Abstract
Background
Reconstructed human epidermal culture skin models have been developed for cosmetic and pharmaceutical research.
Objective
This study evaluated the total and carboxyl esterase activities (i.e., Km and Vmax, respectively) and localization in two reconstructed human epidermal culture skin models (LabCyte EPI-MODEL [Japan Tissue Engineering] and EpiDerm [MatTek/Kurabo]). The usefulness of the reconstruction cultured epidermis was also verified by comparison with human and rat epidermis.
Methods
Homogenized epidermal samples were fractioned by centrifugation. p-nitrophenyl acetate and 4-methylumbelliferyl acetate were used as substrates of total esterase and carboxyl esterase, respectively.
Esterases hydrolyze ester compounds and are classified as carboxyl, aryl, or acetylcholine esterases. These enzymes are present in various tissues and organs including the mammalian liver, skin, and plasma1,2. In the skin, esterases have particularly high activity in the epidermis and hair follicles3, with carboxyl and aryl esterases having been discovered in the cytosol and endoplasmic reticulum in the skin4,5,6,7,8. These intracellular esterases are important for metabolism9,10,11 and/or pro-drug activation12. This information has led to the development of cosmetics13 and transdermal drugs14 that include ester compounds, such as vitamin C and/or steroid derivatives. The application of ester compounds to the skin surface enhances their partition by increasing their lipophilic properties15. Metabolism experiments involving ester compounds have been performed using skin from rats, mini-pigs, and humans16. As an alternative to animal testing, in vitro skin irritation studies have been performed using reconstructed human epidermal culture skin models. However, the efficacy of these substitutes in such studies has not been evaluated. Bätz et al.17 used fluorescein diacetate as a substrate for esterase activity in the reconstructed human skin model, but there is no report about the intracellular localization of esterase in reconstructed human skin.
Therefore, this study measured the intracellular localizations of various esterases and their activities in hairless rat and human epidermis as well as reconstructed human epidermal culture skin models. Finally, the utility of reconstructed human cultured epidermis was assessed.
p-Nitrophenyl acetate (PNPA), 4-methylumbelliferyl acetate (MUA), and paraoxon were purchased from Sigma Aldrich (St. Louis, MO, USA).
A summary of the skin samples and used in this study manuscript areis shown in Table 1. Hairless rats were purchased from Saitama Experimental Animal Supply Co., Ltd. (Saitama, Japan). They were animals were housed under a 12/12-h light/and dark cycle in a temperature-controlled room (25℃). They had free access to food and water. All animal procedures were approved by the Ethics Committee of Josai University (Sakado, Saitama, Japan) in accordance with the National Institute of Health (Tokyo, Japan). The two reconstructed human epidermal culture skin models were used: LabCyte EPI-MODEL were purchased from (Japan Tissue Engineering, Gamagori, Japan) and EpiDerm (EPI-200) obtained from MatTek/Kurabo (Ashland, MA, USA), respectively. Human skin (TRANSKIN) was obtained from Biopredic International (Rennes, France) through KAC (Kyoto, Japan). The excised human skin was approved by the KAC Ethics Committee for human-derived products. This was obtained from Biopredic International. Hairless rat and human full-thickness epidermis were heated at 60℃ for 90 s, and the epidermis was carefully removed.
Epidermis samples from hairless rats and humans as well as LabCyte EPI-MODEL and EpiDerm were minced with scissors on ice. Epidermis samples were homogenized using a Teflon homogenizer (1,000 rpm, 3 strokes) in 0.25 M sucrose solution. Homogenates were centrifuged at 600g for 10 minutes, and the supernatant was collected as the post-nuclear fraction. The precipitant was dispersed using polytron homogenizer (25,000 rpm, 1 min). The supernatant was collected as the non-destruction nuclear fraction. The post-nuclear fraction was centrifuged at 3,300g for 10 minutes, and the supernatant was centrifuged again at 12,500g for 20 minutes. These precipitates were the mitochondria and light mitochondria fractions. Finally, the supernatant was ultracentrifuged at 105,000g for 70 minutes to obtain the microsomal and cytosolic fractions. All fractionation procedures were performed at 4℃.
