Abstract
Background
We developed an ethanol extract of peanut sprouts (EPS), a peanut sprout-derived natural product, which contains a high level of trans-resveratrol (176.75 µg/ml) and was shown to have potent antioxidant activity.
Objective
We evaluated the potential anti-inflammatory activity of EPS by measuring its antioxidant potential in skin.
Methods
The anti-inflammatory activity of EPS was tested using two models of skin inflammation: oxazolone (OX)-induced contact dermatitis in mice and compound 48/80-treated HaCaT cells. As biomarkers of skin inflammation, cyclooxygenase-2 (COX-2) and nerve growth factor (NGF) levels were measured.
Results
OX-induced contact dermatitis was suppressed markedly in mice that were treated with an ointment containing 5% EPS as evidenced by a decrease in the extent of scaling and thickening (p<0.05) and supported by a histological study. COX-2 (messenger RNA [mRNA] and protein) and NGF (mRNA) levels, which were upregulated in the skin of OX-treated mice, were suppressed markedly in the skin of OX+EPS-treated mice. Consistent with this, compound 48/80-induced expression of COX-2 (mRNA and protein) and NGF (mRNA) in HaCaT cells were suppressed by EPS treatment in a dose-dependent manner. As an inhibitor of NF-κB, IκB protein levels were dose-dependently upregulated by EPS. Fluorescence-activated cell sorting (FACS) analysis revealed that EPS scavenged compound 48/80-induced reactive oxygen species (ROS) in HaCaT cells.
Peanuts contain bioactive compounds with antioxidant activity, such as resveratrol, flavonoids, phenolic compounds, and phytosterols. Peanut sprouts have been reported to contain a high concentration of trans-resveratrol, which is known to have a variety of beneficial roles including anti-inflammatory, anti-aging, anti-cancer, and cardiovascular-protective effects1. In our previous paper, we demonstrated that an ethanol extract of peanut sprout (EPS) contained 54.2 µg/ml of transresveratrol and protected cells from ultraviolet B-induced oxidative stress2. EPS had potent anti-oxidant activity and scavenged reactive oxygen species (ROS) and activated the Nrf2/anti-oxidant system2. For this study, we prepared a more concentrated EPS of higher trans-resveratrol content (176.75 µg/ml) with the expectation that this would possess a more potent anti-oxidant activity.
Steroids are widely used as anti-inflammatory agents for the treatment of acute and chronic eczema. However, long-term steroid administration inevitably leads to various side effects. Oxidative stress is known to be induced by excess production of ROS and/or insufficient scavenging of ROS, triggering downstream signaling cascades of inflammatory cytokines3. ROS could therefore act as secondary messengers in the induction of inflammation. Thus, anti-oxidants may act as anti-inflammatory agents by scavenging ROS. For these reasons, the development of non-steroidal anti-inflammatory drugs based on anti-oxidants is a promising approach in the dermatology field.
Compound 48/80, first described as a mast cell secretagogue, is a polymer derived from N-methyl-p-methoxyphenethylamine, which activates phospholipase C in mast cells via hetero-trimeric guanosine triphosphate (GTP)-binding proteins4,5. In addition to its role as a mast cell degranulator, compound 48/80 mediates inflammation, proliferation, and differentiation. Based on a previous report that compound 48/80 produced ROS in mast cells6, we tested whether compound 48/80 could induce ROS production in HaCaT cells. If so, we hypothesized that EPS could block compound 48/80-mediated ROS production as well as downstream events in skin inflammation.
Chronic eczemas are characterized by recurrent attacks of skin inflammation accompanied by severe pruritus. Skin damage due to scratching facilitates the release of neurotrophins (NTs) and/or neuropeptides (NPs) from free nerve endings in the skin, which leads to or aggravates skin inflammation7. Thus, NT- and NP-mediated neurogenic inflammation has the potential to stimulate peripheral nerves to produce not only an itching sensation, but also localized disturbances in sweating, thermoregulation, and vascular regulation8. As keratinocytes (KCs)-derived nerve growth factor (NGF) is known to be a common NT, it has been used as a biomarker for evaluating the severity of atopic dermatitis (AD)9. Thus, we investigated the levels NGF and cyclooxygenase-2 (COX-2) in skin inflammation of epidermal KCs10,11,12.
In this study, we evaluated the anti-inflammatory activity of EPS in vivo and in vitro using an oxazolone (OX)-induced contact dermatitis mouse model and compound 48/80-treated HaCaT cells, respectively.
