Abstract
Background
Human skin is exposed to various environmental stresses, such as heat, cold, and ultraviolet (UV) radiation. Heat shock proteins (HSPs) induced by temperature elevations, as a physiologic response to mediate repair mechanisms and reduce cellular damage.
Objective
The purpose of this study was to investigate the induction of HSPs in human skin cells after UV exposure.
Methods
We performed immunoblotting using a specific monoclonal antibody to the HSP70 family, one of the best-conserved stress proteins in humans, with cultured normal human keratinocytes, A431 cells, human melanocytes, SK30 cells, and human dermal fibroblasts (HDF).
Results
Our results indicated that high expression of HSP70 in the unstressed state was noted in epidermal cells, including normal human keratinocytes, A431 cells, human melanocytes, and SK30 cells, but epidermal cells showed no additional up-regulation of HSP70 after UV irradiation. On the other hand, HDF expressed very small amounts of HSP70 at baseline, but significantly higher amounts of HSP70 after UV exposure.
In 1962, Ritossa incidentally made the seminalfinding of a 'puffing' pattern in the salivary glandpolytene chromosomes of Drosophila busckii afterexposure to heat12. This finding was the first step inthe study of a group of proteins, termed heat shock proteins (HSPs), which turned out to be expressed in all cells and organisms from prokaryotes to humans3.
Heat shock and other forms of pathophysiologic stressors induce the expression of HSPs in all types of cells and tissues. The heat shock response results in an increased expression of HSPs, enabling cells to resist damage from further stress exposure. These proteins are found intracellularly and exhibit high evolutionary conservation. This conservation implicates that HSP are necessary for successful survival under hostile environmental conditions4.
In addition to heat, a wide variety of other stress factors, including chemicals (heavy metals and amino acid analogs), glucose starvation, pathogens, and states of diseases, have been described as inducers of HSP expression567. These proteins are therefore referred to as stress proteins68. Ultraviolet (UV) radiation is one of the most abundant and potentially harmful environmental factors for human skin, so it is reasonable to inquire whether sun light exposure also induces HSP.
In order to understand the response of human epidermal cell lines (normal human keratinocytes, A431 cells, human melanocytes, and SK30 cells) we have examined the expression of HSP70 in those cells. In addition, the expression of HSP70 was determined in human dermal fibroblasts (HDF), which are the main cellular components of the dermis at baseline, and after heat treatment, UVA irradiation, and UVA+UVB irradiation. In this study, we used an immunoblotting method with monoclonal HSP70 antibody, which is a more sensitive method for detection of HSP than immunochemical techniques.
Normal human keratinocytes (NHKs): Neonatal keratinocytes were grown from human neonatal foreskin. The keratinocytes were cultured in keratinocyte growth media (Cascade Biologics, Portland, OR, USA).
A431 cells: The epidermoid carcinoma cell line, A431 cells, were cultured in RPMI 1640 (Gibco, Grand Island, NY, USA) supplemented with 10% fetal bovine serum.
Normal human melanocytes (NHMs): Human melanocytes were grown from human neonatal foreskin. The human melanocytes were cultured in MGM (Cascade Biologics).
SK30 cells: The malignant melanoma cell line, SK30 cells, was cultured in RPMI 1640 supplementedwith 10% fetal bovine serum.
Human dermal fibroblasts (HDFs): HDFs were grown from human neonatal foreskin. The cultured neonatal fibroblasts were grown in M199 (Gibco)supplemented with 10% fetal bovine serum.
The NHKs, A431 cells, NHMs, SK30 cells, and HDFs were incubated in a 43℃ dry heat chamberfor 90 min. After heat treatment, they were transferred to a CO2 incubator and incubated at 37℃ for 10 hours. The corresponding control cells (unstressed cells) were processed in the same manner.
A SOL 3 solar simulator (Dermalight Systems, Studio City, CA, USA) emitting 10% UVB+90% UVA with a H1 filter (295~400 nm), which issimilar to natural sunlight, and 100% UVA with a H2 filter (320~400 nm) were used. The intensity of 10% UVB+90% UVA was measured with a radiometer equipped with a UVB sensor. The NHKs, A431 cells, HDFs, NHMs, and SK30 cells were exposed to 10, 20, 30 and 50 mJ/cm2 amounts in the 10% UVB+90% UVA range. These cells were also irradiated at 4, 16, 32, and 48 J/cm2 amounts of 100% UVA. All the irradiated cells were incubated at 37℃ for 10 hours in a CO2 incubator.
We used acridine orange/ethidium bromide (AO/EB) staining in Lab-tek slides to determine cell viability. AO/EB solution 0.1 mg acridine orange (Sigma, St. Louis, MO, USA) and 0.1 mg ethidium bromide (Sigma), dissolved in 100 ml phosphate buffered saline (PBS) was added to each chamber (approximately 0.5 cc) and allowed to stand for 40~60 sec at room temperature. We then placed a cover slip on a slide and used a fluorescence microscope to examine it. Live cells had green nuclei, while non-viable cells had orange nuclei.