Each fraction was mixed with 100 mM Tris-HCl buffer (pH 7.4) on a 96-well plate and was pre-incubated at 37℃. Various concentrations of PNPA solution were added, and concentrations of p-nitrophenol (PNP) were measured by a microplate reader (Spectra Max M5e; Molecular devices LLC, Sunnyvale, CA, USA) for 10 minutes at 1-minute intervals at 37℃). The enzymatic parameters (i.e., Km and Vmax) of the microsomal and cytosolic fractions were determined from the reaction rates according to the nonlinear least square method using the values calculated from the Hanes-Woolf plot. Protein levels were measured by the Lowry method18.
Paraoxon and MUA were used as substrates for aryl and carboxyl esterase, respectively. The 4-methylumbellifer-one and PNP generated by enzyme reactions were measured over time. The samples used were hairless rat abdominal epidermis, human abdominal epidermis, LabCyte EPI-MODEL, and EpiDerm.
Hairless rat epidermis was separated by the heat separation method. The influence of heat separation on the esterase activity of hairless rat epidermis is shown in Fig. 1. The protein contents of the samples are shown in Table 2. Heat treatment did not influence esterase activity or protein content.
The esterase activities and protein contents of hairless rat and human abdominal epidermis as well as LabCyte EPI-MODEL and EpiDerm were evaluated. The esterase activity patterns are shown in Fig. 2. Total esterase activity was present in the cytosolic fraction of all epidermis samples. The specific activity in the microsomal and cytosolic fractions was subsequently compared. The total esterase activities of the microsomal and cytosolic fractions in various skin samples are shown in Fig. 3. The specific esterase activities in the microsomal and cytosolic fraction were highest in the rat epidermis, followed by the EpiDerm, LabCyte EPI-MODEL, and human skin samples. The enzyme parameters Km and Vmax were also calculated (Table 3). The Km values in the microsomal and cytosolic fractions were highest in human epidermis.
Carboxyl and aryl esterase activities were measured. The specific activities of carboxyl esterase in the microsomal and cytosolic fractions were measured in the epidermis samples and models (Fig. 4). Carboxyl esterase activity was higher in the cytosolic fraction than the microsomal fraction, similar to the trend observed for total esterase activity. Meanwhile, aryl esterase activity was not detected using this method. The Km and Vmax values of the microsomal and cytosolic fractions were highest in rat epidermis and approximately equal in human epidermis, LabCyte EPI-MODEL, and EpiDerm (Table 4).
In this study, total, carboxyl, and aryl esterase activities and their intracellular localizations were evaluated in two reconstructed human epidermal culture skin models as well as human and rat epidermis.
Heat treatment was used to separate the epidermis and dermis19. Besides heat treatment, EDTA treatment20 and trypsin treatments21 have also been used for the same purpose. We used the heat separation method because of its speed. Epidermal esterase activity was first assessed after heat separation. Esterase activity and protein content did not change as a result of heat separation at 60℃ for 90 s (Fig. 1).
Total esterase in the epidermis and dermis was subsequently examined (Fig. 2). The specific activity of total esterase was highest in the microsomal and cytosolic fractions (Fig. 2). In addition, enzyme parameters were calculated. The Km values in the microsomal and cytosolic fractions of human epidermis were highest. Jewell et al.2 report that esterase activity in rat or human full-thickness skin is higher in the cytosolic fraction. In the present study, the epidermis and dermis were separated by heat, and the localization of esterase in these tissues was examined.