An EPS stock solution was prepared as described previously2. Briefly, peanut sprouts were steamed and cooled twice, followed by vacuum concentration to obtain high a product with a high resveratrol content. The EPS stock solution was found to contain trans-resveratrol at a concentration of 176.75±3.63 µg/ml.
HaCaT cells (Lot-no.: 300493-Pr35) were purchased from CLS Cell Lines Service (Eppelheim, Germany). HaCaT cells were cultured in Dulbecco's modified Eagle's medium supplemented with 10% fetal bovine serum and antibiotics.
Cell viability was assayed using the colorimetric 3-[4,5-dimethylthiazol-2-yl]-2,5-diphenyltetrazoliumbromide (MTT) kit (Chemicon International Inc., Billerica, MA, USA) according to the manufacturer's instructions.
Animal experiments were approved by the Animal Care and Use Committee of the Chonnam National University Hospital, Gwangju, Korea (IACUC number: CNU IACUCH-2011-17).
Induction of allergic-type contact dermatitis for use as an animal model of skin inflammation was performed as described before with a minor modification13. SKH1 mice (Charles River Laboratory, Boston, MA, USA) were purchased from Orient Bio (Seongnam, Korea). The mice (female, 8 weeks old) were fed with normal food and tap water, and raised in the Individually Ventilated Cage Rack System (Tecniplast, Casale Litta, Italy). Briefly, contact dermatitis was induced by sensitization with 5% OX three times in the first week, followed by administration of 0.5% OX three times per week on an every-other-day schedule for the following 3 weeks (total, 9 administrations). From the third week, 5% EPS ointment was applied to the dorsal skin of the mice three times a week for 2 weeks. For control mice, petrolatum ointment base was applied instead of 5% EPS ointment on the same schedule. Mice were killed by cervical dislocation to obtain skin samples.
To semi-quantify the degree of skin inflammation, we measured whole skin thickness with a dial thickness gauge (Peacock, Scotts Valley, CA, USA). After the hairless mice were sacrificed by cervical dislocation, a skin flap containing the epidermis and dermis was immediately obtained by removing the skin layer from the underlying muscle with iris scissors.
To evaluate the degree of skin inflammation, we arbitrarily graded skin inflammation with the three criteria of scaling, edema, and thickening (lichenification). Each was graded as 0 (none), 1 (mild), 2 (moderate), and 3 (severe).
Fluorogenic 2',7'-dichlorodihydrofluorescein diacetate (DCF-DA; Invitrogen, Carlsbad, CA, USA) reacts with intracellular ROS, and can be detected by fluorescence-activated cell sorting (FACS) analysis. Briefly, HaCaT cells were seeded in each well of a 60 mm culture dish at a density of 5×105 cells per well and pretreated with EPS for 1 h. The cells were then treated with compound 48/80 (10 µg/ml) for 6 h. For FACS analysis, the cells were incubated with the redox-sensitive indicator DCF-DA (10 µM) in a 37℃ incubator for 30 min and intracellular ROS production was analyzed using flow cytometry (FACSCalibur; Becton Dickinson, San Jose, CA, USA) with an excitation wavelength of 488 nm and an emission wavelength of 530 nm. Data analyses were based on 10,000 detection events using the Cell Quest software.
A polymerase chain reaction (PCR) pre-mixture kit (ELPIS, Daejeon, Korea) was used for reverse transcriptase-PCR (RT-PCR). PCR reactions were performed with the following primers: human COX-2 (forward: 5'-cattctttgcccagcacttcac-3', reverse: 5'-gaccaggcaccagaccaaagac-3'), human NGF (forward: 5'-catacaggcggaaccacact-3', reverse: 5'-gaattcgcccctgtggaaga-3'), human glyceraldehyde 3-phosphate dehydrogenase (GAPDH; forward: 5'-gtcttcaccaccatggagaaggc-3', reverse: 5'-cggaaggccatgccagtgagctt-3'); mouse COX-2 (forward: 5'-agcagatgactgcccaactc-3', reverse: 5'-gaacccaggtcctcgcttat-3'), mouse NGF (forward: 5'-catacaggcggaaccacact-3', reverse: 5'-gaattcgcccctgtggaaga-3'), and mouse β-actin (forward: 5'-tcatgaagtgagacgttgacatccgt-3', reverse: 5'cctagaagcacttgcggtgcacgatg-3'). Annealing temperatures were 55℃ for human COX-2 and mouse β-actin, 60℃ for human GAPDH, and 61℃ for human NGF, mouse NGF and mouse COX-2. PCR products were analyzed using 1.5% agarose gel electrophoresis, stained with SYBR Safe DNA gel stain buffer (Invitrogen), and visualized using luminescence (LAS 3000; Fujifilm, Tokyo, Japan). Expression levels were normalized to endogenous β-actin and GAPDH as controls.
