Abstract
Background
Although reactive oxygen species (ROS) have been produced in both mouse bone marrow-derived dendritic cells (DCs) and XS-106 DCs by contact sensitizers and irritants in previous studies, the generation of ROS in human monocyte-derived DCs (MoDCs) and their role in contact hypersensitivity (CHS) has yet to be elucidated.
Objective
The purpose of this study was to determine whether contact allergens and irritants induce ROS in MoDCs and, if so, to evaluate the role of contact allergen and irritant induced-ROS in MoDCs in CHS.
Methods
Production of ROS was measured by 5-(and-6)-chloromethyl-2',7'-dichlorodihydrofluorescein diacetate (CM-H2DCFDA) assay. Surface CD86 and HLA-DR molecules were detected by flow cytometry. Protein carbonylation was detected by Western blotting.
Results
ROS were produced by contact allergens such as dinitrochlorobenzene (DNCB) and thimerosal and the irritant benzalkonium chloride (BKC). DNCB-induced, but not BKC-induced, ROS increased surface CD86 and HLA-DR molecules on MoDCs and induced protein carbonylation. These changes were reduced in the presence of antioxidant N-acetyl cysteine.
Reactive oxygen species (ROS) are important players in host defenses against bacterial infection1,2, skin aging3,4, cancer5, and various chronic diseases6. However, little is known about the role of ROS in skin diseases7 and contact hypersensitivity (CHS). ROS are molecular oxygen derivatives that have various functions in cells8. Besides acting as direct bacterial killing mechanisms, ROS also stimulate the immune response through activation of signaling pathways, upregulation of surface co-stimulatory molecules, protein carbonylation, and secretion of proinflammatory cytokines such as IL-129. ROS induction and inactivation are tightly regulated by enzymatic and non-enzymatic antioxidant systems10. Interruption of this system can lead to increased amounts of ROS, especially secondary or toxic ROS that are potentially damaging to cellular macromolecules5,6.
Contact dermatitis is an inflammatory skin disorder caused by environmental skin-sensitizing substances and is classified into 2 types: allergic contact dermatitis (ACD) and irritant contact dermatitis (ICD). ACD is considered to be a cell-mediated, delayed-type hypersensitivity immune response11, whereas ICD is thought to be a non-immunological, local inflammatory reaction to chemicals. As mentioned above, ROS can be important in CHS; for this reason, the ROS-inducing potential of various contact allergens and irritants in DCs has been studied.
Antigen presenting cells play an essential role in the T cell-mediated immune response12,13. In the skin, there are different antigen presenting cells localized in the epidermis (Langerhans cells [LCs]) and dermis (dermal dendritic cells [DDCs]). However, after applying haptens to the epidermis of mice genetically engineered to be deficient in LCs, decreased CHS response was not observed14. Therefore, whether or not LCs are involved in the induction of CHS remains controversial. Recently DDCs were reported to be more important than LCs in CHS14. Monocyte-derived DCs (MoDCs) have similar characteristics to DDCs or interstitial DCs15. Therefore, MoDCs were used as the experimental model in the present study.
The purpose of this study was to determine whether ROS are induced by contact allergens and irritants in MoDCs and, if so, to evaluate the role of hapten or irritant induced-ROS in CHS.
Contact allergens, such as 2,4-dinitrochlorobenzene (DNCB), 2,4-dinitrofluorobenzene (DNFB), 2,4,6-trinitrobenzene sulfonic acid (TNBS) and thimerosal (Thim); irritants, such as benzalkonium chloride (BKC) and sodium dodecyl sulfate (SDS); N,N1-dicyclohexylcarbodiimide (DCCD), an ATP synthase inhibitor (ASI); and the antioxidant N-acetyl cysteine (NAC), were purchased from Sigma-Aldrich (Sigma, St. Louis, MO, USA). 5-(and-6)-chloromethyl-2',7'-dichlorodihydrofluorescein diacetate (CM-H2DCFDA) was obtained from Molecular Probes (Eugene, OR, USA). X-VIVO 15 (BioWhittaker Cambrex, Walkersville, MD, USA), and recombinant human (rh) GM-CSF (Novartis Pharma GmbH, Nuremberg, Germany) and rhIL-4 (Strathmann Biotech GMBH, Hamburg, Germany) were purchased for MoDC generation. Immature MoDCs were treated with sublethal concentrations of chemicals with or without pretreatment with ROS inhibitors such as NAC and catalase. NAC was dissolved in warm phosphate buffered saline (PBS) immediately prior to pre-treatment. 30µM of DCCD, an ASI, was used as a positive control to induce ROS production from MoDCs. It was incubated with MoDCs for 30 min.
