Abstract
Background
S-methylmethionine sulfonium chloride was originally called vitamin U because of its inhibition of ulceration in the digestive system. Vitamin U is ubiquitously expressed in the tissues of flowering plants, and while there have been reports on its hypolipidemic effect, its precise function remains unknown.
Objective
This study was designed to evaluate the anti-obesity effect of vitamin U in 3T3-L1 pre-adipocyte cell lines.
Methods
We cultured the pre-adipocyte cell line 3T3L1 to overconfluency and then added fat differentiation-inducing media (dexamethasone, IBMX [isobutylmethylxanthine], insulin, indomethacin) and different concentrations (10, 50, 70, 90, 100 mM) of vitamin U. Then, we evaluated changes in the levels of triglycerides (TGs), glycerol-3-phosphate dehydrogenase (G3PDH), AMP-activated protein kinase (AMPK), adipocyte-specific markers (peroxisome proliferator-activated receptor γ [PPAR-γ], CCAAT/enhancer-binding protein α [C/EBP-α], adipocyte differentiation and determination factor 1 [ADD-1], adipsin, fatty acid synthase, lipoprotein lipase) and apoptosis-related signals (Bcl-2, Bax).
Results
There was a gradual decrease in the level of TGs, C/EBP-α, PPAR-γ, adipsin, ADD-1 and GPDH activity with increasing concentrations of vitamin U. In contrast, we observed a significant increase in AMPK activity with increasing levels of vitamin U. The decrease in bcl-2 and increase in Bax observed with increasing concentrations of vitamin U in the media were not statistically significant.
Adipose tissue serves as an energy storage depot to maintain lipid homeostasis, thereby promoting the survival of the human body. Obesity occurs when the adipose tissue is overloaded with high-energy nutrients without subsequent expenditure1.
Because adipocytes play a critical role in energy balance through the storage of triglycerides (TGs) or the release of free fatty acids in response to changes in energy demands, understanding the molecular mechanisms of adipocyte differentiation may provide clues for the development of strategies for the prevention and treatment of obesity. The mechanisms of adipocyte differentiation have been extensively studied in preadipocyte culture systems. Characterization of the regulatory regions of adipose-specific genes has led to the identification of key transcription factors in the complex transcriptional cascade that occurs during adipocyte differentiation2. These factors include peroxisome proliferator-activated receptor γ (PPAR-γ)3, CCAAT/enhancer binding protein (C/EBP)4,5, adipocyte differentiation and determination factor 1 (ADD-1)6, fatty acid synthase (FAS) and lipoprotein lipase (LPL)7.
In addition to playing a central role in the storage of lipids, adipose tissue secretes numerous bioactive substances called adipokines that contribute to cell proliferation as well as the differentiation of preadipocytes into adipocytes. These substances include tumor necrosis factor-α, adiponectin, adipsin, and interleukin-6 (IL-6), which also regulate the inflammatory response8-14.
Recently, a number of natural compounds, such as herbal drugs and flavonoids, have received considerable attention for their abilities to regulate adipogenesis. S-methylmethionine sulphonium chloride is abundant in the tissues of flowering plants, especially in raw cabbage, and has also been called vitamin U (from the Latin 'ulcus' meaning sore or ulcer15), although its classification as a vitamin has not yet been accepted16. Gessler et al.17 reported that vitamin U can decrease the level of lipid peroxidation and inhibit monoamine oxidase activity. Also, they reported that vitamin U has a hypolipidemic effect.
Our study was conducted to determine whether vitamin U can modulate differentiation in 3T3-L1 adipocytes. The involvement of glycerol-3-phosphate dehydrogenase (G3PDH), AMP-activated protein kinase (AMPK) and adipocyte-specific markers (PPAR-γ, C/EBP-α, ADD-1, adipsin, FAS, and LPL) were evaluated.
3T3-L1 cells and vitamin U were purchased from the Korea Cell Line Bank (Seoul, Korea) and TCI Corp. (Tokyo, Japan), respectively. 3T3-L1 preadipocytes were incubated at a density of 8,000 cells/cm2 (10 cm2 dish) and grown in Dulbecco's modified Eagle's medium (DMEM) containing 10% fetal bovine serum (FBS) at 37℃ in 5% CO2 in air. Confluent preadipocytes were induced to differentiate with inducing medium containing 500 µM IBMX (isobutylmethylxanthine), 1 µM dexamethasone, 100 µM indomethacin and 10 µg/ml insulin. To test the effect of vitamin U on inhibition of adipocyte differentiation, we treated cells with vitamin U (10, 50, 70, or 100 mM) at day 0. To test the effect of vitamin U on fat in adipocytes, we changed the medium to DMEM (with 10% FBS) on day 6 after the induction of adipocyte differentiation and then treated the cells with vitamin U at different concentrations for seven days. Cultures were re-fed every 2-3 days to allow 90% of the cells to reach full differentiation before they were chemically treated. Chemicals were freshly diluted in adipocyte medium before treatment. Cells were washed with fresh adipocyte medium, re-fed with medium containing vitamin U, and then incubated at 37℃ in 5% CO2 in air before analysis.