After incubation of the cell cultures at the conditions indicated above, the culture media were discarded, and the cells were lysed by repeated cycles of freeze-thawing in purified water. The lysates were centrifuged, and the supernatants were collected. The total protein content was measured by the method of Lowry et al9. Sodium dodecylsulfate-polyacrylamide gel electrophoresis (SDS-PAGE) was performed. Twenty micrograms of total protein were loaded onto each lane of a 12% gel. After electrophoresis, proteins were transferred to nitrocellulose membranes10. Slot blots were then performed for further quantification of HSP70 in whole cell extracts. Blots were incubated with primary antibody, the monoclonal mouse anti-human HSP70 antibody (Santa Cruz Biotechnology, Santa Cruz, CA, USA). This antibody reacts with HSP70 members, including the constitutive and inducible forms. Bound antibody was detected by peroxidaseconjugated goat anti-mouse immunoglobulins (Tagoimmunologicals, Camarillo, CA, USA). Enhanced chemiluminiscence (ECL; Amersham, Little Chalfont, Buckinghamshire, UK) was used as a detecting agent. We measured the density and the area of the expressed bands with the TINA program, version 2.0 (Raytest Isotopenmessgeraete, Straubenhardt, Germany), assigned a numerical value to them, converted them into percentages, and used them as the result from our study
Most cultured human epidermal cells viability did not change until 16 mJ/cm2 UVA irradiation. However, some floating cells with orange nuclei were observed in cultured A431 cells, NHMs, and SK30 cells after 16 mJ/cm2 UVA irradiation. Especially, in SK30 cells and NHMs, more nonviable cells were observed after UVA irradiation at doses of 16 and 32 J/cm2. In HDFs, some non-viable cells were observed after 32 mJ/cm2 UVA irradiation. In all cultured epidermal cells, after exposure to 10% UVB+90% UVA, non-viable cells were detected at 10 mJ/cm2 and the amount increased in a dose-dependent pattern.
Cultured NHKs: These cells constitutively expressed high amounts of HSP70 without stress. The expression of HSP70 was significantly increased after heat treatment. The expression of HSP70 was not greater after UVA exposure than at baseline. After exposure to 10% UVB+90% UVA, the expression of HSP70 did not change until 30 mJ/cm2 irradiation, but slight upregulation was noted at 50 mJ/cm2 (Fig. 1).
Cultured A431 cells: A431 cells constitutively expressed high amounts of HSP70 under unstressed conditions. The increased expression of HSP70 was induced after heat treatment. The expression of HSP70 was the same after UV exposure and at baseline. A431 cells showed an inverse proportion of HSP70 expression to the range of UVA doses. After exposure to 10% UVB+90% UVA, the expression of HSP70 did not change (Fig. 2).
Cultured NHMs: HSP70 was constitutively expressed under unstressed conditions in NHMs. The expression of HSP70 was highly increased after heat treatment. After 10 hours of incubation, irradiation with UVA at doses of 32 and 48 J/cm2 resulted in decreased expression of HSP70 compared to irradiation with UVA at 4 and 16 J/cm2. When subjected to 10% UVB+90% UVA, there was a dosedependent increase in the expression of the HSP70 family, but the expression was decreased at 50mJ/cm2(Fig. 3).
Cultured SK30 cells: At baseline (unstressed condition), HSP70 was constitutively expressed. The increased expression of HSP70 was noted after heat treatment. HSP70 was not up-regulated by UVA or by 10% UVB+90% UVA exposure after 10 hours of incubation (Fig. 4).
Cultured HDFs: The expression of HSP70 was markedly increased after heat treatment. Unlike epidermal cells, HDFs expressed very high amounts of HSP70 compared to baseline after UVA and 10% UVB+90% UVA exposures. After exposure to 10% UVB+90% UVA, HSP70 expression increased in a dose-dependent pattern (Fig. 5).
The HSP transiently bind to most cellular proteins and assist in both stabilization and conformational changes from synthesis to degradation.Therefore, HSP carry out molecular chaperone activity111213. Molecular chaperones are able to inhibit the aggregation of partially denatured proteins and to refold them using ATP14. Molecular chaperones prevent inappropriate associations of proteins with each other, and are involved in intracellular transport and the maintenance of proteins in an inactive form until required