MoDCs were generated as previously described16,17. Briefly, mononuclear cells were separated by a density gradient using Ficoll-Paque PLUS (GE Healthcare Bio-Sciences AB, Uppsala, Sweden) and monocyte cells were isolated by the plastic adherence method, incubating the mononuclear cells in 3% X-VIVO for 40 min and washing vigorously with PBS to remove non-adherent lymphocytes. Isolated monocytes were cultured in 10% plasma in X-VIVO 15 for 6 days with 800 U/ml of rhGM-CSF and 1,000 U/ml of rhIL-4. On alternate days, 1 ml of culture medium was removed and fresh medium containing rhGM-CSF and rhIL-4 was added.
After treatment with sublethal concentrations of chemicals, MoDCs were harvested, washed twice with prewarmed PBS, and resuspended in 50µl PBS. The cells were stained with 3µM CM-H2DCFDA at 37℃ for 15 min and washed twice with PBS. Before analysis, 10µl propodium iodide (PI) (100µg/ml) was added to exclude dead cells and to control toxicity of chemicals. Samples were analyzed by flow cytometry (FACSCalibur, Becton Dickinson, Mountain View, CA, USA) for fluorescence signals within the PI- populations.
To determine the phenotypes of DCs, the following surface molecules were analyzed by flow cytometry. Mouse monoclonal fluorescence-conjugated antibodies (mAbs) against CD86 (FITC), HLA-DR (FITC), CD80 (PE), and CD83 (PE) (BD Pharmingen, San Diego, CA, USA) and the appropriate isotype controls were used. After treatment with haptens or irritants for 24 and 48 h, MoDCs were harvested, washed, and stained with the above mAbs. Samples were analyzed by flow cytometry.
Culture supernatants of MoDCs were analyzed by enzyme-linked immunosorbent assay (ELISA), according to the manufacturer's provided protocols. IL-1β, TNFα, and IL-12p70 cytokines were purchased from R&D Systems Inc. (Minneapolis, MN, USA) and ELISA was performed according to the manufacturer's protocol.
The cytoplasmic and nuclear proteins were extracted using ProteoJET Cytoplasmic and Nuclear Protein Extraction Kit (Fermentas Inc, Baltimore, MD, USA) according to the manufacturer's protocol. The protein concentrations were determined using BCA assay. 30µg of proteins were loaded by 10% SDS-PAGE and electrotransferred to polyvinylidene difluoride membranes (Millipore Corporation, Bedford, MA, USA). The membranes were incubated with anti-DNP (Cell Signaling, Beverley, MA, USA) antibodies at -4℃ overnight. They were then washed, incubated further with HRP-conjugated, anti-rabbit IgG (1/1,000 dilution), and detected using an ECL detection system (Amersham Biosciences, Piscataway, NJ, USA). An anti-actin antibody (Sigma) was used as a loading control at 1:5,000.
Production of ROS and expression of surface molecules were described through mean fluorescence intensity using flow cytometry. Data were analyzed using a Student's t-test. For statistical analyses, we used SPSS version 13.0 (SPSS Inc., Chicago, IL, USA). p-values <0.05 were considered to be significant.
After determining the optimal concentrations of chemicals to use (Table 1), immature MoDCs were exposed to contact allergens and irritants to examine ROS induction. Of the contact sensitizers studied, DNCB, TNBS, Thim, and DNFB were found to induce ROS. DNCB, TNBS, Thim and DNFB increased ROS production 3.8-fold, 2.9-fold, 3.3-fold, and 1.5-fold, respectively, compared to the control. Of these, the relative increase of ROS production by DNCB, Thim, and DNFB were statistically significant (p-values were 0.031, 0.029, and 0.034, respectively). ROS production after treatment with HQ, cobalt, and nickel was not detectable (data not shown). Of the irritants used, BKC and SDS induced ROS significantly (the relative ROS increase with BKC was 2.6-fold and that with SDS was 2.0-fold; p-values were 0.028 and 0.013, respectively) (Fig. 1).
MoDCs were treated with DNCB and BKC and carbonylated proteins were detected using anti-DNP antibody by Western blotting. Although the allergen DNCB and the irritant BKC both induced ROS similarly, only DNCB exposure was associated with protein carbonylation (Fig. 2).