Esterase specific activity was higher in the epidermis than the dermis (data not shown), which is consistent with the results of McCracken et al.5,6. The esterase activity in the epidermis was higher in the cytosolic fraction than the microsomal fraction in all samples. This suggests the localizations of esterase in LabCyte EPI-MODEL, EpiDerm, and rat epidermis are similar to that in human skin. Esterase activities in LabCyte EPI-MODEL and EpiDerm were greater in the cytosolic fraction than the microsomal fraction, and the esterase-specific activities in LabCyte EPI-MODEL and EpiDerm were closer to that in human epidermis than rat epidermis. Enzyme affinities in the microsomal and cytosolic fractions of LabCyte EPI-MODEL and EpiDerm were higher than those in human epidermis. The Km and Vmax values of total esterase did not differ among the microsomal and cytosolic factions of LabCyte EPI-MODEL and EpiDerm, while those of carboxyl esterase were similar. In addition, the Km and Vmax values of total and carboxyl esterase from human skin were higher than those in the reconstructed human epidermal culture skin models. As the reconstructed human epidermal culture skin models comprise reconstituted human keratinocytes, the Km values were predicted to be similar to those in human skin; however, the Km values differed between them. This is thought to be because the human skin samples have great inter-individual variability and were freeze-thawed. Taking these factors into consideration, the affinity of the esterases of reconstructed human epidermal culture skin models and human epidermis may in fact be the same.
Like total esterase activity, there was high carboxyl esterase activity in the cytosolic fractions of all samples. Moreover, there were no differences in enzyme activities between rat epidermis and reconstructed human epidermal culture skin models. The carboxyl esterase-specific activity in the microsomal and cytosolic fractions did not differ markedly with respect to total esterase activity among rat epidermis, LabCyte EPI-MODEL, and EpiDerm. Male rat epidermis, female human epidermis, and reconstructed human epidermal cultures from male epidermis were used in the present study. Gupta and Gupta report the serum metabolic activity of aspirin esterase is higher in males than females22. Moreover, Rainsford et al.23 report that the reason for the sex difference in esterase activity is unknown; furthermore, there are no studies on the differences in esterase activities between male and female epidermis. Therefore, sex differences were not considered in the present study.
In conclusion, LabCyte EPI-MODEL and EpiDerm are potentially useful for predicting esterase activity in human epidermis.
Figures and Tables
Fig. 1
Effects of heat separation of rat epidermis and dermis on esterase activity. Left bar: intracellular localization of esterase activity in the whole epidermis without heat separation. Right bar: intracellular localization of esterase activity in the epidermis and dermis after heat separation. The esterase activities in epidermis and dermis are also shown. Data are mean±standard deviation (n=3).
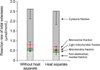
Fig. 2
Total esterase activities of various skin samples. Mitochondria (M), light mitochondria (L), microsomal (P) and cytosolic (S) fractions. (A) Rat epidermis, (B) human epidermis, (C) LabCyte EPI-MODEL, (D) EpiDerm.
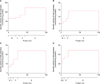
Fig. 3
Total esterase activities in the microsomal and cytosolic fractions of various skin samples. Data are mean±standard deviation (n=3).
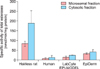
Fig. 4
Carboxyl and aryl esterase activities in the microsomal and cytosolic fractions of various skin samples. (A) Carboxyl esterase, (B) aryl esterase. Data are mean±standard deviation (n=3). ND: not detected.
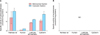
Table 1
Details of hairless rat skin, human skin and reconstructed human epidermal culture skin model used in this study