These HSP are classified into the following five major family groups in mammalian cells14 based on their molecular size: HSP27, HSP60, HSP70, HSP90, and HSP110 proteins in mammalian cells14,1516. HSP70 is a set of the most conserved and the best characterized proteins in the mammalian system171819. HSP70 is composed of two major forms in mammalian cells. One is a constitutive form, Hsp73 (Hsc70), which is expressed at high levels at normal temperature and after heat shock. The other is the stress-inducible form Hsp72 (Hsp70), which is expressed at low levels at normal temperatures and at high levels after heat shock. HSP70 plays a major role in keeping the properly folded conformation of important structural proteins below the detection of HSP601620212223. Hsp70 is constitutively expressed in specific skin cells, such as epidermal keratinocytes, that provides a natural barrier against potential environmental stressful attacks242526. The expression of Hsp70 may be further induced in epidermal keratinocytes by heat treatment, providing a state of resistance against the deleterious effects of heat16
UV-induced HSP70 expression was first reported by Muramatsu et al7,27 in normal human skin system by an immunofluorescence method; Hsp70 expression was not detected at baseline7,27. However, Trautinger et al24 indicated that human keratinocytes constitutively express significant amounts of Hsp70. They24 explained this discrepancy may be due to different techniques, in particular to a higher sensitivity of their three-step immunochemical techniques, as compared to the indirect immunofluorescence of Muramatsu et al7,27. These different results raise the question as to whether UV exposure induces expression of HSP70 in normal human keratinocytes.
Our results showed that in cultured NHKs, down-regulation of HSP70 was noted after UVA exposure. Yet, after 10% UVB+90% UVA exposure, HSP70 expression did not change until 30 mJ/cm2, and at 50 mJ/cm2 slight up-regulation of HSP70 expression was noted. NHMs showed a high expression of HSP70 at baseline, and a slightly decreased HSP70 expression after 32 and 48 mJ/cm2 of UVA exposure, although not a significant difference. A431 and SK30 cells constitutively expressed high amounts of HSP70. No additional up-regulated expression of HSP70 was found after UV exposures than baseline. These data indicate that UV exposure may not have much of an effect on HSP70 expression in epidermal cells. Indeed, our results are supported by two other studies. Maytin5 found 19 stress proteins in mouse keratinocytesusing two-dimensional gel electrophoresis and classified them into 3 groups. According to this classification, the HSP70 and HSP90 families are belong to Group 1, which contains the stress proteins induced only by heat shock (not by UV exposure). Brunet and Giacomoni28 also indicated that Hsp70 mRNA up-regulation could not be induced by UV exposure, at least within 24 hours.
Other data indicated that HDFs show very high up-regulation of HSP70 after UVB and UVA exposure. This is supported by the fact that HSP70 overexpression in transfected fibroblasts was shown to improve cell survival after UV exposure29. Keyse and Tyrrell30 found 32 kD HSP after UVA exposure in EK4 fibroblasts and this HSP may be involved in a photoprotective function. Muramatsu et al7,27 suggested that a possible mechanism of inducing HSP expression in UV irradiated fibroblasts was due to UV-labile proteins or UV-induced DNA damage. Trautinger et al31 reported the expression of HSP72 after UVA radiation in a human fibrosarcoma cell line and suggested that induction of HSP72 is part of an adaptive response mechanism in human cells to UV-related stress.
In summary, epidermal cells (NHK, A431 cells, NHMs, and SK30 cells) showed high baseline HSP70 expression and no more up-regulation after UV irradiation, whereas HDFs showed very low baseline HSP70 expression and high up-regulation of expression after UV irradiation. Thus, the epidermal cells, which are directly exposed to the environment at the outer layer of the skin, possess the molecular chaperone activity of HSP70, not only in the physiologic state, but also in the stressed state. On the other hand, HDFs acquire molecular chaperone function after UV exposure, not in the physiologic state, but in the stress state.
Figures and Tables
Fig. 1
Western blot analysis of HSP70 expression from keratinocytes. UV exposure did not up-regulate HSP70 expression. Slight up-regulation was noted at 50 J/cm2 after 10% UVB+90% UVA exposure.
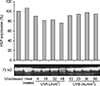
Fig. 2
Western blot analysis of HSP70 expression from A431 cells. No additional expression of HSP70 occurred after UV radiation compared to baseline. They showed a reverse dose-dependent pattern of HSP70 expression in the UVA range after 10 hours of incubation.
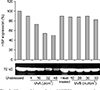
Fig. 3
Western blot analysis of HSP70 expression from melanocytes. The irradiation of UVA at doses of 32 and 48 J/cm2 resulted in a decreased expression of HSP70 compared to 4 and 16 J/cm2.
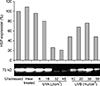
References
1. Ritossa F. A new puffing pattern induced by temperature shock and DNP in drosophila. Experientia. 1962; 18:571–573.