To demonstrate that immature MoDCs treated with DNCB and BKC have enhanced expression of CD80, CD86, CD83, and HLA-DR, we used flow cytometry analysis. CD86 and HLA-DR expression on MoDCs cultured with DNCB were increased 2.5-fold and 1.2-fold, respectively (with p-values of 0.018 and 0.033, respectively), compared to that of the control, which was statistically significant (Fig. 3A, C and Table 2A), while CD80 and CD83 expression was not augmented by DNCB. In contrast, irritant BKC did not induce the expression of all surface molecules on MoDCs (Fig. 3B, D).
After demonstrating the production of ROS from MoDCs cultured with DNCB and BKC, the effect of the antioxidant NAC on these ROS production was examined (Fig. 4A). Pre-treatment of MoDCs with 5 mM of NAC reduced the ROS production induced by DNCB significantly (the relative ROS increase with DNCB treatment was 3.8-fold while that with pre-treatment with NAC plus DNCB treatment was 0.56-fold p≤0.0001). BKC-induced ROS production was decreased by pre-treatment with NAC (the relative ROS increase of BKC was 2.6-fold while that with pre-treatment with NAC plus BKC treatment was 0.70-fold).
To confirm that protein carbonylation is induced by ROS, we used antioxidant NAC, expecting that protein carbonylation would decrease as the amount of ROS decreased. After incubation of MoDCs treated with DNCB in the presence of 5 mM NAC, the carbonylated protein level was indeed found to be decreased (Fig. 4B).
To verify that DNCB-induced up-regulation of CD86 and HLA-DR is related to the generation of ROS, we examined these parameters with the addition of the antioxidant NAC. MoDCs were treated with DNCB in the presence or absence of NAC and cell surface molecules were analyzed by flow cytometry. Augmentation of CD86 and HLA-DR by DNCB was decreased by adding 10 mM NAC (Fig. 4C and Table 2B).
Allergen- and irritant-induced ROS may have a distinct role depending on their source. The source of ROS generated by each substance was examined and we found that contact allergen DNCB induced-ROS are reduced in the presence of diphenylene iodonium (DPI) (Fig. 5A) while the irritant BKC induced-ROS are decreased slightly by N-monomethyl-L-arginine (NMMA) (Fig. 5B and Table 3).
Cytokine secretion (IL-1β, IL-12p70, and TNFα) was assayed with ELISA in MoDCs treated by the contact allergen DNCB or the irritant BKC. TNF-α secretion in the DNCB- and BKC-treated MoDCs were increased by 1.8-fold and 2.7-fold respectively compared to the control, but these increments were not statistically significant. Other cytokines (IL-1β, IL-12p70) were not detectable in the DNCB- and BKC-treated MoDCs (Table 4).
Using the well-established mouse XS-106 DC line and mouse bone marrow-derived DCs, we previously demonstrated that contact allergens induce ROS, which in turn stimulate IL-12 cytokine secretion and the Th1 response during CHS9,18. We therefore sought to confirm the result in the human system. In the first part of this experiment, the optimal concentration of haptens and irritants, at which the percentage of live cells exceeded 90%, was found after incubation with these substances for 1 h and 24 h (Table 1). Subsequently, ROS levels were measured to determine whether these chemicals did in fact induce ROS in MoDCs, which was confirmed. The ASI, N,N1-DCCD, was used as a positive control and catalase was used as an enzymatic inhibitor of ROS. The results were similar to those of our previous study18. However, some of the contact sensitizers, such as nickel, did not generate ROS in amounts detectable by CM-H2DCFDA assay. Other studies have reported that both DNCB and nickel induce redox imbalances and further increase surface molecules on DCs19,20. The conflicting data can be explained by the usage of different ROS detection assays. Although there are several assays used to measure levels of ROS21-24, we chose CM-H2DCFDA as it is a widely-used, simple assay. CM-H2DCFDA is sensitive to oxidation by peroxynitrite (ONOO-) and hydrogen peroxide (H2O2) in the presence of cellular peroxidase and hydroxyl radicals (OH-). Unfortunately, it may have some disadvantages. For example, it is not suitable for measurement of nitric oxide (NO), hypochlorite (HOCl), or superoxide (O2-) in biological systems21. ROS detection by the CM-H2DCFDA method does not have selectivity; it reacts with many ROS and does not distinguish between the different types22. ROS is a general term given to a variety of ROS and radicals. It is possible that each different allergen and irritant may induce a distinct type of ROS with different types having different roles in contact dermatitis.
