofilm on porcine skin explants. Int Wound J. 2013; 10:Suppl 1. 48–55.


12. Bedi VP, Chan KF, Sink RK, Hantash BM, Herron GS, Rahman Z, et al. The effects of pulse energy variations on the dimensions of microscopic thermal treatment zones in nonablative fractional resurfacing. Lasers Surg Med. 2007; 39:145–155.


13. Hantash BM, Bedi VP, Kapadia B, Rahman Z, Jiang K, Tanner H, et al. In vivo histological evaluation of a novel ablative fractional resurfacing device. Lasers Surg Med. 2007; 39:96–107.


14. Jung KE, Jung KH, Park YM, Lee JY, Kim TY, Kim HO, et al. A split-face comparison of ablative fractional lasers (CO(2) and Er:YAG) in Asian patients; postprocedure erythema, pain and patient's satisfaction. J Cosmet Laser Ther. 2013; 15:70–73.


15. Lee HM, Haw S, Kim JE, Won CH, Lee MW, Choi JH, et al. A fractional 2940 nm short-pulsed, erbium-doped yttrium aluminium garnet laser is effective and minimally invasive for the treatment of photodamaged skin in Asians. J Cosmet Laser Ther. 2012; 14:253–259.


16. Foitzik K, Paus R, Doetschman T, Dotto GP. The TGF-beta2 isoform is both a required and sufficient inducer of murine hair follicle morphogenesis. Dev Biol. 1999; 212:278–289.


17. Collins KB, Patterson BK, Naus GJ, Landers DV, Gupta P. Development of an in vitro organ culture model to study transmission of HIV-1 in the female genital tract. Nat Med. 2000; 6:475–479.


18. Neumann RA, Knobler RM, Pieczkowski F, Gebhart W. Enzyme histochemical analysis of cell viability after argon laser-induced coagulation necrosis of the skin. J Am Acad Dermatol. 1991; 25:991–998.


19. Steinstraesser L, Rittig A, Gevers K, Sorkin M, Hirsch T, Kesting M, et al. A human full-skin culture system for interventional studies. Eplasty. 2009; 9:e5.
20. Xu W, Jong Hong S, Jia S, Zhao Y, Galiano RD, Mustoe TA. Application of a partial-thickness human ex vivo skin culture model in cutaneous wound healing study. Lab Invest. 2012; 92:584–599.


21. Helbig D, Moebius A, Simon JC, Paasch U. Nonablative skin rejuvenation devices and the role of heat shock protein 70: results of a human skin explant model. J Biomed Opt. 2010; 15:038002.


22. Alster TS, West TB. Resurfacing of atrophic facial acne scars with a high-energy, pulsed carbon dioxide laser. Dermatol Surg. 1996; 22:151–154. discussion 154-155.


23. Bernstein LJ, Kauvar AN, Grossman MC, Geronemus RG. Scar resurfacing with high-energy, short-pulsed and flashscanning carbon dioxide lasers. Dermatol Surg. 1998; 24:101–107.


24. Cotton J, Hood AF, Gonin R, Beesen WH, Hanke CW. Histologic evaluation of preauricular and postauricular human skin after high-energy, short-pulse carbon dioxide laser. Arch Dermatol. 1996; 132:425–428.


25. Kauvar AN, Waldorf HA, Geronemus RG. A histopathological comparison of "char-free" carbon dioxide lasers. Dermatol Surg. 1996; 22:343–348.


26. Nanni CA, Alster TS. Complications of carbon dioxide laser resurfacing. An evaluation of 500 patients. Dermatol Surg. 1998; 24:315–320.


27. Ross EV, McKinlay JR, Anderson RR. Why does carbon dioxide resurfacing work? A review. Arch Dermatol. 1999; 135:444–454.
28. Nagy IZ, Tóth VN, Verzár F. High-resolution electron microscopy of thermal collagen denaturation in tail tendons of young, adult and old rats. Connect Tissue Res. 1974; 2:265–272.


29. Ross EV, Naseef GS, McKinlay JR, Barnette DJ, Skrobal M, Grevelink J, et al. Comparison of carbon dioxide laser, erbium:YAG laser, dermabrasion, and dermatome: a study of thermal damage, wound contraction, and wound healing in a live pig model: implications for skin resurfacing. J Am Acad Dermatol. 2000; 42:92–105.


30. Verzár F, Nagy IZ. Electronmicroscopic analysis of thermal collagen denaturation in rat tail tendons. Gerontologia. 1970; 16:77–82.


32. Tanzi EL, Alster TS. Single-pass carbon dioxide versus multiple-pass Er:YAG laser skin resurfacing: a comparison of postoperative wound healing and side-effect rates. Dermatol Surg. 2003; 29:80–84.


33. Xu XG, Luo YJ, Wu Y, Chen JZ, Xu TH, Gao XH, et al. Immunohistological evaluation of skin responses after treatment using a fractional ultrapulse carbon dioxide laser on back skin. Dermatol Surg. 2011; 37:1141–1149.


34. Dierickx CC, Khatri KA, Tannous ZS, Childs JJ, Cohen RH, Erofeev A, et al. Micro-fractional ablative skin resurfacing with two novel erbium laser systems. Lasers Surg Med. 2008; 40:113–123.


35. Kist DA, Elm CM, Eleftheriou LI, Studer JA, Wallander ID, Walgrave SE, et al. Histologic analysis of a 2,940 nm fractional device. Lasers Surg Med. 2011; 43:79–91.