Abstract
Background
Diabetic wounds are a major clinical challenge, because minor skin wounds can lead to chronic, unhealed ulcers and ultimately result in infection, gangrene, or even amputation. Studies on bone marrow derived mesenchymal stem cells (BMSCs) and a series of growth factors have revealed their many benefits for wound healing and regeneration. Platelet-rich plasma (PRP) may improve the environment for BMSC development and differentiation. However, whether combined use of BMSCs and PRP may be more effective for accelerating diabetic ulcer healing remains unclear.
Objective
We investigated the efficacy of BMSCs and PRP for the repair of refractory wound healing in a diabetic rat model.
Methods
Forty-eight rats with diabetes mellitus induced by streptozotocin were divided into four groups: treatment with BMSCs plus PRP, BMSCs alone, PRP alone, phosphate buffered saline. The rate of wound closure was quantified. A histopathological study was conducted regarding wound depth and the skin edge at 7, 14, and 28 days after surgery.
Results
Wound healing rates were significantly higher in the BMSC plus PRP group than in the other groups. The immunohistochemistry results showed that the expression of platelet/endothelial cell adhesion molecule 1, proliferating cell nuclear antigen, and transforming growth factor-β1 increased significantly in the BMSC plus PRP group compared to the other treatment groups. On day 7, CD68 expression increased significantly in the wounds of the BMSC plus PRP group, but decreased markedly at day 14 compared to the controls.
Diabetic foot ulcers (DFUs), a significant complication in diabetes, have a significant impact on the quality of life and mortality of patients1. It is estimated that approximately 170 million patients have diabetes worldwide2. Normal wound repair is a complex and dynamic process, including inflammation, angiogenesis, granulation tissue formation, and remodeling3. Diabetic wounds exhibit impaired angiogenesis, reduced growth factor levels, and reduced chemotactic ability to recruit inflammatory cells to the wound4. Poor vascularization and maintenance of a chronic inflammatory state limit the healing capacity of DFUs. Many studies have suggested that bone marrow derived mesenchymal stem cells (BMSCs) contribute to rapid healing in a variety of reconstructive and restorative surgeries5-7. In addition, platelet-rich plasma (PRP) therapy is an efficacious treatment modality for DFUs8,9.
BMSCs represent a small portion of the cells in the bone marrow stromal compartment but have the potential to differentiate into multiple lineages, including osteoblasts, adipocytes, myoblasts, epithelial, and neurons cells10. In diabetics, the contribution of BMSCs to skin repair may rely on independent immunomodulatory actions and recruitment of cells to neo-angiogenic sites to accelerate revascularization11. Neovascularization supports newly formed tissue and transports circulatory cells to the wound region12. BMSCs recruit endogenous stem cells to neoangiogenic sites to accelerate the revascularization process and participate in the inflammatory phase of wound repair to initiate wound healing13. BMSCs can be obtained in a culture, making them a cost-effective alternative for the treatment of chronic wounds.
PRP has been used in a wide variety of clinical treatments and surgical procedures, specifically in the field of soft tissue ulcerations and chronic skin disease. PRP contains more than 20 types of growth factors, such as platelet-derived growth factor, vascular endothelial growth factor (VEGF), and transforming growth factor (TGF)-β114. These factors not only regulate cell migration and proliferation but also remodel the extracellular matrix (ECM) and promote angiogenesis, creating a beneficial environment that enhances wound healing15. In the present study, we evaluated the beneficial effects of BMSCs and PRP for diabetic wound repair.
Forty-eight male Sprague-Dawley rats (weight, 200 to 220 g) were obtained from the Animal Center of Wenzhou Medical College (Wenzhou, China). The animals were housed in spacious cages and were given food and water ad libitum. The animal room was ventilated and was under a 12 hours/12 hours light-dark schedule. All animal care complied with the legal guidelines, and the experimental procedures were conducted following approval by the Wenzhou Medical College Animal Policy and Welfare Committee (Approval Document NO. 2009/APWCWC/0031).
Diabetes was induced by a single intraperitoneal injection of streptozotocin (STZ) (Sigma, St. Louis, MO, USA) dissolved in sodium citrate (0.1 mM, pH 4.5) at a dose of 60 mg/kg as described previously16. Blood glucose levels were measured by blood tail sampling with a glucometer (B. Braun, Seoul, Korea). Rats with glucose levels >300 mg/d (16.7 mmol/L) were considered diabetic and manifested the diabetic state for 4 weeks prior to the study.
BMSCs were harvested from the femurs and tibias of normal male rats (100 g) under aseptic conditions according to methods described previously with minor modifications17. Briefly, the cells were cultured in DMEM/F-12 medium (Gibco, Grand Island, NY, USA), supplemented with 10% fetal bovine serum (Gibco) 100 U/ml penicillin (Invitrogen, Carlsbad, CA, USA), and 100 µg/ml streptomycin (Invitrogen). BMSCs at passage 3 were used for treatment.
BMSCs harvested at passage 2 were assessed by flow cytometry (Epics-XL; Beckman Coulter, Tokyo, Japan). The cells were incubated with fluorescein isothiocyanate-conjugated polyclonal antibodies against rat CD29, CD45, CD90, and CD11b/c (all from Biolegend, San Diego, CA, USA).
PRP was harvested via the double centrifugation of blood, as described previously18. Human blood was collected from healthy volunteers in a container containing citric acid-citrate-dextrose anticoagulant. The whole blood was centrifuged at 2,400 rpm for 10 minutes at 22℃. The sample was further centrifuged at 3,500 rpm for 15 minutes to obtain the platelet concentrate19. Platelets were counted using an automated hematology analyzer (XE-2100; Sysmex, Kobe, Japan). Platelet counts >1×109 platelets/ml were used. A solution of 1,000 IU thrombin (Sigma) and 10% calcium chloride was added to the PRP at a volume ratio of 10:1 to activate the platelets. The sample was incubated for 1 hour at room temperature, centrifuged at 3,000 × g for 20 minutes, and the supernatant was collected20. The supernatant (activated PRP) was stored at -80℃ until use.
