Abstract
Background
Aquaporins (AQPs) are a family of water transporting proteins present in many mammalian epithelial and endothelial cell types. Among the AQPs, AQP3 is known to be a water/glycerol transporter expressed in human skin.
Objective
The relationship between the expression level of AQP3 and transpidermal water loss (TEWL) in the lesional and peri-lesional skin of psoriasis-affected patients, and skin hydration in the lesional and peri-lesional skin of psoriasis patients, was investigated.
Methods
The expression of AQP3 in psoriasis-affected and healthy control skin was determined using immunohistochemical and immunofluroscence staining. TEWL and skin hydration were measured using a Tewameter® TM210 (Courage & Khazaka, Cologne, Germany) and a Corneometer® CM 820 (Courage & Khazaka), respectively.
Results
AQP3 was mainly expressed in the plasma membrane of stratum corneum and the stratum spinosum in normal epidermis. Unlike the normal epidermis, AQP3 showed decreased expression in the lesional and peri-lesional epidermis of psoriasis. TEWL was increased, and skin hydration was decreased, in the lesional and peri-lesional skin of psoriasis patients, compared with the healthy control sample.
Psoriasis is a chronic disease of the skin, characterized by erythematous plaques with thick scales. Patients with psoriasis usually complain of skin dryness. Avoiding skin dryness by appropriate use emollients helps prolong therapy-free intervals and aids in the prevention of early recurrence1,2. Although there are many studies regarding the mechanisms involved in the skin dryness of atopic dermatitis, there are few studies relating specifically to psoriasis.
Aquaporins (AQPs) are proteins that facilitate entry of water into cells and, currently, 13 family members have been identified in mammals. Unlike other AQPs, AQP3 is expressed in epithelial tissues and works as a water/glycerol channel3. The functional role of AQP3 in human epidermis is an area of current research interest, as AQP3 is abundantly expressed in the basal and spinous layers of the epidermis.
Recent studies have shown that AQP3 is not only involved in water transport and hydration in the human epidermis but also in the regulation of keratinocyte proliferation, cell migration, and tumorigenesis4,5. Current studies of AQP3 related to atopic dermatitis focus on skin dryness. However, little is known about AQP3 expression in relation to skin hydration and transepidermal water loss (TEWL) in psoriatic skin lesions compared to healthy skin.
The aim of this study was to determine the expression of AQP3 in the epidermis of psoriasis and to investigate the relationship between AQP3 expression and skin hydration in psoriasis.
A total of 5 patients with psoriasis and 5 healthy volunteers participated in this study for immunohistochemical and immunofluorescent staining. A total of 19 patients with psoriasis and 10 healthy volunteers participated for measurement of TEWL and skin hydration. None of the patients had received phototherapy or topical treatments during the previous year. All skin samples were obtained under the written informed consent of donors, and the study was approved in accordance with the ethical committee approval process of Chungnam National University Hospital.
Normal human epidermal keratinocytes were cultured as previously reported6. Keratinocytes were grown in a keratinocyte-serum-free medium supplemented with bovine pituitary extract and recombinant human epidermal growth factor (Invitrogen, Grand Island, NY, USA).
Cells were lysed in Proprep solution (Intron). The total protein content was measured using a Bradford protein assay kit (Bio-Rad Laboratories, Hercules, CA, USA). Samples were run on sodium dodecyl sulfate-polyacrylamide gel, transferred onto nitrocellulose membranes, and incubated with appropriate antibodies. Blots were then incubated with peroxidase-conjugated secondary antibodies and visualized using enhanced chemiluminescence (Intron). The following primary antibodies were used in this study: AQP3, AQP9, filaggrin, involucrin (Santa Cruz Biotechnologies, Santa Cruz, CA, USA), and actin (Sigma, St. Louis, MO, USA).