3T3-L1 cells were treated with lipopolysaccharide (100 ng), interferon-γ (10 ng) and different concentrations of S-methylmethionine sulfonium chloride (50, 70, 90, 100 mM). After cells were incubated for 24 hr, MTT (5 mg/ml) was added. Then, the supernatant was discarded, dimethyl sulfoxide was added to the cells and formazan was formed via MTT reduction. Cell viability was measured using an ELISA at 570 nm.
The formation of oil droplets in cells was analyzed using Oil Red O staining as follows. After removal of culture medium, cells were washed twice with phosphate-buffered saline (PBS) and then fixed for at least one hour with pre-chilled 10% formaldehyde in PBS. Cells were stained with 0.7% Oil Red O solution for two hours, followed by three washes with PBS. Cells were maintained in water and photographed. Fat droplets in the adipocytes were stained red, and the Oil Red O stain was eluted with isopropanol and was quantified by measuring the optical density at 515 nm.
3T3-L1 adipocytes that were treated as described above were washed with PBS, harvested into 25 mM Tris buffer (pH 7.5) containing 1 mM ethylenediaminetetraacetic acid (EDTA), and lysed via brief sonication. We quantified TG levels in the lysates using the LabAssay™ Triglyceride kit (Wako Pure Chemical Industries. Ltd., Osaka, Japan).
After removal of the culture medium, cells were washed twice with PBS and lysed in enzyme extraction buffer. Immediately after the extraction, we added an equal volume of dilution buffer supplemented with 2-mercaptoethanol to the sample to prepare a two-fold diluent. We dispensed the substrate solution into each 96-well plate and preincubated the plate at 30℃. We then added 25 µl of a diluted sample and agitated the plate and determined the decrease in absorbance at 340 nm at 30℃.
Mouse adipocyte-specific markers (PPAR-γ, C/EBP-α, ADD-1, adipsin, FAS, LPL) were quantitatively measured using an RNeasy Lipid mini kit (Qiagen, Austin, TX, USA). The primers and probe sets were ordered from Bioneer Corp. (Daejeon, Korea) and were used according to the manufacturer's instructions. The probe and primer sequences are shown in Table 1.
Cells were incubated in a humidified 5% CO2 incubator. They were collected and lysed in ice-cold lysis buffer (20 mM Tris-HCl (pH 7.4), 2 mM EDTA, 500 µM sodium orthovanadate, 1% Triton X-100, 0.1% SDS, 10 mM NaF, 10 µg/ml leupeptin and 1 mM PMSF). The protein concentration was estimated with the Bio-Rad DC protein assay (Bio-Rad Laboratories, Hercules, CA, USA) using bovine serum albumin as a standard. Total protein (50~60 µg) was separated using sodium dodecyl sulfate-polyacrylamide gel electrophoresis (SDS-PAGE) in a 10% polyacrylamide gel. The proteins in the gel were transferred to a nitrocellulose membrane. The membrane was blocked with 5% skim milk in PBST (0.1% v/v Tween-20 in PBS, pH 7.2) for 1 h. Membranes were incubated with primary antibody at 4℃ overnight and then with a secondary antibody for 1 h. Membranes were washed in PBST for ten min, three times between each step. The signal was detected using the Amersham ECL system (Amersham-Pharmacia Biotech, Arlington Heights, IL, USA). The relative expressions of PPAR-γ and AMPK in 3T3-L1 adipocytes were quantified densitometrically using Image J software and were calculated according to the reference bands of β-actin.
3T3-L1 cell viability after treatment with different concentrations of vitamin U was measured using the MTT assay. The results showed that cell viability decreased slightly as the concentration of vitamin U increased (Fig. 1). However, the differences in cell viability were not statistically significant compared with that of the controls.
The effects of vitamin U on adipocyte differentiation were evaluated using Oil Red O staining and through the analysis of cellular TG content and G3PDH activity. Oil droplets were abundant in differentiated adipocytes, and the number of oil droplets was significantly lower than that in the untreated control cells (Fig. 2A, B). Consistent with the appearance of the formed oil droplets, vitamin U significantly reduced cellular TG levels in a concentration-dependent manner (Fig. 2C). We found that vitamin U significantly inhibited intracellular G3PDH (Fig. 2D).
To study the mechanisms by which vitamin U inhibits adipocyte differentiation, we assessed the effects of vitamin U on the expression of PPAR-γ, C/EBP-α, ADD-1, adipsin, FAS and LPL. The mRNA levels of C/EBP-α, ADD-1 and adipsin were decreased, and both the mRNA and protein levels of PPAR-γ were substantially decreased by vitamin U in a dose dependent manner. Vitamin U did not significantly block the induction of LPL or FAS (Fig. 3).
AMPK activation was examined using western blot analysis. AMPK phosphorylation increased with vitamin U treatment in a dose-dependent manner during adipogenic differentiation (Fig. 4).