Rats with diabetes mellitus induced by STZ (DMIS rats) were weighed and anesthetized with 60 mg/kg Nembutal (Sigma). Abdominal hair was clipped and depilated, and the skin was cleansed with Betadine and 70% alcohol solution. A standardized 2 cm2 full-thickness wound was made. Each rat had two wounds created, one on the left side and one on the right side. The wounds were photographed and covered with semi-occlusive polyurethane dressings (Tegaderm; 3M Health Care, St. Paul, MN, USA) to avoid desiccation. Approximately 6×106 BMSCs were resuspended in 0.2 ml phosphate buffered saline (PBS) and injected at 10 different sites. PRP was injected through the dressing into the wounds. All rats were grouped randomly as follows: treatment with BMSC plus PRP (n=12), BMSCs (n=12), PRP (n=12), and PBS as controls (n=12).
Digital photographs were taken every day after surgery and were compared to initial photographs (0 day) using planimetric methods (Image J1.38e; National Institutes of Health, Bethesda, MD, USA). The investigators who measured the wounds were blinded. Wound closure was quantified by measuring the reepithelialization and open wound as a percentage of the original wound area, which was calculated as (1-open wound area/original wound area) ×100%.
Sections (5 µm thick) were stained according to routine hematoxylin and eosin (H&E) protocols. Panoramic crosssectional digital images of each wound were prepared using the protocols and were then examined histologically; the collagen formation assay was then performed after Masson's trichrome staining. These sections were viewed under a fluorescence microscope (ECLIPSE 80i; Nikon, Tokyo, Japan) and the images were blindly quantified by an independent observer.
After deparaffinization and rehydration, paraffin-embedded sections (4 µm thick) were treated with 3% hydrogen peroxide for 10 minutes and then with 1% bovine albumin in PBS for 1 hour. The sections were incubated at 4℃ overnight with the primary antibodies against proliferating cell nuclear antigen (PCNA) (1:200; Santa Cruz Biotechnology, Santa Cruz, CA, USA), platelet/endothelial cell adhesion molecule 1 (PECAM-1) (1:50; Santa Cruz Biotechnology), CD68 (1:200; Abcam, Cambridge, UK), or TGF-β1 (1:200; Boster, Wuhan, China). After specific binding of the secondary antibodies to the primary antibodies and conducting the streptavidin horseradish peroxidase reaction together with 3,3-diaminobenzidine tetrahydrochloride solution (Sigma), the images were viewed under a fluorescence microscope (×40, Nikon).
Homogenized skin tissue samples were ground and lysed. Then, 50 mg protein was denatured and separated via 10% sodium dodecyl sulfate-polyacrylamide gel electrophoresis and blotted onto a nitrocellulose membrane. The blots were blocked with 5% milk in Tris-buffered saline (pH 7.6) for 1 hour at room temperature. Each nitrocellulose membrane was incubated with specific antibodies. Immunoreactive bands were detected using enhanced chemiluminescence reagents (Bio-Rad, Hercules, CA, USA). The amount of protein was analyzed using Image J analysis software version 1.38e (National Institutes of Health) and normalized to their respective controls.
A flow cytometry analysis of the BMSCs from passage 2 showed that they expressed typical CD90 and CD29 surface markers but were negative for CD11b/c and pan-hematopoietic CD45. More than 90% of the cultured BMSCs were positive for CD90 and CD 29 but <2% of the cells were positive for CD45 and CD11b/c (Fig. 1).
Digital photographs and the rate of wound closure are shown in Fig. 2. Wound closure increased significantly in the BMSC plus PRP group compared to the PRP (p<0.05) and BMSC (p<0.05) groups at 3, 7, 10, 14, and 17 days after wounding. On day 21 after surgery, 96.1% wound closure was reached in the BMSC plus PRP group, which was considered completely healed, whereas control wounds reached 72% closure (Fig. 2B).
Masson's trichrome-stained images are shown in Fig. 3. Collagen protein expression was higher on the BMSCs plus PRP side, with better organization of the thicker collagen fiber bundles than that on the PRP-only side (Fig. 3A~D). In addition, H&E staining suggested that mean epidermal thickness increased significantly and that sweat gland-like structures were enhanced in the BMSC plus PRP tissues compared to the control group, suggesting better cutaneous regeneration (Fig. 3E~H).
Wound tissues were stained for PECAM-1 to quantitatively assess angiogenesis. The results revealed that treatment with BMSCs plus PRP stimulated blood vessel formation within the diabetic wound bed at day 7 post-injury (Fig. 4A). Wounds treated with BMSCs and PRP had 1.5-fold more blood vessels than those in animals treated with PRP alone (p=0.0014). The number of blood vessels within the wounds of BMSC-treated rats was equal to that in the PRP-treated wounds (p>0.05).
We performed immunohistochemistry staining of wound tissues with the anti-PCNA antibody to assess cell proliferation (Fig. 5). PCNA-positive cells in the subcutis increased markedly in the BMSC plus PRP group compared to the PRP-treated group (p<0.05). In addition, the PCNA-positive cells that appeared in the subcutis and epidermis increased significantly in the BMSC plus PRP rats on day 14 compared to rats in the control group (p<0.05). The skin tissues from each group were collected and homogenized, and proteins were detected using Western blot analysis. As shown in Fig. 5C~D, a significant increase was shown in PCNA protein expression after treatment with BMSCs plus PRP compared to BMSCs (p<0.0001) and PRP alone (p<0.001).
Wound tissues were stained for CD68 to observe macrophage infiltration. A 1.4-fold increase was observed in CD68 expression in the wounds of the BMSC plus PRP group compared to the PRP wounds on day 7 after wounding (p<0.05). Significantly fewer wounds were observed in the BMSC plus PRP group than in the other groups on day 14 (p<0.05; Fig. 6B). The Western blot data also showed that BMSCs and PRP showed increased CD68 protein expression during the early phase of wound healing, which decreased significantly at day 14 after wounding (Fig. 6D).