Whole cell and nuclear extracts were prepared as described previously2. Protein bands were probed with the following primary antibodies: anti-IκBα (#9242, 1 : 1,000; Cell Signaling Technology, Boston, MA, USA), anti-COX-2 (ab15191, 1 : 200; Abcam, Cambridge, MA, USA), and anti-β-actin (ab-6276, 1 : 1,000; Abcam). horseradish peroxidase (HRP)-conjugated rabbit anti-goat immunoglobulin G (IgG) (1 : 5,000, Jackson Immunoresearch, West Grove, PA, USA) and HRP-conjugated anti-mouse IgG antibody (1 : 5,000; Jackson Immunoresearch) were used for secondary antibodies. All data shown are representative of three experiments. Densitometric analyses were performed by using Multi Gauge V3.0 software (Fujifilm).
All experiments were carried out in triplicate, and results are expressed as means±standard deviations. Comparisons between samples were carried out using Student's t-test. SPSS ver. 15.0 (SPSS Inc., Chicago, IL, USA) was used to analyze the data with p-values<0.05 to be the statistically significant.
To investigate whether EPS could exert anti-inflammatory activity based on its anti-oxidant properties, the anti-inflammatory activity of EPS was tested on mouse skin in vivo. From physical examination, we could observe inflammatory changes, such as edema, scaling, and thickening (lichenification), in the dorsal skin of OX-treated mice. The OX-induced inflammatory changes were suppressed markedly in OX+EPS-treated mice (Fig. 1A). Semiquantitative scores of the three inflammatory criteria in the OX-treated group (n=4) versus the OX+EPS-treated group (n=4) were: 2.50±0.58 versus 1.50±0.58 for edema (p=0.097), 2.75±0.50 versus 1.50±0.58 for scaling (p=0.017), and 2.75±0.50 versus 1.25±0.50 for thickening (p=0.005), respectively (Table 1). In the histological study, inflammatory changes in the skin, including hyperkeratosis and spongiosis in the epidermis, as well as vasodilation and the presence of inflammatory cell infiltrates in the dermis, were clearly observed in OX-treated mice, but were less prominent in the OX+EPS-treated mice (hematoxylin and eosin [H&E] staining, ×100; Fig. 1B). Skin thickness, measured using a dial thickness gauge, in the OX-treated mice increased to 233.2%±22.52% versus the untreated control group. The OX-induced increase in skin thickness was less prominent in the OX+EPS-treated mice to be 201.6%±12.46% of the control group (Fig. 2). There was no statistically significant difference between the OX-treated and the OX+EPS-treated mice groups (p=0.328, n=4).
Next, we assessed the expression levels of COX-2 and NGF-used as biomarkers for skin inflammation-in skin extracts from the OX-treated mice, OX+EPS-treated mice, and untreated control mice groups (n=4 for each group). Using RT-PCR and western blot analyses, we found that mRNA and protein levels of COX-2 were upregulated in OX-treated mice, but this increase was suppressed markedly in OX+EPS-treated mice (Fig. 3A, B). Using RT-PCR, NGF mRNA was found to be upregulated in OX-treated mice, versus the control mice, but this was suppressed in OX+EPS-treated mice (Fig. 3A). Densitometric analysis to quantify the relative expression levels compared to the control group indicated a significant difference in expression levels of COX-2 and NGF between the OX-treated group and the OX+EPS-treated group in a dose-dependent manner (p<0.01; Fig. 3C).
To confirm our observation that EPS had an anti-inflammatory activity in mouse skin, we developed an in vitro system to assess skin inflammation. Based on a report that compound 48/80 could induce ROS production in mast cells in a manner similar to allergic inflammation6, we tested whether compound 48/80 had a similar effect on HaCaT cells. In a previous study, HaCaT cells were reported to produce COX-2 and MMP-9 when stimulated with ultraviolet B irradiation12. In preliminary experiments using the MTT cell viability assay, it was found that 0.1~12.8 µg/ml of compound 48/80 (Fig. 4A) and 0.1~1.6 mg/ml of EPS (Fig. 4B) were the optimum, non-toxic concentrations for use in HeCaT cells. Therefore, all following experiments were performed using a concentration of 10 µg/ml of compound 48/80. In a FACS analysis, EPS was found to effectively scavenge the compound 48/80-produced ROS from HaCaT cells in a dose-dependent manner, showing DCF-DA(+) signals to be 302% of the untreated control in compound 48/80-treated cells, 142% of the control in compound 48/80+EPS (0.5 mg/ml)-treated cells, and 104% of the control in compound 48/80+EPS (1.0 mg/ml)-treated cells (Fig. 4C).