References
1. Satoh T, Hosokawa M. The mammalian carboxylesterases: from molecules to functions. Annu Rev Pharmacol Toxicol. 1998; 38:257–288.


2. Jewell C, Ackermann C, Payne NA, Fate G, Voorman R, Williams FM. Specificity of procaine and ester hydrolysis by human, minipig, and rat skin and liver. Drug Metab Dispos. 2007; 35:2015–2022.


3. Müller B, Kasper M, Surber C, Imanidis G. Permeation, metabolism and site of action concentration of nicotinic acid derivatives in human skin. Correlation with topical pharmacological effect. Eur J Pharm Sci. 2003; 20:181–195.


4. Winkelmann RK, Schmit RW. Cholinesterase in the skin of the rat, dog, cat, guinea pig and rabbit. J Invest Dermatol. 1959; 33:185–190.
5. McCracken NW, Blain PG, Williams FM. Nature and role of xenobiotic metabolizing esterases in rat liver, lung, skin and blood. Biochem Pharmacol. 1993; 45:31–36.


6. McCracken NW, Blain PG, Williams FM. Human xenobiotic metabolizing esterases in liver and blood. Biochem Pharmacol. 1993; 46:1125–1129.


7. Clark NW, Scott RC, Blain PG, Williams FM. Fate of fluazifop butyl in rat and human skin in vitro. Arch Toxicol. 1993; 67:44–48.


8. Mutch E, Nave R, McCracken N, Zech K, Williams FM. The role of esterases in the metabolism of ciclesonide to desisobutyryl-ciclesonide in human tissue. Biochem Pharmacol. 2007; 73:1657–1664.


9. Dean RA, Christian CD, Sample RH, Bosron WF. Human liver cocaine esterases: ethanol-mediated formation of ethylcocaine. FASEB J. 1991; 5:2735–2739.


10. Zhang J, Burnell JC, Dumaual N, Bosron WF. Binding and hydrolysis of meperidine by human liver carboxylesterase hCE-1. J Pharmacol Exp Ther. 1999; 290:314–318.
11. Alexson SE, Diczfalusy M, Halldin M, Swedmark S. Involvement of liver carboxylesterases in the in vitro metabolism of lidocaine. Drug Metab Dispos. 2002; 30:643–647.


12. Ettmayer P, Amidon GL, Clement B, Testa B. Lessons learned from marketed and investigational prodrugs. J Med Chem. 2004; 47:2393–2404.


13. Boehnlein J, Sakr A, Lichtin JL, Bronaugh RL. Characterization of esterase and alcohol dehydrogenase activity in skin. Metabolism of retinyl palmitate to retinol (vitamin A) during percutaneous absorption. Pharm Res. 1994; 11:1155–1159.
14. Crespi HG. Topical corticosteroid therapy for children: alclometasone dipropionate cream 0.05%. Clin Ther. 1986; 8:203–210.
15. Van Gelder J, Shafiee M, De Clercq E, Penninckx F, Van den Mooter G, Kinget R, et al. Species-dependent and site-specific intestinal metabolism of ester prodrugs. Int J Pharm. 2000; 205:93–100.


16. Prusakiewicz JJ, Ackermann C, Voorman R. Comparison of skin esterase activities from different species. Pharm Res. 2006; 23:1517–1524.


17. Bätz FM, Klipper W, Korting HC, Henkler F, Landsiedel R, Luch A, et al. Esterase activity in excised and reconstructed human skin--biotransformation of prednicarbate and the model dye fluorescein diacetate. Eur J Pharm Biopharm. 2013; 84:374–385.


18. Lowry OH, Rosebrough NJ, Farr AL, Randall RJ. Protein measurement with the Folin phenol reagent. J Biol Chem. 1951; 193:265–275.


19. Prausnitz MR, Bose VG, Langer R, Weaver JC. Electroporation of mammalian skin: a mechanism to enhance transdermal drug delivery. Proc Natl Acad Sci U S A. 1993; 90:10504–10508.


20. Bhatt RH, Micali G, Galinkin J, Palicharla P, Koch RL, West DP, et al. Determination and correlation of in vitro viability for hairless mouse and human neonatal whole skin and stratum corneum/epidermis. Arch Dermatol Res. 1997; 289:170–173.


21. Obata Y, Utsumi S, Watanabe H, Suda M, Tokudome Y, Otsuka M, et al. Infrared spectroscopic study of lipid interaction in stratum corneum treated with transdermal absorption enhancers. Int J Pharm. 2010; 389:18–23.