After confirming the generation of ROS, we sought to determine the distinct effects of contact sensitizer induced- and irritant induced-ROS on DC activation. ROS seem to have two primary roles. First, ROS can act as second messengers that alter DC function during antigen presentation when induced in moderate concentrations. The signal may depend on the type of ROS; for example, weak oxidizing (moderately toxic) ROS such as hydrogen peroxide and superoxide may be important for organ development25, secondary signal transduction6,8 and immune system enhancement9 while highly toxic ROS such as peroxynitrite, hydroxyl radicals, and hypochlorate may be transducers of cell death signaling pathways26. Secondly, ROS may induce oxidative stress, which arises when the concentration of ROS exceeds the cell's ability to neutralize them and repair cellular damage. Ultimately, this results in the widespread oxidation of biomolecules. ROS are mainly generated by mitochondrial electron transport systems I and III (ETS-I and III), nicotinamide adenine dinucleotide phosphate (NADPH) oxidase, xanthine oxidase, nitric oxide synthase (NOS), cyclooxygenase (COX), and ribonucleotide reductase systems27. From the above results, it can be postulated that BKC-induced ROS may consist of nitric oxides or highly toxic peroxynitrite, while contact allergen induced-ROS may consist of hydrogen peroxide or superoxide, which are derived from the NOX2 pathway.
ROS can cause a number of protein modifications, such as protein carbonylation and modification of protein side chains2. Carbonylation results from direct oxidation of amino acids side chains. Since carbonylation involves the addition of a relatively large and reactive group to the peptide backbone, it can have a variety of effects on the protein's properties, including covalent intermolecular cross-linking and cleavage to yield lower molecular weight species2,28. Our study revealed that contact allergen DNCB was associated with protein carbonylation detectable by DNP immunoblotting while BKC was not. This suggests that protein carbonylation might be involved in the pathogenesis of CHS. This result is similar to the results of our previous study9. Incubation with the thiol-containing antioxidant NAC reduced the amount of carbonylated protein. Although the decrease in the carbonylated protein level was small, this finding suggests that protein carbonylation may be caused by ROS.
Antioxidants and ROS scavengers appear to have anti-inflammatory effects, likely by counteracting the effects of ROS. Therefore, we examined the levels of hapten-induced ROS in the presence of antioxidants. Although several antioxidants, such as Vitamins E and A, alpha-lipoic acid, and glutathione (GSH), did not reduce the ROS level (data not shown), incubation with NAC decreased both DNCB- and BKC-induced ROS. GSH is a key component in protection against oxidative stress because it is a co-factor of several antioxidant enzymes and scavenges OH- and O- directly. It also is able to regenerate other important antioxidants such as vitamins C and E back to their active forms29. Pretreatment with GSH alone did not affect the ROS level; however, its precursor NAC did reduce the ROS level. This may be due to the fact that high concentrations of reduced GSH in the cell interfere with the diffusion of GSH into cells. GSH is abundant in the cytoplasm (1~11 mM), in the mitochondria (3~11 mM), and in the nucleus (5~15 mM)30.
Surface molecule expression varied depending on the chemicals that were applied to cells. Expression of CD86, ICAM (CD54) and HLA-DR molecules on MoDCs was upregulated by contact allergens31 while the amount of CD80 and CD83 molecules was not increased. Our study demonstrated that these upregulations were dependent on the ROS. Rutault et al.20 reported that hydrogen peroxide induces upregulation of several DC surface molecules, such as MHC class II and co-stimulatory molecules including CD40 and CD86, and that this enhancement is blocked by NAC. These results confirm the results of our previous studies9,18 which showed that ROS produced by contact allergen increase surface molecules and produce cytokines such as IL-12p70 in mouse XS-106 DCs cell lines9 and mouse bone-marrow derived DCs18. This study also proves that the results obtained from the mouse system are similar to that of the human system. Irritant BKC did not cause an increase in any of the co-stimulatory molecules on MoDCs. Even though both DNCB and BKC produced ROS from MoDCs, the ROS produced by DNCB and BKC have different roles in the change of surface molecules of MoDCs. Therefore, it may be useful to further characterize the types of ROS produced by DNCB and BKC in future studies. Furthermore, it may be necessary to use other detection methods besides the CM-H2DCFDA assay.