Immunohistochemistry staining for TGF-β1 revealed that applying BMSCs and PRP to diabetic wounds effectively promoted TGF-β1 expression (Fig. 7A). The number of TGF-β1-positive cells increased in the BMSC plus PRP group on day 7, relative to the PRP group (p<0.05; Fig. 7B), and the increased TGF-β1 expression appeared mainly in the Western blot data (Fig. 7C, D).
Although a number of recent studies have reported that cell therapy and growth factors improve diabetic wound healing, diabetic wounds remain a significant clinical problem. Wound healing in patients with diabetes is a complex and multifactorial process due to dysregulated molecular interactions and signal transductions that delay chronic wound healing21,22. Studies suggest that BMSCs can differentiate into other cells within the injured tissue to promote repair and regeneration of the skin23. PRP may offer a suitable microenvironment for BMSCs to promote proliferation and differentiation. Our results show that the combined treatment of BMSCs and PRP accelerates wound healing by increasing angiogenesis, enhancing cell proliferation, affecting the infiltration response, and inducing TGF-β1 expression.
The skin-healing rates in DMIS rats treated with BMSCs alone, PRP alone, BMSCs plus PRP, or PBS were compared. Among the four treatment groups, the most effective was the BMSC plus PRP group (Fig. 2B). At day 7, this group showed significantly better wound closure (44.0%±2.4%) compared to the BMSC-treated group (24.7%±3.2%). Masson's trichrome-stained sections showed that the BMSC plus PRP group exhibited a greater number, and a higher density, of collagen fibers (Fig. 3A~E). Collagen is an important ECM component that provides integrity and structure to normal tissues24. These results are in good agreement with the recent work by Elsharawy et al.25.
Our results on vessel density clearly suggest that both BMSCs and PRP can induce a robust angiogenic response in healing wounds. In particular, wounds treated with BMSCs plus PRP showed a 12.5 blood vessels/high power field (HPF) compared to 8 and 4.5 blood vessels/HPF in wounds treated with BMSCs alone and PBS, respectively, at day 7 (p<0.05; Fig. 4B). The angiogenic response is crucial for wound healing, and is necessary to increase oxygen and nutrient delivery, ensure the survival of keratinocytes, and sustain newly formed granulation tissue. PRP is a rich source of growth factors that stimulates angiogenesis in diabetic wounds26. Studies have shown that angiogenic factors including VEGF, hepatic growth factor, and angiopoietins are released from injured tissue and that BMSCs are recruited to the wound27. Our data concurs with the findings mentioned above, supporting the hypothesis that BMSCs in combination with PRP have a direct or indirect modulating effect on angiogenesis through the growth factors involved in wound healing.
In addition, the number of PCNA-positive cells increased significantly at days 7 and 14 in the BMSC plus PRP group, and was 1.7-fold greater in wounds of animals treated with PRP alone (Fig. 5B). These results show that combined treatment with BMSCs and PRP may increase fibroblasts and keratinocytes in wound tissue. Fibroblasts repair and remodel wounded skin, and the migration of keratinocytes promotes re-epithelialization during the healing process. The BMSC plus PRP group showed 1.5-fold and 1.4-fold higher TGF-β1 expression than PRP and BMSCs alone, respectively (Fig. 7B). This increased expression may promote the synthesis of collagen proteins, fiberconnexin, and integrin in fibroblasts and enhance epithelial cell migration28. We speculate that BMSCs and PRP stimulate the proliferation of granulation tissue cells, promote the proliferation of epithelial cells, and increase TGF-β1 expression during the early phase of healing.
CD68, a specific marker for monocytes/macrophages, showed an elevated expression at day 7 in wounds treated with BMSC plus PRP (264.0±18.8 cells/HPF) but the levels decreased significantly at day 14 after surgery (85.67±13.5 cells/HPF). However, the control animals had significantly increased CD68 levels on day 14 (177.3±11.85 cells/HPF; Fig. 6B). BMSCs may be involved in active inflammation during repair and healing of chronic unhealed wounds29. While inflammation is essential for successful wound healing, prolonged or excess inflammation can result in unhealed chronic wounds that remain in a persistently inflamed state. Studies have shown that PRP may inhibit excessive early inflammation and interact with macrophages to improve tissue healing and regeneration30. These data suggest that applying BMSCs and PRP activates the inflammatory phase of wound healing earlier and limits inflammation during the late phase of wound healing.
Applying BMSCs or PRP alone is likely to be insufficient in improving the healing of healthy skin in patients with DFUs. However, applying BMSCs and PRP may be an advantageous way to treat DFUs. Improved treatments will result from a greater understanding of the molecular pathway of BMSCs and PRP, including homing of BMSCs to the wound, and in understanding how PRP supports and regulates the activation and differentiation of BMSCs during wound healing and its possible implications for treating patients who have chronic wounds caused by irradiation, diabetes, or burns.
Figures and Tables
Fig. 1
Flow cytometry analysis of cultured bone marrow-derived mesenchymal stem cells. The samples had no surface marker expression for hematopoietic stem cells (CD45 [2.0%] and CD11b/c [1.7%]), but highly expressed the surface markers for mesenchymal stem cells (CD29 [98.8%] and CD90 [98.4%]). SD: side scatter.
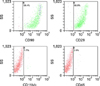
Fig. 2
Treatment with bone marrow-derived mesenchymal stem cells (BMSCs) plus platelet-rich plasma (PRP) improves wound healing in diabetic rats. (A) Representative photographs of skin wounds from each group at various time points after surgery. (B) Wound closure rates. Results represent the mean±standard error; n=3 rats. *p<0.05 vs. PRP, *p<0.05 vs. BMSCs.