This study suggests that vitamin U inhibits adipocyte differentiation via down-regulation of adipogenic factors. We investigated the effects of vitamin U on the inhibition of intracellular TG and G3PDH activities, marker enzymes of adipogenesis, in 3T3-L1 adipocytes. Fasting induces conversion of glycerol into TG through an induction of several hepatic enzymes such as G3PDH and glycerol kinase. Tomiyama et al.18 indicated that the expression of G3PDH is induced several fold upon conversion of preadipocytes to adipocytes, which is the predominant substrate for TG synthesis in adipose tissue. Our data indicated that the exposure of 3T3-L1 adipocytes to vitamin U results in lower levels of intracellular TGs and G3PDH than did other compounds tested. Adipose tissue is now known to produce and secrete PPAR-γ, C/EBP-α, ADD-1 and adipsin, which have roles in adipocyte differentiation because they are transcriptional factors for numerous genes3-6,9. The present experiment indicated that vitamin U treatment inhibited the mRNA expression levels of PPAR-γ, C/EBP-α, ADD-1 and adipsin, which demonstrates that adipogenesis is inhibited by the upstream transcriptional factor cascade. There were no noticeable changes in mRNA levels of LPL or FAS, which indicates that vitamin U does not affect adipocyte function itself.
Here, we present evidence that vitamin U inhibits adipocyte differentiation through the activation of AMPK. AMPK is a well-known metabolic master switch, activated by various stimuli, including exercise, hypoxia and reactive oxygen species19. Once activated, AMPK blocks anabolic pathways and promotes catabolic pathways, protecting the cell from various stress stimuli20. Hwang et al.21 suggested that several naturally occurring compounds, such as genistein, EGCG and capsaicin, activate AMPK in a dose-dependent manner, leading to the inhibition of adipocyte differentiation.
Although we found that AMPK and adipocyte differentiation factors (PPAR-γ, C/EBP-α, ADD-1 and adipsin) are involved in the antiobesity effects exerted by vitamin U, no direct correlation was found between AMPK and the signaling pathways of other adipocyte differentiation factors. Therefore, possible connections between AMPK and the signaling pathways of other adipocyte differentiation factors should be investigated in future studies.
In the present study, we elucidated the inhibitory effects of vitamin U on 3T3-L1 adipocytes, as indicated by decreases in intracellular TG content and G3PDH activity. The inhibitory effect of vitamin U appears to be mediated by the down-regulated expressions of adipogenic transcription factors (PPAR-γ, C/EBP-α and ADD-1) and adipocyte-specific proteins (adipsin) and the upregulated expression of AMPK. Since obesity is a social issue and a well-established risk factor for cardiovascular disease and diabetes, our study suggests that it may be worthwhile to determine if vitamin U has an inhibitory effect on adipogenesis in vivo.
Figures and Tables
Fig. 1
Cell viability of vitamin U at different concentrations in 3T3-L1 cell lines. The results show a slight decrease in viability with higher concentrations of vitamin U. However, no statistically significant differences were observed.
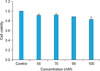
Fig. 2
Effect of vitamin U on the inhibition of adipocyte differentiation in Oil Red O staining, triglyceride (TG) assay and glycerol-3-phosphate dehydrogenase (G3PDH) activity assay. Oil droplets were abundant in differentiated adipocytes, and the number of oil droplets decreased with vitamin U treatment in a concentration-dependent manner (A, B). Consistent with the appearance of formed oil droplets, vitamin U significantly reduced cellular TG levels in a concentration-dependent manner (C). We found that vitamin U resulted in a significant inhibition of intracellular G3PDH (D). *Significant at p<0.05. ND: non-differentiated, NT: non-treated, Vit U: vitamin U, OD: optical density, G3PDH: glycerol-3-phosphate dehydrogenase.
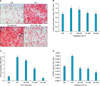
Fig. 3
Effects of vitamin U on the expressions of PPAR-γ, C/EBP-α, ADD-1, adipsin, FAS and LPL in 3T3-L1 adipocytes. The mRNA levels of C/EBP-α, ADD-1 and adipsin decreased, and both the mRNA and protein levels of PPAR-γ remarkably decreased with vitamin U treatment (A, B). DM: differentiated media, Vit U: vitamin U, PPAR-γ: peroxisome proliferator-activated receptor γ, C/EBP-α: CCAAT/enhancer binding protein α, FAS: fatty acid synthase, ADD-1: adipocyte differentiation and determination factor 1, LPL: lipoprotein lipase.
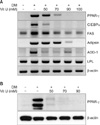
Fig. 4
Effect of vitamin U on activation of AMP-activated protein kinase (AMPK) detected using western blotting. Protein levels of phosphorylated AMPK increased with vitamin U treatment in a dose-dependent manner during adipogenic differentiation (A, B). DM: differentiated media, Vit U: vitamin U, AMPK: AMP-activated protein kinase, ND: non-differentiated, NT: non-treated. *Significant at p<0.05.
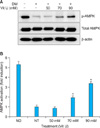
References
2. Spiegelman BM, Hu E, Kim JB, Brun R. PPAR gamma and the control of adipogenesis. Biochimie. 1997. 79:111–112.
3. Tontonoz P, Hu E, Spiegelman BM. Stimulation of adipogenesis in fibroblasts by PPAR gamma 2, a lipid-activated transcription factor. Cell. 1994. 79:1147–1156.