Among the cytokines (IL-1β, IL-12p70, and TNFα) involved in CHS, only TNF-α secretion was detected after treatment with DNCB and BKC in this study. Other groups have also reported the detection of TNF-α at mRNA level32 and small amounts of TNF-α at the protein level after treatment with DNCB33. Cytokines (IL-1β, IL-12p70) at the protein level in MoDCs were undetected both in the control and experimental groups in this study. Contact allergen and irritant alone may not be sufficient stimulus leading to the secretion of the biologically active form of IL-1β, but when combined with other molecules such as LPS or TNF-α, they can further enhance the production of IL-1β34. IL-12p70 was easily secreted by mouse XS-106 DCs after incubation with contact allergen9 in our previous study. We could not detect, however, any trace of IL-12p70 in the control and experimental groups in this experiment. This may be explained through the fact that MoDCs, different from the mouse XS106 DCs line, do not typically secrete IL-12p70.
The mere fact that ROS, in the process of antigen presentation, play a role in the activation of DCs and their interactions with T cells35, together with the fact that extracellular superoxide dismutase impairs LC migration and therefore suppresses mouse CHS36, confirms the involvement of ROS in CHS. Our study has further demonstrated the involvement of ROS in CHS through hapten-induced ROS production in human MoDCs, similar to that of the involvement of ROS in mouse CHS, which was shown through hapten-induced ROS production in mouse XS 106 DC line9 and mouse bone marrow DCs18.
In conclusion, our results suggest that DNCB and BKC may induce different ROS in MoDCs. DNCB-induced ROS may induce the CHS response by activating DC.
Figures and Tables
![]() | Fig. 1(A) Monocyte-derived dendritic cells (MoDCs) produce reactive oxygen species (ROS) after incubation with various chemicals including allergens and irritants. Immature MoDCs were incubated for 1 h with sublethal concentration of allergens, such as (a) dinitrochlorobenzene (DNCB), (b) thimerosal (Thim), (c) dinitrofluorobenzene (DNFB) and (d) trinitrobenzene sulfonic acid (TNBS); and irritants, such as (e) benzalkonium chloride (BKC) and (f) sodium dodecyl sulfate (SDS). ROS production was detected by CM-H2DCFDA assay. (A) Filled line represents control cells without CM-H2DCFDA staining; dotted line indicates physiologic ROS level in the cells (DCFDA staining only) without treatment; solid line indicates ATP synthase inhibitor (ASI)-treated cells as a positive control; solid thick line indicates ROS level induced by chemicals. Contact allergens DNCB, TNBS, Thim and DNFB increased ROS production 3.8-fold, 2.9-fold, 3.3-fold, and 1.5-fold, respectively, compared to the control. Irritants BKC and SDS induced ROS significantly (2.6-fold and 2.0-fold, respectively). (B) Relative increase of ROS after incubation with various chemicals including allergens and irritants. The relative increase in ROS was calculated by comparing the mean fluorescence intensity (MFI) of CM-H2DCFDA stained-treatment groups to that of the control group. Of these, the relative increases of ROS production by DNCB, Thim, and DNFB were statistically significant (p-values were 0.031, 0.029, and 0.034, respectively). Of the irritants used, BKC and SDS induced ROS significantly (p-values were 0.028 and 0.013, respectively). |
![]() | Fig. 2Contact allergen-DNCB, but not irritant-BKC, induced protein carbonylation. Immature day 6 MoDCs were incubated with 20µm DNCB and 10µM BKC for 6 h. Carbonylated groups were detected by immunoblotting with an anti-DNP antibody. DNCB treated cells but not BKC treated cells showed increased carbonylation compared with the control. Actin is included as a loading control. These data are representative of two independent experiments. |
