Abstract
Background
The extracellular matrix (ECM) produced by dermal fibroblasts supports skin structure, and degradation and/or reduced production of ECM are the main causes of wrinkle formation.
Objective
The aim of this study was to identify the active ingredient that enhances ECM production in dermal fibroblasts.
Methods
Polarity-based fractionation was used to isolate the active ingredient from natural extracts, and the effects of cedrol (isolated from Pterocarpus indicusirginia) on ECM production in cultured human dermal fibroblasts was investigated by reverse transcription-polymerase chain reaction, enzyme linked immunosorbent assay, and Western blot analysis.
Results
Cedrol accelerated fibroblast growth in a dose-dependent manner and increased the production of type 1 collagen and elastin. Phosphorylation of p42/44 extracellular signal-regulated kinase, p38 mitogen-activated protein kinase, and Akt was markedly increased by cedrol, indicating that enhanced ECM production is linked to activation of intracellular signaling cascades.
The extracellular matrix (ECM) has important functions in the maintenance of proper skin tensile strength and elasticity. ECM components are found in the dermis, in which mesenchyme-derived fibroblasts produce most of the ECM components, including several types of collagen molecules, polymeric glycosaminoglycans, and elastic fibers1. Of these, type 1 collagen is the most abundant structural protein in skin connective tissue and has a direct influence on skin tension. During the aging process, skin gradually loses mechanical strength and elasticity, which leads to the formation of wrinkles. This phenomenon is largely due to the collapse of dermal connective tissue, which coincides with the loss of fibroblast proliferation and reduced ECM production2. An abnormality in type I collagen synthesis has been suggested to cause an imbalance in ECM metabolism and the clinical changes observed in aged skin. Therefore, it has long been believed that enhancing fibroblast activity, in the context of ECM production, may help maintain skin texture.
In the course of screening stimulators for ECM production from natural compounds we found that cedrol, derived from Pterocarpus indicusirginia (Ja Dan Hyang), activates dermal fibroblasts. In a previous study, it was demonstrated that cedrol acts on the autonomic nervous system, producing a sedative effect3. Cedrol inhalation increases parasympathetic activity and decreases sympathetic activity in healthy human subjects. Cedrol also increases baroreceptor sensitivity in the same healthy human subjects4. However, it has never been reported whether cedrol affects fibroblast activity based on ECM production. In this study, we determined the effect of cedrol on ECM production in dermal fibroblasts and investigated the putative action mechanism of cedrol. We found that cedrol stimulates production of type I collagen via activation of mitogen-activated protein kinase (MAPK) signaling pathways. However, it has never been reported whether cedrol affects fibroblast activity based on ECM production.
In this study, we determined the effect of cedrol on ECM production in dermal fibroblasts and investigated the putative action mechanism of cedrol. We found that cedrol stimulates production of type I collagen via activation of mitogen-activated protein kinase (MAPK) signaling pathways.
Normal human skin samples were obtained from circumcisions in accordance with the ethical committee approval process of Chungnam National University Hospital. Specimens were briefly sterilized in 70% ethanol, minced, and then incubated in Dulbecco's Modified Eagle's Medium supplemented with 10% FBS and antibiotics (Gibco BRL, Rockville, MD, USA). Dermal fibroblasts were produced from explants after 5~7 days. At confluence, cells were routinely subcultured using a 1 : 4 split ratio. Cells were used between passages 4~15. For treatment with cedrol, approximately 1×106 cells were seeded on 100-mm culture dishes and grown to sub-confluence. Cells were serum-starved for 24 h and then treated with cedrol in serum-free medium.
We isolated cedrol from the lignum of Pterocarpus indicusirginia (Kyongdong Herbal Market, Seoul, Korea) using bioassay-directed fractionation. Air-dried P. indicusirginia (10 kg) was cut into pieces and extracted with five volumes of 80% ethanol for 5 days at room temperature. The extract was evaporated under reduced pressure conditions, then reconstituted with distilled water. The aqueous fraction was successively extracted with chloroform and n-butanol. The chloroform-dissolved fraction was then chromatographed on silica gel with a developing solution of n-hexane and chloroform. As a result, the chloroform-dissolved fraction was separated into 18 sub-fractions. Of these, the eighth sub-fraction was re-chromatographed on silica gel using a gradient developing solution (n-hexane : chloroform, 10 : 1~2 : 1), and then divided into eight additional sub-fractions. Of these, the fifth was recrystallized using a solvent of n-hexane and ethanol (Fig. 1A). The structure of the resultant crystal was determined using high performance liquid chromatography, nuclear magnetic resonance (NMR), and electrospray ionization mass spectrometry, and identified as cedrol (Fig. 1B). A total of 120 mg of crystalline cedrol was obtained using this fractionation protocol.