Fig. 3
Masson's trichrome staining (A~D: ×40) and H&E staining (E~H: ×20) of wounded skin sections. Results from a representative animal of four studied in each group are shown. BMSCs: bone marrow-derived mesenchymal stem cells, PRP: platelet-rich plasma.
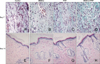
Fig. 4
Effects of bone marrow-derived mesenchymal stem cells (BMSCs) and platelet-rich plasma (PRP) on wound vascularity. (A) Platelet/endothelial cell adhesion molecule 1 staining of blood vessels in the wound bed (×40). a: Control, b: BMSCs, c: PRP, d: BMSCs plus PRP. Results from a representative animal of four studied from each group are shown. (B) Quantification of vessel density. Bar graph shows mean±standard error in each group. HPF: high power field. *p<0.05 vs. control, **p<0.05 vs. PRP, **p<0.05 vs. BMSCs.
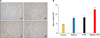
Fig. 5
Assessment of cell proliferation in wounds by proliferating cell nuclear antigen (PCNA). (A) PCNA staining (brown) of proliferating cells in the wound bed (×40). Results from a representative animal of four studied in each group are shown. (B) Quantification of PCNA-positive cells. HPF: high power field. *p<0.05 vs. control, **p<0.05 vs. platelet-rich plasma (PRP), **p<0.05 vs. bone marrow-derived mesenchymal stem cells (BMSCs). (C) The PCNA protein was detected by Western blot. Glyceraldehyde 3-phosphate dehydrogenase (GAPDH) was used as the loading control. (D) The column figure shows the normalized optical density of PCNA/GAPDH. Columns represent mean±standard error of three independent experiments. *p<0.0001 vs. control, **p<0.001 vs. PRP, **p<0.0001 vs. BMSCs.