![]() | Fig. 3Augmentation of CD86 and HLA-DR expression on MoDCs by DNCB but not by BKC. MoDCs were treated with 10µM DNCB or 5µM BKC for 24 h and then analyzed by flow cytometry. (A, B) Thick solid line indicates CD86 expression on MoDCs after treatment with DNCB and BKC, respectively; solid narrow line indicates positive control (treatment with cytokine cocktail); filled line indicates untreated DCs; dotted line indicates DCs stained with CD86 only. (C, D) Thick solid line indicates HLA-DR expression on MoDCs after treatment with DNCB and BKC, respectively; solid narrow line indicates positive control, filled line indicates untreated DCs, and dotted line indicates DCs stained with HLA-DR only. CD86 and HLA-DR expression on MoDCs cultured with DNCB was increased 2.5-fold and 1.2-fold respectively; p-values were 0.018 and 0.033, respectively, compared to that of the control. |
![]() | Fig. 4(A) Hapten and irritant-induced ROS diminished by N-acetyl L-cystein. MoDCs were pre-treated with 5 mM NAC for 30 min and then treated with 30µM DNCB or 20µM BKC. After 1 h they were stained with 3 mM CM-H2DCFDA and analyzed by flow cytometry. The histogram of chemical-treated MoDCs in the presence or the absence of NAC is expressed as a thick solid line or solid line, whereas a filled line or a dotted line indicates histograms of untreated DCs or of DCs stained with CM-H2DCFDA, respectively. Pre-treatment of MoDCs with 5 mM of NAC reduced the ROS production induced by DNCB significantly (the relative ROS increase with DNCB treatment was 3.8-fold while that with pre-treatment with NAC plus DNCB treatment was 0.56-fold. p≤0.0001). BKC-induced ROS production was decreased by pre-treatment with NAC (the relative ROS increase with BKC treatment was 2.6-fold while that with pre-treatment with NAC plus BKC treatment was 0.70-fold). Each experiment was repeated three times and one representative result is shown. (B) These carbonylated proteins were decreased slightly by NAC. Immature day 6 MoDCs were incubated with 20µM DNCB or 10µM BKC in the presence or absence of 5 mM NAC for 30 min. After 6 h, equal amounts of whole cell protein extracts were loaded in SDS gel and carbonylated proteins were detected using DNP antibody by Western blotting. Allergen DNCB but not irritant BKC induced protein carbonylation and the resulting carbonylated protein was decreased slightly by NAC. (C) NAC suppressed the augmentation of CD86 and HLA-DR molecules on MoDCs that were induced by contact allergen DNCB. MoDCs were cultured with DNCB in the presence or absence of pre-treatment 5 mM NAC. After 24 h their surface molecules were analyzed by flow cytometry. Thick solid line indicates the histogram of chemical-treated MoDCs with NAC; narrow solid line indicates treatment without NAC; filled line indicates non-treatment; dotted line indicates treatment with CD86 antibody only. Augmentation of CD86 and HLA-DR by DNCB was decreased by adding 10 mM NAC. |
![]() | Fig. 5Inhibition of ROS generating pathway. (A) DNCB induced-ROS blocked by NADPH synthase inhibitor DPI. MoDCs were treated with 20µM DNCB for 1 h in the presence (thick solid line) or absence (solid narrow line) of DPI (a specific blocker of NADPH oxidase). Filled line indicates no staining, while dotted line indicates staining with CM-H2DCFDA only. (B) BKC induced-ROS blocked slightly by iNOS inhibitor NMMA. MoDC treated with 10µM BKC in the absence (solid narrow line) or presence (thick solid line) of NMMA. |
Table 1
The optimal concentrations of chemicals used
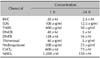
The concentrations of various chemicals at which the percentage of live monocyte-derived dendritic cells (Annexin Y/PI) exceeded 90% when cultured for 1 and 24 h. BKC: benzalkonium chloride, SDS: sodium dodecyl sulfate, TNBS: trinitrobenzene sulfonic acid, DNCB: dinitrochlorobenzene, DNFB: dinitrofluorobenzene.
Table 2
The change of mean fluorescence intensity of surface molecule expression on MoDC after incubation with contact allergen DNCB, irritant BKC, and cytokine cocktail as a positive control
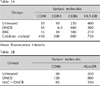
ACKNOWLEDGEMENTS
The authors thank Hyo Jin Roh and Jin Mo Park for giving experienced advice and kind support.
References
1. Rada B, Leto TL. Oxidative innate immune defenses by Nox/Duox family NADPH oxidases. Contrib Microbiol. 2008. 15:164–187.