4. Jonak C, Klosner G, Trautinger F. Heat shock proteins in the skin. Int J Cosmet Sci. 2006; 28:233–241.


5. Maytin EV. Differential effects of heat shock and UVB light upon stress protein expression in epidermal keratinocytes. J Biol Chem. 1992; 267:23189–23196.


6. Holland DB, Roberts SG, Wood EJ, Cunliffe WJ. Cold shock induces the synthesis of stress proteins in human keratinocytes. J Invest Dermatol. 1993; 101:196–199.


7. Muramatsu T, Yamashina Y, Tada H, Kobayashi N, Yamaji M, Ohno H, et al. 8-methoxypsoralen plus UVA induces the 72 kDa heat shock protein in organ-cultured normal human skin. Photochem Photobiol. 1993; 58:809–812.


8. Welch WJ. Mammalian stress response: cell physiology, structure/function of stress proteins, and implications for medicine and disease. Physiol Rev. 1992; 72:1063–1081.


9. Lowry OH, Rosebrough NJ, Farr AL, Randall RJ. Protein measurement with the Folin phenol reagent. J Biol Chem. 1951; 193:265–275.


10. Laemmli UK. Cleavage of structural proteins during the assembly of the head of bacteriophage T4. Nature. 1970; 227:680–685.


11. Hendrick JP, Hartl FU. Molecular chaperone functions of heat-shock proteins. Annu Rev Biochem. 1993; 62:349–384.


12. Craig EA, Weissman JS, Horwich AL. Heat shock proteins and molecular chaperones: mediators of protein conformation and turnover in the cell. Cell. 1994; 78:365–372.


13. Wynn RM, Davie JR, Cox RP, Chuang DT. Molecular chaperones: heat-shock proteins, foldases, and matchmakers. J Lab Clin Med. 1994; 124:31–36.
14. Minowada G, Welch WJ. Clinical implications of the stress response. J Clin Invest. 1995; 95:3–12.


15. Welch WJ, Suhan JP. Cellular and biochemical events in mammalian cells during and after recovery from physiological stress. J Cell Biol. 1986; 103:2035–2052.


16. Maytin EV. Heat shock proteins and molecular chaperones: implications for adaptive responses in the skin. J Invest Dermatol. 1995; 104:448–455.


17. Pelham HR. Hsp70 accelerates the recovery of nucleolar morphology after heat shock. EMBO J. 1984; 3:3095–3100..


18. Ungewickell E. The 70-kd mammalian heat shock proteins are structurally and functionally related to the uncoating protein that releases clathrin triskelia from coated vesicles. EMBO J. 1985; 4:3385–3391.


19. Beckmann RP, Mizzen LE, Welch WJ. Interaction of Hsp 70 with newly synthesized proteins: implications for protein folding and assembly. Science. 1990; 248:850–854.


21. Trent JD, Nimmesgern E, Wall JS, Hartl FU, Horwich AL. A molecular chaperone from a thermophilic archaebacterium is related to the eukaryotic protein t-complex polypeptide-1. Nature. 1991; 354:490–493.


22. Horwich AL, Willison KR. Protein folding in the cell: functions of two families of molecular chaperone, hsp 60 and TF55-TCP1. Philos Trans R Soc Lond B Biol Sci. 1993; 339:313–325.


23. Frydman J, Nimmesgern E, Ohtsuka K, Hartl FU. Folding of nascent polypeptide chains in a high molecular mass assembly with molecular chaperones.
. Nature. 1994; 370:111–117.


24. Trautinger F, Trautinger I, Kindas-Mugge I, Metze D, Luger TA. Human keratinocytes in vivo and in vitro constitutively express the 72-kD heat shock protein. J Invest Dermatol. 1993; 101:334–338.


25. Charveron M, Calvo M, Gall Y. Cell stress and implications of the heat-shock response in skin. Cell Biol Toxicol. 1995; 11:161–165.


26. Laplante AF, Moulin V, Auger FA, Landry J, Li H, Morrow G, et al. Expression of heat shock proteins in mouse skin during wound healing. J Histochem Cytochem. 1998; 46:1291–1301.


27. Muramatsu T, Tada H, Kobayashi N, Yamji M, Shirai T, Ohnishi T. Induction of the 72-kD heat shock protein in organ-cultured normal human skin. J Invest Dermatol. 1992; 98:786–790.


28. Brunet S, Giacomoni PU. Heat shock mRNA in mouse epidermis after UV irradiation. Mutat Res. 1989; 219:217–224.


29. Simon MM, Reikerstorfer A, Schwarz A, Krone C, Luger TA, Jaattela M, et al. Heat shock protein 70 overexpression affects the response to ultraviolet light in murine fibroblasts. Evidence for increased cell viability and suppression of cytokine release. J Clin Invest. 1995; 95:926–933.