Fibroblast cells were seeded in a 60-mm culture dish and treated with 1 µCi of [3H]thymidine (Amersham, Buckinghamshire, UK) for a [3H]thymidine uptake assay. Following incubation for the indicated time points, cells were washed twice with phosphate buffered saline and incubated with 0.1 N NaOH at room temperature. Radioactivity in cell lysates was measured using a liquid scintillation counter.
An ELISA kit for type 1 pro-collagen was purchased from Takara Bio Inc. (Shiga, Japan). Levels of type 1 pro-collagen secreted from fibroblasts were quantified according to the manufacturer's recommended protocols. Measurements were repeated at least three times with independent cell batches.
Cells were lysed in Proprep solution (Intron, Daejeon, Korea). After vigorous pipetting, extracts were centrifuged for 15 min at 13,000 rpm. Total protein was measured using a Bradford protein assay kit (Bio-Rad Laboratories, Hercules, CA, USA). Samples were run on sodium dodecyl sulfate-polyacrylamide gels, transferred to nitrocellulose membranes, and incubated with appropriate antibodies overnight at 4℃ with gentle agitation. Blots were then incubated with peroxidase-conjugated secondary antibodies for 30 minutes at room temperature, and visualized using enhanced chemiluminescence (Intron, Scottsdale, AZ, USA). The following primary antibodies were used: collagen type 1 α1 and elastin (Santa Cruz Biotechnology, Santa Cruz, CA, USA); phospho-p38 MAPK, total-p38 MAPK, phospho-p42/44 extracellular signal-regulated kinase (ERK), total-p42/44 ERK, phospho-Akt, total-Akt, phospho-Smad 2, total-Smad 2, phospho-Smad 3, total-Smad 3 (Cell Signaling Technology, Danvers, MA, USA), and actin (Sigma, St. Louis, MO, USA).
Two µg of total RNA was reverse-transcribed using M-MLV reverse transcriptase (ELPIS Biotech, Daejeon, Korea). Aliquots of the RT mixture were subjected to PCR with specific primer sets (Table 1).
We used the polarity-based sequential fractionation method to screen the putative fibroblast activators. This process involved two step chromatography based on a silica matrix, and one step recrystalization (Fig. 1A). Results of 1H-NMR, 13C-NMR, and electrospray Ionization mass spectrometry showed that cedrol from the lingnum of P. indicusirginia was an active ingredient (Fig. 1B)5. The purity of cedrol was approximately 99%.
To investigate the effect of cedrol on fibroblast growth, we first performed a [3H]thymidine uptake assay. Dermal fibroblasts were treated with various concentrations of cedrol and incubated for 2 d. As shown in Fig. 2, cedrol increased [3H]thymidine uptake of fibroblasts in a dose-dependent manner.
Because the proliferative property of fibroblasts is frequently linked to ECM production (especially collagen) in fibroblasts6, we next determined the effect of cedrol on collagen and elastin production. An ELISA showed that cedrol increased secretion of type 1 procollagen in a dose-dependent manner, comparable to results using ascorbic acid (Fig. 3A). Concomitantly, RT-PCR and Western blot analyses demonstrated that cedrol induced transcription and an increase in the intracellular protein level of collagen type 1 α1 (Fig. 3B, C). Cedrol also increased elastin production (similar to collagen), suggesting that cedrol activates fibroblasts.
As the intracellular signaling cascades p42/44 ERK and p38 MAPK are implicated in the regulation of ECM production7-9, we investigated the effect of cedrol on MAPK activation. As shown in Fig. 4, cedrol treatment led to quick phosphorylation of p42/44 ERK and p38 MAPK. Additionally, the phosphorylation level of Akt, an important signaling molecule for cell survival, also increased following treatment with cedrol. As the Smad pathway is critical for collagen synthesis during the wound healing process10-12, we also investigated Smad protein activation. However, cedrol did not influence the Smad2/3phosphorylation, suggesting that cedrol affects ECM production via the intracellular MAPK signaling cascades.
We determined the effect of cedrol on collagen production using primary cultured dermal fibroblasts. Cedrol isolated from P. indicusirginia not only enhanced fibroblast proliferation but also increased the production of type 1 collagen and elastin. The cedrol effect was linked to activation of the ERK1/2, p38 MAPK, and Akt intracellular signaling pathways.