2. Zabucchi G, Bellavite P, Berton G, Dri P. Free radicals generation by the inflammatory cells. Agents Actions Suppl. 1980. 7:159–166.


4. Finkel T, Holbrook NJ. Oxidants, oxidative stress and the biology of ageing. Nature. 2000. 408:239–247.


5. Waris G, Ahsan H. Reactive oxygen species: role in the development of cancer and various chronic conditions. J Carcinog. 2006. 5:14.


6. Valko M, Leibfritz D, Moncol J, Cronin MT, Mazur M, Telser J. Free radicals and antioxidants in normal physiological functions and human disease. Int J Biochem Cell Biol. 2007. 39:44–84.


7. Bickers DR, Athar M. Oxidative stress in the pathogenesis of skin disease. J Invest Dermatol. 2006. 126:2565–2575.


8. Bartosz G. Reactive oxygen species: destroyers or messengers? Biochem Pharmacol. 2009. 77:1303–1315.


9. Je JH, Lee TH, Kim DH, Cho YH, Lee JH, Kim SC, et al. Mitochondrial ATP synthase is a target for TNBS-induced protein carbonylation in XS-106 dendritic cells. Proteomics. 2008. 8:2384–2393.


10. Nordberg J, Arner ES. Reactive oxygen species, antioxidants, and the mammalian thioredoxin system. Free Radic Biol Med. 2001. 31:1287–1312.


12. Banchereau J, Steinmann RM. Dendritic cells and the control of immunity. Nature. 1998. 392:245–252.


13. Banchereau J, Briere F, Caux C, Davoust J, Lebecque S, Liu YJ, et al. Immunobiology of dendritic cells. Annu Rev Immunol. 2000. 18:767–811.


14. Fukunaga A, Khaskhely NM, Sreevidya CS, Byrne SN, Ullrich SE. Dermal dendritic cells, and not Langerhans cells, play an essential role in inducing an immune response. J Immunol. 2008. 180:3057–3064.


15. Grassi F, Dezutter-Dambuyant C, McIlroy D, Jacquet C, Yoneda K, Imamura S, et al. Monocyte-derived dendritic cells have a phenotype comparable to that of dermal dendritic cells and display ultrastructural granules distinct from Birbeck granules. J Leukoc Biol. 1998. 64:484–493.


16. Sallusto F, Lanzavecchia A. Efficient presentation of soluble antigen by cultured human dendritic cells is maintained by granulocyte/macrophage colony-stimulating factor plus interleukin 4 and downregulated by tumor necrosis factor alpha. J Exp Med. 1994. 179:1109–1118.


17. Choi GS, Kang JM, Lee MG. Analysis of methods for the generation of dendritic cells from human peripheral blood monocytes. Yonsei Med J. 2000. 41:642–650.