From the above results, we confirmed that compound 48/80 stimulated HaCaT cells to produce ROS, which might, in turn, induce skin inflammation. As markers for skin inflammation, expression levels of COX-2 and NGF were measured in compound 48/80-treated HaCaT cells. In RT-PCR and western blot analysis, mRNA and protein levels of COX2 (Fig. 5A, B) were upregulated by treatment with 10 µg/ml of compound 48/80 for 6 h. Compound 48/80-induced COX-2 expression was suppressed markedly by EPS treatment in a dose-dependent manner, at doses of between 0.01 and 1.0 mg/ml at the mRNA level and between doses of 0.1 and 1.0 mg/ml at the protein level. Next, we checked the expression levels of NGF, a biomarker of neurogenic inflammation, in compound 48/80-treated HaCaT cells. In RT-PCR, NGF mRNA was upregulated by treatment with 10 µg/ml compound 48/80 for 24 h (Fig. 5C). The compound 48/80-induced NGF expression was suppressed by EPS treatment in a dose-dependent manner between doses of 0.01 and 1.0 mg/ml (Fig. 5C). Densitometric analysis showed that there was a statistically significant difference between HeCaT cells treated with compound 48/80-treated and HeCaT cells treated with compound 48/80+different concentrations of EPS (p<0.01; Fig. 5D).
After demonstrating the anti-inflammatory activity of EPS in vivo and in vitro, we examined the regulatory effects of EPS by assessing IκB expression levels in HaCaT cells. Western blot analyses indicated that EPS induced upregulation of IκBα, an inhibitor of NFκB, in a dose-dependent manner; this indicates that EPS exerts an anti-inflammatory effect by inhibiting NFκB transcription factor activity in HaCaT cells (Fig. 6).
In this study, we assessed whether EPS-with its potent anti-oxidant properties-is able to exert an antiinflammatory effect against skin inflammation. Oxidative stress, caused by overproduction of ROS and/or by insufficient scavenging of anti-oxidants, is known to induce harmful biological responses in the skin, such as inflammation, aging, and cancer. Oxidative stress in the skin is thought to induce dysfunction in cell signaling pathways, triggering downstream signaling cascades and producing inflammatory cytokines in skin inflammation14. This work demonstrates that EPS had potent anti-inflammatory activity in mouse skin in vivo; EPS suppressed OX-induced contact dermatitis in hairless mice, and EPS downregulated expression levels of COX-2 and NGF in skin extracts of OX-treated mice. The in vitro anti-inflammatory activity of EPS was examined using compound 48/80-treated HaCaT cells.
Unbalanced cellular redox status is known to cause skin inflammation, and it is also known that a variety of anti-oxidants are potent anti-inflammatory agents14. Although the beneficial effects of anti-oxidants such as resveratrol can be demonstrated in vitro, there is frequently little correlation between in vitro and in vivo activity. The inconsistency between in vitro and in vivo systems may simply due to there being insufficient levels of active ingredients to cause pharmacological activity in the body, or inefficient delivery of anti-oxidants. Recently, the popularity of resveratrol-containing foods and beverages has increased worldwide because of their antioxidant activities, and both grapes and red wine have been studied as natural sources of resveratrol. The resveratrol content of grape skin is 35.6~170.6 µg/g, and that of red wine is 0.05~10.9 µg/ml in the trans-form and 0.04~8.71 µg/ml in the cis-form15,16. Peanut sprouts have also been reported to be a source of resveratrol2,17,18. In our previous study, EPS was found to contain a relatively high concentration of trans-resveratrol (54.2 µg/ml) compared other sources2,19. Besides resveratrol, the presence of other polyphenols in EPS, such as epicatechin (EC), epicatechin gallate, epigallocatechin, and epigallocatechin-3-gallate1, are thought to further enhance its antioxidant activity. For this study, we prepared a more concentrated form of EPS containing 176.75 µg/ml of trans-resveratrol-a value far higher than the resveratrol content of red wine. To our knowledge, our new preparation of EPS contains the highest reported concentration of trans-resveratrol obtained from a natural source. Per-orally administered resveratrol has been reported to have a half-life of 8~14 min, suggesting that resveratrol is metabolized rapidly and would not be expected to show much biological activity in the body20. In contrast, per-cutaneous application of EPS was considered to be advantageous as it would not be metabolized as rapidly21.