Fig. 6
Effect of treatment with bone marrow-derived mesenchymal stem cells (BMSCs) and platelet-rich plasma (PRP) on the inflammatory response during wound healing. (A) CD68 staining (brown) of proliferating cells in the wound bed (×40). Results from a representative animal of four studied in each group are shown. (B) Quantification of CD68-positive cells. HPF: high power field. *p<0.05 vs. control, **p<0.05 vs. PRP, **p<0.05 vs. BMSCs. (C) CD68 protein level was detected by Western blot, and glyceraldehyde 3-phosphate dehydrogenase (GAPDH) was used as the loading control. (D) The column figure shows the normalized optical density of CD68/GAPDH. Columns represent mean±standard error of three independent experiments. *p<0.05 vs. control, **p<0.05 vs. PRP, **p<0.05 vs. BMSCs.
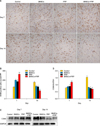
Fig. 7
Effect of treatment with bone marrow-derived mesenchymal stem cells (BMSCs) and platelet-rich plasma (PRP) on transforming growth factor-β1 (TGF-β1) level. (A) TGF-β1 staining (brown) of proliferating cells in the wound bed (×40). a: Control, b: BMSCs, c: PRP, d: BMSCs plus PRP. Results from a representative animal out of four studied in each group are shown. (B) Quantification of TGF-β1-positive cells. HPF: high power field. *p<0.05 vs. control, **p<0.05 vs. PRP, **p<0.05 vs. BMSCs. (C) TGF-β1 protein level was detected by Western blot. Glyceraldehyde 3-phosphate dehydrogenase (GAPDH) was used as the loading control. (D) The column figure shows the normalized optical density of TGF-β1/GAPDH. Columns represent mean±standard error of three independent experiments. *p<0.05 vs. control, **p<0.05 compared to any other group.
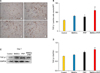
ACKNOWLEDGMENT
Financial support was provided by the National Natural Science Funding of China (80271772, 81071751) and a Medicine and Health Grant from Wenzhou Bureau of Science and Technology (Y20090251).
References
1. Blumberg SN, Berger A, Hwang L, Pastar I, Warren SM, Chen W. The role of stem cells in the treatment of diabetic foot ulcers. Diabetes Res Clin Pract. 2012; 96:1–9.