Dermis predominantly contains type I collagen synthesized from dermal fibroblasts as precursor molecules called procollagen. Type I collagen, the major component of ECM in skin, bone, and ligaments, is composed of glycine- and proline-rich two-α1 (I) and one-α2 chains, which are products of the COL1A1 and COL1A2 genes13. Because collagen has a long fibrous structure and constitutes a major part of the extracellular space in the dermis, its primary role is to support skin structure and provide tensile strength to the skin. In this regard, fibroblasts are primarily responsible for producing collagen and other ECM. This notion is clearly supported by the observation that collagen biosynthesis by fibroblasts is markedly diminished in aged persons2. Therefore, activation of cell proliferation and enhancement of collagen production to strengthen skin texture is an attractive approach for treating connective tissue-related skin problems, such as wrinkle formation.
Elastin, physiologically important in skin elasticity, is also secreted from skin fibroblasts as the soluble precursor tropoelastin, then subsequently cross-linked into insoluble elastin14. Therefore, our results indicate that cedrol can be used to strengthen skin texture and/or treat connective tissue-related skin problems.
A number of intracellular signal transduction pathways have been reported to play a role in the regulation of many cellular functions. For example, cell growth, differentiation, and apoptosis are all tightly regulated by the activation status of many intracellular signaling systems15-17. Gene expression is also regulated by an intracellular signaling system. Intracellular signaling cascades including MAPK, Akt, and Smad, are involved in the regulating collagen expression13. The primary role of MAPK has been previously recognized as procollagen synthesis8,18,19. We have demonstrated that cedrol significantly increases the phosphorylation of ERK1/2, p38 MAPK and Akt. Cedrol apparently acts as an intracellular signal modulator based on the association between collagen synthesis and MAPK activation. As cedrol has a two ring-structure and shows oily characteristics, cedrol probably penetrates the plasma membrane and directly affects intracellular signaling. However, the precise intracellular action mechanism of cedrol remains to be determined.
In summary, we have demonstrated that cedrol stimulates collagen and elastin production in dermal fibroblasts. Cedrol should be investigated for treatment of connective tissue-related skin problems as a co-modality together with first-line treatments.
Figures and Tables
Fig. 1
(A) Isolation of cedrol from Pterocarpus indicus. (B) Chemical structure of cedrol. Cedrol was isolated from the lignum of P. indicusirginia using bioassay-directed fractionation. After solvent fractionation, two applications of column chromatography followed by recrystallization were used to isolate the active compound. The purity of cedrol was confirmed to be ~99%, as determined by high performance liquid chromatography, nuclear magnetic resonance, and electrospray ionization mass spectrometry studies.
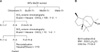
Fig. 2
Effect of cedrol on growth of dermal fibroblasts. Cells were treated with cedrol at the indicated concentrations for 2 d in the presence of [3H]thymidine. Radioactivity was measured using a liquid scintillation counter. Results are shown as a percentage of a control±the standard deviation (*p<0.05 vs. control).
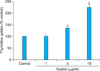
Fig. 3
Effect of cedrol on collagen and elastin production in dermal fibroblasts. (A) Cells were treated with cedrol at the indicated concentrations for 2 d. A conditioned medium was collected, and the amount of secreted procollagen type 1 was measured using an enzyme linked immunosorbent assay. Results are shown as a percentage of a control±the standard deviation (*p<0.05 vs. control). (B) Cellular proteins were harvested and the protein levels of collagen type 1 α1 and elastin were verified using Western blot analysis. (C) The expression levels of the COL1A1 and COL1A2 genes were determined using reverse transcription-polymerase chain reaction. CTL: control.
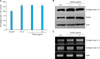
Fig. 4
Effect of cedrol on intracellular signaling pathways. Cells were treated with cedrol for the indicated times. Cellular proteins were prepared and phosphorylation of ERK, p38 MAPK, Akt, and Smad 2 and 3 was determined using Western blot analysis. CTL: control, ERK: extracellular signal-regulated kinase, MAPK: mitogen-activated protein kinase.
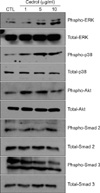
References
1. Pieraggi MT, Bouissou H, Angelier C, Uhart D, Magnol JP, Kokolo J. The fibroblast. Ann Pathol. 1985. 5:65–76.
2. Uitto J. Connective tissue biochemistry of the aging dermis. Age-related alterations in collagen and elastin. Dermatol Clin. 1986. 4:433–446.
3. Yada Y, Sadachi H, Nagashima Y, Suzuki T. Overseas survey of the effect of cedrol on the autonomic nervous system in three countries. J Physiol Anthropol. 2007. 26:349–354.