Because epidermal KCs represent ~90% of all epidermal cells, some mediators in epidermal KCs are likely to play important roles in the pathogenesis of skin inflammation. For this study, we selected compound 48/80 as a non-immunological stimulator to induce ROS-mediated skin inflammation in HaCaT cells. In comparison with other chemicals (irritant or allergic) used in in vitro skin inflammatory models, compound 48/80 is thought to play a similar role to substance P (SP) in the stimulation of KCs during neurogenic inflammation. Various studies have clearly demonstrated that neurogenic inflammation plays a crucial role in the pathogenesis of skin inflammation7,9,22,23. Itch is known to be meditated primarily by histamine, and thus anti-H1 blockers are major drugs used to control itch in various skin inflammatory conditions. However, the fact that antihistamines do not alleviate itch effectively in many chronic eczemas indicates that there are other mediators beyond mast cell-derived histamine. Recent studies have suggested that NPs, NTs, proteases, cytokines, and opioids may also be involved in a histamine-independent itch pathway23. The itch sensation is mediated by unmyelinated, afferent C-fibers derived from the dorsal root ganglia, and NGF is the major NT to be expressed at C-fiber endings24. Damage to skin by scratching is an important triggering factor inducing NGF expression, which, in turn, facilitates abnormal innervation of C-fibers with upregulated NGF expression in the epidermis25,26. Thus, NGF is considered to play an important role in skin inflammation in the epidermis. For example, there is a positive correlation between NGF levels in the epidermis and itch severity or objective symptoms of chronic eczema and psoriasis9,26. NGF levels in the horny layer of AD patients reflect both the severity of itch and the extent of skin lesions9, suggesting that NGF may be a good marker to predict the severity of subjective symptoms (itch) and/or objective symptoms (skin eruptions) in chronic eczema. Tumor necrosis factor-α (TNF-α) and interleukin (IL)-1 are key proinflammatory cytokines in AD to induce NGF production, which activates T cells and thus stimulates the production of IL-427,28. Thus, we selected NGF as a suitable marker for assessing the anti-inflammatory effect of EPS in HaCaT cells or skin extracts. In this study, NGF was found to be upregulated by compound 48/80, and the effect could be blocked by EPS treatment in HaCaT cells. This suggests that compound 48/80-stimualted HaCaT cells could be a useful new cell model for studying ROS-mediated skin inflammation. The results from our in vitro model of skin inflammation were consistent with those from the contact dermatitis model of mice in vivo, in that NGF and COX-2 were up-regulated by compound 48/80.
Compound 48/80 is commonly used to stimulate mast cells to release histamine, by immunological or non-immunological mechanisms. Compound 48/80 was reported to play either a similar role of SP, which induces histamine production in mast cells, or to upregulate COX-2 expression3,22,29. SP augments the production of pro-inflammatory cytokine of IL-1 from KCs30. In a previous study, compound 48/80 up-regulated SP and NGF, and it induced ROS production from mast cells31. Interestingly, compound 48/80 was reported to induce skin inflammation, especially neurogenic inflammation, in mice32,33. In this study, we treated HaCaT cells with compound 48/80, instead of SP, in order to induce ROS production and skin inflammation. In our study, compound 48/80-stimulated HaCaT cells produced ROS, which are thought to act as second messengers, and might induce skin inflammation by upregulating biomarkers such as COX-2 and NGF. The compound 48/80-induced inflammation (via ROS) in HaCaT cells was further confirmed by our results that EPS blocked ROS production in HaCaT cells, as it suppressed the expression levels of biomarkers of skin inflammation. The ear swelling response (ESR) has been commonly used as a non-invasive technique to measure inflammatory responses in intact skin in vivo34. For this study, we modified the ESR technique to allow the degree of skin inflammation to be semi-quantified. The technique is considered to be invasive and aggressive for mice, but we can semi-quantify the degree of skin inflammation in vivo without further manipulation. Using our new technique, we could easily detect the decrease in skin thickness in the OX+EPS-treated group versus the OXtreated group (Fig. 3).
In conclusion, EPS was found to have anti-inflammatory activity via its anti-oxidant properties in both a mouse model of OX-induced contact dermatitis and compound 48/80-treated HaCaT cells in vitro. In addition, we propose a new cell model to test neurogenic inflammation of the skin by treating HaCaT cells with compound 48/80. From these results, we expect to further develop EPS for use as a novel anti-inflammatory agent against skin inflammation in chronic eczemas.
Figures and Tables