Specimens were embedded in paraffin. Specimen sections were dewaxed, rehydrated, and washed three times in phosphate-buffered saline (PBS). After treatment with proteinase K (1 mg/ml), for 5 minutes at 37℃, sections were treated with H2O2 for 10 minutes at room temperature, blocked in 0.1% Tween-20 and 1% bovine serum albumin in PBS for 20 minutes, and were allowed to react with appropriate primary antibodies for 4 hours. Sections were incubated sequentially with secondary antibody (anti-mouse IgG-TR, Santa Cruz Biotechnologies) and were observed and imaged under fluorescent microscopy (Olympus IX71). The fluorescence intensity was analyzed using an image analyzer (i-solution TM, iMTechnology, Bucheon, Korea).
Normal human epidermal keratinocytes were cultured in serum free keratinocyte medium until 80% confluence, then starved in Keratinocyte-basal medium (KBM) for 1 day. Cells were then incubated in KBM containing 1 uCi/ml of [3H]glycerol with and without 1.2 mM Ca2+ for 0 minute, 10 minutes, 30 minutes, 60 minutes, 4 hours, 12 hours, 24 hours, and 48 hours. Reactions were stopped by washing twice with ice-cold PBS. Cells were subsequently solubilized in 1 N NaOH and aliquots of this extract were subjected to liquid scintillation counting. Counts obtained from duplicate samples at each time point for the two conditions were averaged and graphed, and a linear equation was determined for each condition. Correlation coefficients obtained were typically 0.99 or greater. Slopes determined from multiple experiments were averaged and analyzed statistically for significant differences between conditions7.
To determine the moisture level of the skin surface, we performed TEWL and skin hydration measurements. Results were used to provide information regarding the status of the permeability barrier under normal, experimentally perturbed, and diseased conditions. Published guidelines for the measurement of TEWL and skin hydration were followed. The condition of all subjects was first stabilized for 15 to 20 minutes, in a climate- and humidity-controlled room. The ambient temperature ranged between 21℃ and 24℃, with a mean relative humidity range of 39~50%. Two plaques on the arm were selected for study of lesional psoriatic skin, and normal appearing skin around the psoriatic plaques was selected as peri-lesional psoriatic skin. None of the selected psoriasis patients received phototherapy and topical treatment for at least the past year. The TEWL value was measured using a Tewameter® TM 210 (Courage & Khazaka, Cologne, Germany) and skin hydration was measure using a Corneometer® CM 820 (Courage & Khazaka)8,9.
Statistical analysis was performed using SPSS ver.12.0 (SPSS Inc., Chicago, IL, USA) for Windows. Results are presented as ± mean standard deviation. All experiments were repeated at least three times, with documented reproducibility. Data were analyzed for statistical significance, using an independent t-test and repeated measures ANOVA. p-values of <0.05 were considered significant.
To verify AQP3 protein expression in normal human epidermis, we performed immunofluorescent staining with an anti-AQP3 antibody. Fig. 1A shows abundant human epidermal AQP3 protein expression located in the keratinocyte plasma membrane of the stratum basale and stratum spinosum. AQP3 was weakly detected in the stratum granulosum and stratum corneum.
To confirm the expression level of the AQP3 protein in the epidermis, we performed an immune blot analysis for calcium-induced keratinocyte differentiation. The immune blot analysis showed that the AQP3 protein was expressed at the endogeneous level in normal epidermal keratinocytes, with a gradual decrease after 3 days. These results are similar to the immunofluorescent staining results (Fig. 2).
As AQP3 is known to participate in water/glycerol transport in the epidermis, the glycerol uptake in calcium differentiated keratinocytes was measured using radiolabeled [3H]glycerol for 48 hours. Fig. 1C shows a gradual increase in [3H]glycerol uptake in calcium differentiated keratinocytes and a significant reduction in glycerol uptake after 48 hours. The [3H]glycerol uptake reflects the results of AQP3 immunofluorescent and the immune blot analysis, which indicated AQP3 expression primarily in the malpighian layer. The glycerol uptake results illustrate the functional ability of AQP3, which is only expressed during early keratinocyte differentiation. These results are consistent with the location of AQP3 in the epidermis.