4. Umek RM, Friedman AD, McKnight SL. CCAAT-enhancer binding protein: a component of a differentiation switch. Science. 1991. 251:288–292.


5. Yeh WC, Cao Z, Classon M, McKnight SL. Cascade regulation of terminal adipocyte differentiation by three members of the C/EBP family of leucine zipper proteins. Genes Dev. 1995. 9:168–181.


6. Kim JB, Spiegelman BM. ADD1/SREBP1 promotes adipocyte differentiation and gene expression linked to fatty acid metabolism. Genes Dev. 1996. 10:1096–1107.


7. Ranganathan G, Unal R, Pokrovskaya I, Yao-Borengasser A, Phanavanh B, Lecka-Czernik B, et al. The lipogenic enzymes DGAT1, FAS, and LPL in adipose tissue: effects of obesity, insulin resistance, and TZD treatment. J Lipid Res. 2006. 47:2444–2450.


8. Friedman JM, Halaas JL. Leptin and the regulation of body weight in mammals. Nature. 1998. 395:763–770.


9. Rosen BS, Cook KS, Yaglom J, Groves DL, Volanakis JE, Damm D, et al. Adipsin and complement factor D activity: an immune-related defect in obesity. Science. 1989. 244:1483–1487.


10. Hotamisligil GS, Shargill NS, Spiegelman BM. Adipose expression of tumor necrosis factor-alpha: direct role in obesity-linked insulin resistance. Science. 1993. 259:87–91.


11. Ahima RS, Flier JS. Adipose tissue as an endocrine organ. Trends Endocrinol Metab. 2000. 11:327–332.


12. Sartipy P, Loskutoff DJ. Monocyte chemoattractant protein 1 in obesity and insulin resistance. Proc Natl Acad Sci USA. 2003. 100:7265–7270.
13. Hardie DG, Scott JW, Pan DA, Hudson ER. Management of cellular energy by the AMP-activated protein kinase system. FEBS Lett. 2003. 546:113–120.


14. Fryer LG, Carling D. AMP-activated protein kinase and the metabolic syndrome. Biochem Soc Trans. 2005. 33:362–366.


15. Kahn BB, Alquier T, Carling D, Hardie DG. AMP-activated protein kinase: ancient energy gauge provides clues to modern understanding of metabolism. Cell Metab. 2005. 1:15–25.


16. Roche-VITEC . Vitamin U. Pig News Info. 1990. 11:329–330.
17. Gessler NN, Kharchenko LI, Pavlovskaia TE, Bykhovskiĭ VIa. Radiation-protective effect of S-methylmethionine (vitamin U). Prikl Biokhim Mikrobiol. 1996. 32:666–668.
18. Tomiyama K, Nakata H, Sasa H, Arimura S, Nishio E, Watanabe Y. Wortmannin, a specific phosphatidylinositol 3-kinase inhibitor, inhibits adipocytic differentiation of 3T3-L1 cells. Biochem Biophys Res Commun. 1995. 212:263–269.


19. Towler MC, Hardie DG. AMP-activated protein kinase in metabolic control and insulin signaling. Circ Res. 2007. 100:328–341.