2. Brem H, Tomic-Canic M. Cellular and molecular basis of wound healing in diabetes. J Clin Invest. 2007; 117:1219–1222.


4. Ansurudeen I, Sunkari VG, Grünler J, Peters V, Schmitt CP, Catrina SB, et al. Carnosine enhances diabetic wound healing in the db/db mouse model of type 2 diabetes. Amino Acids. 2012; 43:127–134.


5. Kwon DS, Gao X, Liu YB, Dulchavsky DS, Danyluk AL, Bansal M, et al. Treatment with bone marrow-derived stromal cells accelerates wound healing in diabetic rats. Int Wound J. 2008; 5:453–463.


6. Schneider RK, Anraths J, Kramann R, Bornemann J, Bovi M, Knüchel R, et al. The role of biomaterials in the direction of mesenchymal stem cell properties and extracellular matrix remodelling in dermal tissue engineering. Biomaterials. 2010; 31:7948–7959.


7. Halkos ME, Zhao ZQ, Kerendi F, Wang NP, Jiang R, Schmarkey LS, et al. Intravenous infusion of mesenchymal stem cells enhances regional perfusion and improves ventricular function in a porcine model of myocardial infarction. Basic Res Cardiol. 2008; 103:525–536.


8. Saad Setta H, Elshahat A, Elsherbiny K, Massoud K, Safe I. Platelet-rich plasma versus platelet-poor plasma in the management of chronic diabetic foot ulcers: a comparative study. Int Wound J. 2011; 8:307–312.


9. Frykberg RG, Driver VR, Carman D, Lucero B, Borris-Hale C, Fylling CP, et al. Chronic wounds treated with a physiologically relevant concentration of platelet-rich plasma gel: a prospective case series. Ostomy Wound Manage. 2010; 56:36–44.
10. Kuo YR, Goto S, Shih HS, Wang FS, Lin CC, Wang CT, et al. Mesenchymal stem cells prolong composite tissue allotransplant survival in a swine model. Transplantation. 2009; 87:1769–1777.


11. Akela A, Nandi SK, Banerjee D, Das P, Roy S, Joardar SN, et al. Evaluation of autologous bone marrow in wound healing in animal model: a possible application of autologous stem cells. Int Wound J. 2012; 9:505–516.