To identify AQP3 protein expression in the epidermis of psoriatic skin, we performed immunofluoresence staining with an anti-AQP3 antibody. Fig. 2 shows decreased expression of AQP3 in psoriatic lesional and peri-lesional skin, compared to the healthy control. The immunoreactivity patterns of AQP3 were different between the healthy control and psoriasis patients. The epidermis of healthy control patients showed AQP3 immunoreactivity localized largely in the plasma membrane of the malpighian layer, while psoriatic lesional and peri-lesional epidermis showed diffuse immunoreactivity localized to the cytoplasm rather than the plasma membrane.
For comparison of TEWL levels and skin hydration between psoriasis-affected (PA) and control patients, measurements were performed at least 5 times at each site. Measurements were made indoors, within a temperature range 21~24℃ and a relative humidity range of 39~50%. Psoriatic skin lesions were divided into the 3 groups of psoriasis lesional, psoriasis peri-lesional, and normal non-psoriasis skin.
Table 1 shows the comparison between PA and control patients. Both psoriatic lesional and peri-lesional skin showed decreased skin hydration and increased TEWL levels, compared to healthy control skin. The skin hydration and TEWL levels of normal non-psoriatic skin of psoriasis patients did not differ from that of the control group. Fig. 3 shows skin hydration and TEWL values in psoriasis patients, divided into the three groups. Psoriasis lesions showed statistically significant decreased skin hydration and increased TEWL levels, compared to peri-lesional and normal non-psoriatic skin (p<0.05). Psoriatic peri-lesional skin also showed statistically significant decreased skin hydration and increased TEWL levels, compared with normal non-psoriatic skin; this suggests a defect in skin moisture in peri-lesional psoriasis, even when the skin appears normal (p<0.05).
Psoriasis patients suffer not only from erythematous scaly plaques on the body but also from itching and dryness of the skin. Although there have been many studies investigating the mechanism of epidermal hyperproliferation and immune cell activation in psoriasis, only a few explore potential reasons that skin lesions involved psoriasis are so dry10.
After the identification of AQP1 in 1993, 11 additional AQPs have been identified in mammals. AQPs have been described in epithelial cells of the skin, kidney, gastrointestinal, and respiratory tracts. The exact function of AQPs in the skin is less thoroughly investigated than in other organs but, recently, AQPs have attracted attention because AQP3 is abundantly expressed in the skin.
The functional role of AQP3 in the skin had recently been demonstrated by the observed phenotype of AQP3 knockout mice, which showed a reduced glycerol content, water holding capacity in the epidermis, decreased skin elasticity, and delayed wound healing11,12. Moreover, a recent study showed decreased AQP3 expression in aging skin, suggesting that AQP3 is involved in the intrinsic aging of non sun-exposed human skin13.
AQP3 expression in the epidermis is localized in the keratinocyte plasma membrane in the stratum basale and stratum spinosum. The question arises of how AQP3 can function in stratum corneum hydration and TEWL when it is mainly located at the bottom of the epidermis. This is explained by a phenotype analysis of AQP3-deficient mice. In the study, high humidity and occlusion increased stratum corneum hydration in the wild-type, but not in AQP3 null mice. Skin conductance values for both the wild-type and AQP3 null mice were similar after exposure to 10% humidity, indicating that water transport via AQP3 is not a rate-limiting factor in TEWL10,11. Both water and glycerol permeability were significantly reduced in the epidermis of AQP3-knockout mice, with a normal concentration of glycerol in the dermis and the serum. Results suggest that there is reduced glycerol transport from the blood into the epidermis in the absence of AQP3, which is located in the malpighian layer of the epidermis, and that glycerol transport, rather than water transport, is responsible for stratum corneum hydration and TEWL, as glycerol is a natural moisturizing factor14.