![]() | Fig. 1Oxazolone (OX)-induced inflammatory changes in mouse skin are suppressed by application of 5% ethanol extract of peanut sprouts (EPS). (A) Representative pictures of untreated control mouse, OX-treated mouse, and OX+EPS-treated mouse. (B) Histological results of OX-treated and OX+EPS-treated mice are shown (H&E, ×100). |
![]() | Fig. 2Anti-inflammatory activity of ethanol extract of peanut sprouts (EPS) reduces the oxazolone (OX)-induced increase of skin thickness in hairless mice. Skin thickness was measured with a dial thickness gauge to semi-quantify the degree of skin inflammation in vivo. Skin flaps were obtained from the dorsal skin of untreated control, OX-treated, and OX+EPS-treated mice. Statistical significance was assessed between the OX-treated and OX+EPS-treated mice groups. |
![]() | Fig. 3Anti-inflammatory activity of ethanol extract of peanut sprouts (EPS) on oxazolone (OX)-induced skin inflammation in hairless mice. (A) In reverse transcriptase-polymerase chain reaction (RT-PCR) and (B) western blot analyses for COX-2, as well as (A) RT-PCR for nerve growth factor (NGF) mRNA expression, OX-treated mice (n=4) showed increased expression levels of the markers tested, but the increase was suppressed markedly in OX+EPS-treated mice (n=4). (C) Densitometric analysis of mRNA and protein bands of A, B was performed, and data represent mean±standard deviation of relative density of each group versus control mice without OX and EPS treatment (n=4 for each group). #p<0.01 between OX-treated and OX+EPS-treated mice groups. Control refers to the OX-untreated mice group. |
![]() | Fig. 4Anti-inflammatory activity of ethanol extract of peanut sprouts (EPS) via reactive oxygen species (ROS)-scavenging effect in compound 48/80-treated HaCaT cells. MTT assay was used to detect non-cytotoxic concentrations of (A) compound 48/80 and (B) EPS in HaCaT cells. (C) Fluorescence-activated cell sorting (FACS) analysis demonstrated that compound 48/80 induced ROS production in HaCaT cells, which was suppressed markedly by EPS treatment. |
![]() | Fig. 5Inhibitory effect of ethanol extract of peanut sprouts (EPS) on compound 48/80-induced expression of cyclooxygenase-2 (COX-2) and nerve growth factor (NGF) in HaCaT cells. In (A) reverse transcriptase-polymerase chain reaction (RT-PCR) and (B) western blot analyses for COX-2, and (C) RT-PCR for NGF mRNA expression, compound 48/80 (10 µg/ml) increased expression levels of COX-2 and NGF, but this induction was suppressed markedly in compound 48/80+EPS-treated HaCaT cells in a dose-dependent manner between 0.01 and 1.0 mg/ml at the mRNA level and 0.1 and 1.0 mg/ml at the protein level. (D) Densitometric analysis for mRNA and protein bands of (A~C) was performed, and data represent means±standard deviations of three independent experiments. #p<0.01 between compound 48/80-treated and compound 48/80+EPS-treated sample with different EPS concentration. N.S. means no significance between samples. GAPDH: glyceraldehyde 3-phosphate dehydrogenase. |
![]() | Fig. 6Ethanol extract of peanut sprouts (EPS) exerts an anti-inflammatory activity by upregulating IκBα expression in compound 48/80-treated HaCaT cells. (A) In western blot analysis, compound 48/80 down-regulated IκBα expression, which was reversed by EPS treatment at a dose of 10 µg/ml in HaCaT cells. The EPS-induced upregulation of IκBα occurred in a time-dependent manner between 15 and 60 min. (B) Densitometric analysis of western blot protein bands was performed, and data represent means±standard deviations of three independent experiments. #p<0.01 between compound 48/80-treated and compound 48/80+EPS-treated samples at each time point (15, 30, and 60 min). |
Table 1
Semi-quantitative analysis on inflammatory scores (n=4)
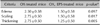
Values are presented as mean±standard deviation. OX: oxazolone, EPS: ethanol extract of peanut sprouts. *The degree of skin inflammation was arbitrarily graded by physical examination. Severity of edema, scaling, and thickening was graded as 0 (none)~3 (severe), and statistical significance was calculated between OX-treated and OX, EPS-treated mice groups.
ACKNOWLEDGMENT
This study was supported by a grant Chonnam National University Hospital Research Institute of Clinical Medicine (CRI-11079-21).
References
2. Choi JY, Choi DI, Lee JB, Yun SJ, Lee DH, Eun JB, et al. Ethanol extract of peanut sprout induces Nrf2 activation and expression of antioxidant and detoxifying enzymes in human dermal fibroblasts: implication for its protection against UVB-irradiated oxidative stress. Photochem Photobiol. 2013; 89:453–460.