12. Li WW, Talcott KE, Zhai AW, Kruger EA, Li VW. The role of therapeutic angiogenesis in tissue repair and regeneration. Adv Skin Wound Care. 2005; 18:491–500.


13. Li YP, Paczesny S, Lauret E, Poirault S, Bordigoni P, Mekhloufi F, et al. Human mesenchymal stem cells license adult CD34+ hemopoietic progenitor cells to differentiate into regulatory dendritic cells through activation of the Notch pathway. J Immunol. 2008; 180:1598–1608.


14. Eppley BL, Woodell JE, Higgins J. Platelet quantification and growth factor analysis from platelet-rich plasma: implications for wound healing. Plast Reconstr Surg. 2004; 114:1502–1508.


15. Demidova-Rice TN, Wolf L, Deckenback J, Hamblin MR, Herman IM. Human platelet-rich plasma- and extracellular matrix-derived peptides promote impaired cutaneous wound healing in vivo. PLoS One. 2012; 7:e32146.


16. Raza H, Prabu SK, John A, Avadhani NG. Impaired mitochondrial respiratory functions and oxidative stress in streptozotocin-induced diabetic rats. Int J Mol Sci. 2011; 12:3133–3147.


17. Wang A, Ding X, Sheng S, Yao Z. Bone morphogenetic protein receptor in the osteogenic differentiation of rat bone marrow stromal cells. Yonsei Med J. 2010; 51:740–745.


18. Carter CA, Jolly DG, Worden CE Sr, Hendren DG, Kane CJ. Platelet-rich plasma gel promotes differentiation and regeneration during equine wound healing. Exp Mol Pathol. 2003; 74:244–255.


19. Bir SC, Esaki J, Marui A, Sakaguchi H, Kevil CG, Ikeda T, et al. Therapeutic treatment with sustained-release platelet-rich plasma restores blood perfusion by augmenting ischemia-induced angiogenesis and arteriogenesis in diabetic mice. J Vasc Res. 2011; 48:195–205.


20. Cho HS, Song IH, Park SY, Sung MC, Ahn MW, Song KE. Individual variation in growth factor concentrations in platelet-rich plasma and its influence on human mesenchymal stem cells. Korean J Lab Med. 2011; 31:212–218.


21. Medina A, Scott PG, Ghahary A, Tredget EE. Pathophysiology of chronic nonhealing wounds. J Burn Care Rehabil. 2005; 26:306–319.


22. Lee YH, Chang JJ, Chien CT, Yang MC, Chien HF. Antioxidant sol-gel improves cutaneous wound healing in streptozotocin-induced diabetic rats. Exp Diabetes Res. 2012; 2012:504693.


23. Schneider RK, Puellen A, Kramann R, Raupach K, Bornemann J, Knuechel R, et al. The osteogenic differentiation of adult bone marrow and perinatal umbilical mesenchymal stem cells and matrix remodelling in three-dimensional collagen scaffolds. Biomaterials. 2010; 31:467–480.


24. Ganeshkumar M, Ponrasu T, Krithika R, Iyappan K, Gayathri VS, Suguna L. Topical application of Acalypha indica accelerates rat cutaneous wound healing by up-regulating the expression of Type I and III collagen. J Ethnopharmacol. 2012; 142:14–22.


25. Elsharawy MA, Naim M, Greish S. Human CD34+ stem cells promote healing of diabetic foot ulcers in rats. Interact Cardiovasc Thorac Surg. 2012; 14:288–293.


26. Roedersheimer M, Nijmeh H, Burns N, Sidiakova AA, Stenmark KR, Gerasimovskaya EV. Complementary effects of extracellular nucleotides and platelet-derived extracts on angiogenesis of vasa vasorum endothelial cells in vitro and subcutaneous Matrigel plugs in vivo. Vasc Cell. 2011; 3:4.


27. Ting AE, Mays RW, Frey MR, Hof WV, Medicetty S, Deans R. Therapeutic pathways of adult stem cell repair. Crit Rev Oncol Hematol. 2008; 65:81–93.