18. Kim DS, Kim DH, Byamba D, Lee TH, Cho YH, Lee MG. The production and functions of reactive oxygen species in mouse bone marrow-derived dendritic cells by various haptens and irritants. Korean J Dermatol. 2008. 46:1470–1477.


19. Mizuashi M, Ohtani T, Nakagawa S, Aiba S. Redox imbalance induced by contact sensitizers triggers the maturation of dendritic cells. J Invest Dermatol. 2005. 124:579–586.


20. Rutault K, Alderman C, Chain BM, Katz DR. Reactive oxygen species activate human peripheral blood dendritic cells. Free Radic Biol Med. 1999. 26:232–238.


21. Myhre O, Andersen JM, Aarnes H, Fonnum F. Evaluation of the probes 2',7'-dichlorofluorescin diacetate, luminol, and lucigenin as indicators of reactive species formation. Biochem Pharmacol. 2003. 65:1575–1582.


22. Wardman P. Fluorescent and luminescent probes for measurement of oxidative and nitrosative species in cells and tissues: progress, pitfalls, and prospects. Free Radic Biol Med. 2007. 43:995–1022.


23. Armstrong JS, Whiteman M. Measurement of reactive oxygen species in cells and mitochondria. Methods Cell Biol. 2007. 80:355–377.


24. Yoshida Y, Shimakawa S, Itoh N, Niki E. Action of DCFH and BODIPY as a probe for radical oxidation in hydrophilic and lipophilic domain. Free Radic Res. 2003. 37:861–872.


25. Covarrubias L, Hernández-García D, Schnabel D, Salas-Vidal E, Castro-Obregón S. Function of reactive oxygen species during animal development: passive or active? Dev Biol. 2008. 320:1–11.
26. Papa L, Gomes E, Rockwell P. Reactive oxygen species induced by proteasome inhibition in neuronal cells mediate mitochondrial dysfunction and a caspase-independent cell death. Apoptosis. 2007. 12:1389–1405.
27. Shin MH, Moon YJ, Seo JE, Lee Y, Kim KH, Chung JH. Reactive oxygen species produced by NADPH oxidase, xanthine oxidase, and mitochondrial electron transport system mediate heat shock-induced MMP-1 and MMP-9 expression. Free Radic Biol Med. 2008. 44:635–645.


28. Dalle-Donne I, Giustarini D, Colombo R, Rossi R, Milzani A. Protein carbonylation in human diseases. Trends Mol Med. 2003. 9:169–176.


29. Arouma OI, Halliwell B, Hoey BM, Butler J. The antioxidant action of N-acetylcysteine: its reaction with hydrogen peroxide, hydroxyl radical, superoxide, and hypochlorous acid. Free Radic Biol Med. 1989. 6:593–597.


30. Curtin JF, Donovan M, Cotter TG. Regulation and measurement of oxidative stress in apoptosis. J Immunol Methods. 2002. 265:49–72.


31. Stepnik M, Arkusz J. Molecular events associated with dendritic cells activation by contact sensitizers. Int J Occup Med Environ Health. 2003. 16:191–199.


32. Aiba S, Manome H, Nakagawa S, Mollah ZU, Mizuashi M, Ohtani T, et al. p38 Mitogen-activated protein kinase and extracellular signal-regulated kinases play distinct roles in the activation of dendritic cells by two representative haptens, NiCl2 and 2,4-dinitrochlorobenzene. J Invest Dermatol. 2003. 120:390–399.


33. Trompezinski S, Migdal C, Tailhardat M, Le Varlet B, Courtellemont P, Haftek M, et al. Characterization of early events involved in human dendritic cell maturation induced by sensitizers: cross talk between MAPK signalling pathways. Toxicol Appl Pharmacol. 2008. 230:397–406.


34. Watanabe H, Gaide O, Pétrilli V, Martinon F, Contassot E, Roques S, et al. Activation of the IL-1beta-processing inflammasome is involved in contact hypersensitivity. J Invest Dermatol. 2007. 127:1956–1963.