3. Gallicchio M, Rosa AC, Benetti E, Collino M, Dianzani C, Fantozzi R. Substance P-induced cyclooxygenase-2 expression in human umbilical vein endothelial cells. Br J Pharmacol. 2006; 147:681–689.


4. Aridor M, Rajmilevich G, Beaven MA, Sagi-Eisenberg R. Activation of exocytosis by the heterotrimeric G protein Gi3. Science. 1993; 262:1569–1572.


5. Ferry X, Brehin S, Kamel R, Landry Y. G protein-dependent activation of mast cell by peptides and basic secretagogues. Peptides. 2002; 23:1507–1515.


6. Okayama Y. Oxidative stress in allergic and inflammatory skin diseases. Curr Drug Targets Inflamm Allergy. 2005; 4:517–519.


7. Truzzi F, Marconi A, Pincelli C. Neurotrophins in healthy and diseased skin. Dermatoendocrinol. 2011; 3:32–36.


8. Aubdool AA, Brain SD. Neurovascular aspects of skin neurogenic inflammation. J Investig Dermatol Symp Proc. 2011; 15:33–39.


9. Yamaguchi J, Aihara M, Kobayashi Y, Kambara T, Ikezawa Z. Quantitative analysis of nerve growth factor (NGF) in the atopic dermatitis and psoriasis horny layer and effect of treatment on NGF in atopic dermatitis. J Dermatol Sci. 2009; 53:48–54.


10. Lee JH, Piao MS, Choi JY, Yun SJ, Lee JB, Lee SC. Up-regulation of cyclooxygenase 2 and matrix metalloproteinases-2 and -9 in cutaneous squamous cell carcinoma: active role of inflammation and tissue remodeling in carcinogenesis. Ann Dermatol. 2013; 25:145–151.


11. Lee JL, Mukhtar H, Bickers DR, Kopelovich L, Athar M. Cyclooxygenases in the skin: pharmacological and toxicological implications. Toxicol Appl Pharmacol. 2003; 192:294–306.


12. Anggakusuma , Yanti , Hwang JK. Effects of macelignan isolated from Myristica fragrans Houtt. on UVB-induced matrix metalloproteinase-9 and cyclooxygenase-2 in HaCaT cells. J Dermatol Sci. 2010; 57:114–122.


13. Man MQ, Hatano Y, Lee SH, Man M, Chang S, Feingold KR, et al. Characterization of a hapten-induced, murine model with multiple features of atopic dermatitis: structural, immunologic, and biochemical changes following single versus multiple oxazolone challenges. J Invest Dermatol. 2008; 128:79–86.