4. Umeno K, Hori E, Tsubota M, Shojaku H, Miwa T, Nagashima Y, et al. Effects of direct cedrol inhalation into the lower airway on autonomic nervous activity in totally laryngectomized subjects. Br J Clin Pharmacol. 2008. 65:188–196.


5. Joseph-Nathan P, Santillan RL, Gutiérrez A. Gutierrez, Carbon-13 NMR. Study of Cedrol, 6-Isocedrol, and α-Cedrene. J Nat Prod. 1984. 47:924–933.
6. Roh SS, Lee MH, Hwang YL, Song HH, Jin MH, Park SG, et al. Stimulation of the extracellular matrix production in dermal fibroblasts by velvet antler extract. Ann Dermatol. 2010. 22:173–179.


7. Ignotz RA, Massagué J. Transforming growth factor-beta stimulates the expression of fibronectin and collagen and their incorporation into the extracellular matrix. J Biol Chem. 1986. 261:4337–4345.


8. Lim IJ, Phan TT, Tan EK, Nguyen TT, Tran E, Longaker MT, et al. Synchronous activation of ERK and phosphatidylinositol 3-kinase pathways is required for collagen and extracellular matrix production in keloids. J Biol Chem. 2003. 278:40851–40858.


9. Lee DJ, Rosenfeldt H, Grinnell F. Activation of ERK and p38 MAP kinases in human fibroblasts during collagen matrix contraction. Exp Cell Res. 2000. 257:190–197.


11. Bhogal RK, Stoica CM, McGaha TL, Bona CA. Molecular aspects of regulation of collagen gene expression in fibrosis. J Clin Immunol. 2005. 25:592–603.


12. Chen SJ, Yuan W, Mori Y, Levenson A, Trojanowska M, Varga J. Stimulation of type I collagen transcription in human skin fibroblasts by TGF-beta: involvement of Smad 3. J Invest Dermatol. 1999. 112:49–57.


13. Ghosh AK. Factors involved in the regulation of type I collagen gene expression: implication in fibrosis. Exp Biol Med (Maywood). 2002. 227:301–314.


14. Sephel GC, Davidson JM. Elastin production in human skin fibroblast cultures and its decline with age. J Invest Dermatol. 1986. 86:279–285.


15. Neumann E, Gay RE, Gay S, Müller-Ladner U. Functional genomics of fibroblasts. Curr Opin Rheumatol. 2004. 16:238–245.


16. Wiedłocha A, Sørensen V. Signaling, internalization, and intracellular activity of fibroblast growth factor. Curr Top Microbiol Immunol. 2004. 286:45–79.
17. Chiquet M, Renedo AS, Huber F, Flück M. How do fibroblasts translate mechanical signals into changes in extracellular matrix production? Matrix Biol. 2003. 22:73–80.