The AQP3 protein immunoreactivity patterns are different in psoriatic lesional and peri-lesional skin, compared to the healthy control skin. Not only is the immunoreactivity of AQP3 remarkably decreased in the lesional and peri-lesional skin of psoriasis, but the AQP3 protein is diffusely expressed in the cytoplasm rather than in the plasma membrane, as it is in the control group15. AQP3 in psoriatic lesional and peri-lesional skin likely cannot function properly because the amount of the AQP3 protein is reduced; AQP3 is also functionally impaired because of an abnormal localization in keratinocytes. The measurement of skin hydration and TEWL in psoriasis and healthy control patients indicated relevance to in vitro results suggesting a decrease in AQP3 protein expression in lesional and peri-lesional psoriasis skin, resulting in decreased skin hydration and increased TEWL. The cause of decreased AQP3 protein expression in psoriasis is unknown. However, there are studies indicating that tumor necrosis factor (TNF)-α and TNF receptor-1 signalling decreases AQP3 protein expression in some cells16,17. Psoriasis is a Th1 cytokine dominant skin disorder, and TNF-α production is increased in keratinocytes and infiltrated immune cells. This may be related to decreased AQP3 protein expression in psoriasis. The retinoic acid, which we use orally and topically in treating PA skin, is known to increase AQP3 expression in normal human skin18. All-trans retinoic acid increased AQP3 mRNA and protein expression in normal human epidermal keratinocytes, indicating that retinoic acid increases AQP3 expression and enhances biological activity in human skin. We can hypothesize that using the retinoic acid in psoriasis can increase the expression of AQP3 in psoriasis epidermis, and AQP3 may play a functional role in improving psoriasis lesions, by regulating the keratinocyte proliferation and differentiation in psoriasis.
In summary, AQP3 is the predominant AQP in the human epidermis, and decreased expression of AQP3 was identified in skin biopsies from lesional and peri-lesional psoriatic skin, compared to a healthy control. Skin hydration and TEWL measurements showed decreased skin hydration and increased TEWL in the lesional and peri-lesional skin of psoriasis, compared to a healthy control, indicating the relevance and significance of the association with AQP3 expression in the epidermis. As AQP3 functions as a water/glycerol transporter in skin, AQP3 may be a key factor affecting decreased skin hydration and increased TEWL in psoriatic skin.
Figures and Tables
Fig. 1
(A) Immunolocalization of aquaporins 3 (AQP3) uisng immunohistochemisty in the human epidermis. AQP3 is expressed in the stratum basale and the stratum spinosum. (B) AQP3 expression in calcium-induced keratinocyte differentiation. AQP3 from cultured keratinocyte extracts of 20 or 30 µg was loaded onto sodium dodecyl sulfate polyacrylamide gel electrophoresis and was detected using a peptide polyclonal anti-AQP3 antibody. Involucrin and filaggrin were used as control makers for differentiation. (C) The [3H]glycerol uptake ability in calcium differentiated human keratinocytes. Near confluent keratinocytes were incubated in Keratinocyte-basal medium containing 1 uCi/ml of [3H]glycerol with and without 1.2 mM Ca2+ for 0 min, 10 min, 30 min, 60 min, 4 hr, 12 hr, 24 hr, and 48 hr. NHEK: normal human epidermal keratinocyte, CPM: counting per minute.
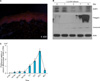
Fig. 2
Aquaporins 3 (AQP3) immunoreactivity in (A) healthy control, (B) psoriatic lesional and (C) peri-lesional skin. AQP3 protein expression is decreased and mislocalized in lesional and peri-lesional skin, showing a diffuse cytoplasmic pattern. (D) AQP3 staining intensity between healthy control and psoriasis. The fluorescence intensity was analyzed using an image analyzer (i-solution TM, iMTechnology, Bucheon, Korea) *p<0.05 vs. healthy control.
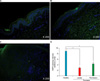
Fig. 3
(A) Differences in skin hydration among psoriatic lesional, peri-lesional, and normal non-psoriatic skin in psoriasis patients. Skin hydration was measured using a Corneometer® CM 820 (Courage & Khazaka, Cologne, Germany). (B) Transpidermal water loss (TEWL) differences among psoriatic lesional, peri-lesional, and normal non-psoriasis skin in psoriasis patients. TEWL was measured using a Tewameter® TM 210 (Courage & Khazaka).
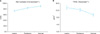
ACKNOWLEDGMENT
This study was financially supported by the research fund of Chungnam National University in 2008.
References
1. Gudjonsson JE, Elder ST. Wolff K, Goldsmith LA, Kats SI, Gilchrest BA, Paller AS, Leffell DJ, editors. Psoriasis. Fitzpatrick's dermatology in general medicine. 2008. 7th ed. New York: McGraw-Hill;169–193.