14. Portugal M, Barak V, Ginsburg I, Kohen R. Interplay among oxidants, antioxidants, and cytokines in skin disorders: present status and future considerations. Biomed Pharmacother. 2007; 61:412–422.


15. Pascual-Martí MC, Salvador A, Chafer A, Berna A. Supercritical fluid extraction of resveratrol from grape skin of Vitis vinifera and determination by HPLC. Talanta. 2001; 54:735–740.


16. Paulo L, Domingues F, Queiroz JA, Gallardo E. Development and validation of an analytical method for the determination of trans- and cis-resveratrol in wine: analysis of its contents in 186 Portuguese red wines. J Agric Food Chem. 2011; 59:2157–2168.


17. Kang HI, Kim JY, Kwon SJ, Park KW, Kang JS, Seo KI. Antioxidative effects of peanut sprout extracts. J Korean Soc Food Sci Nutr. 2010; 39:941–946.


18. Kim HJ, Kang JS, Park HR, Hwang YI. Neuroprotective effects of methanolic extracts from peanut sprouts. J Life Sci. 2010; 20:253–259.


19. Wang KH, Lai YH, Chang JC, Ko TF, Shyu SL, Chiou RY. Germination of peanut kernels to enhance resveratrol biosynthesis and prepare sprouts as a functional vegetable. J Agric Food Chem. 2005; 53:242–246.


20. Baur JA, Sinclair DA. Therapeutic potential of resveratrol: the in vivo evidence. Nat Rev Drug Discov. 2006; 5:493–506.


21. Hung CF, Lin YK, Huang ZR, Fang JY. Delivery of resveratrol, a red wine polyphenol, from solutions and hydrogels via the skin. Biol Pharm Bull. 2008; 31:955–962.


22. Le Filliatre G, Sayah S, Latournerie V, Renaud JF, Finet M, Hanf R. Cyclo-oxygenase and lipoxygenase pathways in mast cell dependent-neurogenic inflammation induced by electrical stimulation of the rat saphenous nerve. Br J Pharmacol. 2001; 132:1581–1589.


23. Tominaga M, Takamori K. An update on peripheral mechanisms and treatments of itch. Biol Pharm Bull. 2013; 36:1241–1247.


24. Albers KM, Wright DE, Davis BM. Overexpression of nerve growth factor in epidermis of transgenic mice causes hypertrophy of the peripheral nervous system. J Neurosci. 1994; 14:1422–1432.


25. Kou K, Nakamura F, Aihara M, Chen H, Seto K, Komori-Yamaguchi J, et al. Decreased expression of semaphorin-3A, a neurite-collapsing factor, is associated with itch in psoriatic skin. Acta Derm Venereol. 2012; 92:521–528.


26. Nakamura M, Toyoda M, Morohashi M. Pruritogenic mediators in psoriasis vulgaris: comparative evaluation of itch-associated cutaneous factors. Br J Dermatol. 2003; 149:718–730.


27. Santambrogio L, Benedetti M, Chao MV, Muzaffar R, Kulig K, Gabellini N, et al. Nerve growth factor production by lymphocytes. J Immunol. 1994; 153:4488–4495.
28. Shi Y, Jin Y, Guo W, Chen L, Liu C, Lv X. Blockage of nerve growth factor modulates T cell responses and inhibits allergic inflammation in a mouse model of asthma. Inflamm Res. 2012; 61:1369–1378.


29. Jallat-Daloz I, Cognard JL, Badet JM, Regnard J. Neuralepithelial cell interplay: in vitro evidence that vagal mediators increase PGE2 production by human nasal epithelial cells. Allergy Asthma Proc. 2001; 22:17–23.


30. Song IS, Bunnett NW, Olerud JE, Harten B, Steinhoff M, Brown JR, et al. Substance P induction of murine keratinocyte PAM 212 interleukin 1 production is mediated by the neurokinin 2 receptor (NK-2R). Exp Dermatol. 2000; 9:42–52.


31. Brooks AC, Whelan CJ, Purcell WM. Reactive oxygen species generation and histamine release by activated mast cells: modulation by nitric oxide synthase inhibition. Br J Pharmacol. 1999; 128:585–590.


32. Baolin L, Weiwei W, Ning T. Topical application of luteolin inhibits scratching behavior associated with allergic cutaneous reaction in mice. Planta Med. 2005; 71:424–428.