2. Hara-Chikuma M, Verkman AS. Roles of aquaporin-3 in the epidermis. J Invest Dermatol. 2008. 128:2145–2151.


4. Hara-Chikuma M, Verkman AS. Aquaporin-3 facilitates epidermal cell migration and proliferation during wound healing. J Mol Med (Berl). 2008. 86:221–231.


5. Hara-Chikuma M, Verkman AS. Prevention of skin tumorigenesis and impairment of epidermal cell proliferation by targeted aquaporin-3 gene disruption. Mol Cell Biol. 2008. 28:326–332.


6. Yoon HK, Sohn KC, Lee JS, Kim YJ, Bhak J, Yang JM, et al. Prediction and evaluation of protein-protein interaction in keratinocyte differentiation. Biochem Biophys Res Commun. 2008. 377:662–667.


7. Zheng X, Bollinger Bollag W. Aquaporin 3 colocates with phospholipase d2 in caveolin-rich membrane microdomains and is downregulated upon keratinocyte differentiation. J Invest Dermatol. 2003. 121:1487–1495.


8. Cho SH, Seo SJ, Hong CK. Expression of antimicrobial peptides according to changes of transepidermal water loss levels in patients with atopic dermatits. Ann Dermatol. 2007. 19:47–54.


9. Heinrich U, Koop U, Leneveu-Duchemin MC, Osterrieder K, Bielfeldt S, Chkarnat C, et al. Multicentre comparison of skin hydration in terms of physical-, physiological- and product-dependent parameters by the capacitive method (Corneometer CM 825). Int J Cosmet Sci. 2003. 25:45–53.


10. Suh DH, Kwon TE, Kim SD, Park SB, Kwon OS, Kim KH, et al. Changes of transepidermal water loss (TEWL) in psoriatic plaques during D-PUVA therapy. Ann Dermatol. 2001. 13:7–11.


11. Ma T, Hara M, Sougrat R, Verbavatz JM, Verkman AS. Impaired stratum corneum hydration in mice lacking epidermal water channel aquaporin-3. J Biol Chem. 2002. 277:17147–17153.


12. Hara M, Ma T, Verkman AS. Selectively reduced glycerol in skin of aquaporin-3-deficient mice may account for impaired skin hydration, elasticity, and barrier recovery. J Biol Chem. 2002. 277:46616–46621.


13. Li J, Tang H, Hu X, Chen M, Xie H. Aquaporin-3 gene and protein expression in sun-protected human skin decreases with skin ageing. Australas J Dermatol. 2010. 51:106–112.


14. Hara-Chikuma M, Verkman AS. Aquaporin-3 functions as a glycerol transporter in mammalian skin. Biol Cell. 2005. 97:479–486.


15. Voss KE, Bollag RJ, Fussell N, By C, Sheehan DJ, Bollag WB. Abnormal aquaporin-3 protein expression in hyperproliferative skin disorders. Arch Dermatol Res. 2011. 303:591–600.


16. Horie I, Maeda M, Yokoyama S, Hisatsune A, Katsuki H, Miyata T, et al. Tumor necrosis factor-alpha decreases aquaporin-3 expression in DJM-1 keratinocytes. Biochem Biophys Res Commun. 2009. 387:564–568.

