Abstract
Central serous chorioretinopathy (CSC) is an eye disease that causes serous retinal detachment in the posterior pole of the retina. The pathogenesis of CSC is not fully understood and various systemic factors have been reported to be associated with CSC. Recently, with the advent of advanced imaging techniques, novel imaging findings for CSC have been reported and the understanding of CSC has increased further. Moreover, in addition to conventional treatment for CSC, new treatment modalities such as photodynamic therapy, anti-vascular endothelial growth factor or subthreshold laser therapy, have emerged. In this article, an overall review and update of CSC, particularly focusing on new imaging findings and treatments, will be discussed.
Central serous chorioretinopathy (CSC) is characterized by the accumulation of subretinal fluid (SRF) at the posterior pole of the retina, which leads to mild to moderate visual disturbance [1]. It was first described as central recurrent retinitis by Albrecht von Graefe in 1866 [2]. In 1965, Maumenee found that macular serous detachment was due to an abnormal leak from retinal pigment epithelium (RPE) through fluorescein angiography (FA) [3] and thereafter, Gass used the term “central serous chorioretinopathy” for the first time [4]. CSC is the fourth most common macular disease following age-related macular degeneration, diabetic retinopathy and retinal vein occlusion, but it is an important clinical issue due to its common incidence seen in the productive age group (young and middle-aged adults) [5].
CSC is an old and well-known disease, however, the exact pathogenesis is still unknown. With the recent introduction of advanced imaging techniques, more improved understanding of CSC is possible and subsequently, better treatment modalities have also been suggested. This article describes a general overview and recent clinical issues of CSC.
Historically, CSC has been known to occur usually in young and middle aged men. A most recent epidemiologic study reported that the average age of CSC has ranged from 39 and 51 years old [5]. A population-based study showed that the incidence of CSC was 9.9 / 100,000 individuals and men are approximately six times more likely to develop CSC compared to women. However, this gender difference was meaningful only in young age groups, indicating women present CSC at more elderly ages [56]. The ethnic differences regarding the incidence of CSC were not confirmed. However, several studies reported that severe and atypical forms of CSC were more frequently observed in African- American or Asian subjects compared to the Western population [789].
CSC can occur without definite known causes but, numerous risk factors have been investigated. Among various risk factors, corticosteroid usage is a most potent factor. Although systemic administration (oral or intravenous) of corticosteroid has been consistently reported as an independent risk factor of CSC, CSC can occur via local administrations such as intranasal, epidural, intra-articular, topical dermal, and periocular [61011]. In addition to the development of CSC, corticosteroid use is associated with prolongation, aggravation and recurrences [12].
Steroid-induced CSC tends to present more severe, bilateral and atypical patterns and has more female dominance than idiopathic CSC [12]. CSC after organ transplantation is associated with the usage of high-dose corticosteroid for immunosuppression [13]. As well as exogenous administration of corticosteroid, elevated level of endogenous corticosteroids such as Cushing's syndrome is also associated with CSC [11].
During pregnancy, risk of CSC is increased, particularly in the third trimester due to elevated level of serum cortisol levels. Pregnancy-induced CSC usually alleviates spontaneously after delivery [14].
Psychopathology such as type-A personality and emotional stress was suggested as a risk factor of CSC by Yannuzzi in 1986 at first [15]. Subsequently, other studies have shown that anti-psychotropic medication use and psychiatric stress were associated with CSC [16].
Some gastrointestinal diseases such as gastroesophageal reflux and peptic ulcer have been reported to be a risk factor of CSC [17]. In addition, several investigators reported that the prevalence of Helicobacter pylori is high in patients with CSC [18].
Various factors other than mentioned above have been reported, but recent meta-analysis study suggested hypertension (odds ratio (OR) = 1.7; 95% confidence interval (CI): 1.28–2.25), Helicobacter pylori infection (OR = 3.12; 95% CI: 1.81–5.40), steroid usage (OR = 4.29; 95% CI: 2.01–9.15), sleeping disturbance (OR = 1.90; 95% CI: 1.28–1.83), autoimmune disease (OR=3.44; 95% CI: 1.90–6.26), psychopharmacologic medication use (OR = 2.69; 95% CI: 1.63–4.45), and Type-A personality (OR = 2.53; 95% CI: 1.08–5.96) as potential risk factors for CSC [10].
According to the duration of symptoms and the recurrence, CSC can be classified into acute and chronic and recurrent form. However, there has been no definite objective system for assessing the chronicity of CSC to date. In most published articles, the duration threshold is set between three and six months arbitrarily [1920].
There has still been controversy over whether the primary lesion of CSC is choroid or abnormal RPE and whether CSC is a focal or widespread disease. However, recent imaging studies have shown that CSC may be developed by abnormal choroidal vascular flow. Various risk factors mentioned above may induce choroidal vascular dysfunction by ischemia, stasis and inflammation, which lead to abnormal hyperpermeability of the inner choroid and retinal pigment epithelial detachment (RPED). Subsequently, serous fluid accumulates in subretinal space through RPED [212223].
Adrenergic stimulation and corticosteroids have been reported to be associated with the pathogenesis of CSC. Yoshioka et al. induced experimental CSC in monkey eyes by injecting epinephrine, which indicated that adrenergic stimulus may lead to choroidal vascular constriction and abnormal choroidal flow resulting in CSC [24]. Particularly, corticosteroids are also considered as a major cause of CSC. The exact role of corticosteroids is unknown but, it can influence the expression of adrenergic receptor genes [25] and hinder the healing process of damaged RPE or weaken the choriocapillaris, thereby leading to choroidal hyperpermeability [26]. In addition, corticosteroids can alter the polarity of RPE by direct influence on ion transport, which facilitate fluid shift to subretinal space from the choroid [27].
Acute CSC is usually considered a benign, self-limiting disease with favorable visual prognosis. Serous retinal detachment usually can resolve spontaneously within 12 weeks without treatment, but visual recovery can be delayed up to 12 months [28]. In contrast, chronic or recurrent CSC with persistent sensory retinal detachment may damage the foveal photoreceptor layer, cause foveal attenuation and chronic macular edema, leading to permanent visual disturbances such as central visual loss, color vision deficiency, relative scotoma, micropsia, metamorhopsia, decreased contrast sensitivity and night blindness [293031]. One-third to one-half of patients with CSC experience recurrence and 10% of them have recurrences of more than three times. In recurrent cases, 50% of them occur within the first 1 year [1920].
A discrete, clear and serous elevated lesion is observed at the posterior pole (Fig 1). In chronic form, multiple small deposits are observed between the RPE and sensory retina. RPEDs can be observed within or near the area of sensory retinal detachment. The characteristics of subretinal fluid is mostly clear but can be turbid due to fibrous deposits in some chronic, recurrent or atypical cases induced by pregnancy, steroid usage or organ-transplant. Persistent subretinal fibrin can resolve spontaneously or remain as sequalae such as macular atrophy, secondary choroidal neovascularization (CNV) or fibrous scar, resulting in permanent visual loss. Subretinal fluid moves downward under the influence of gravity, resulting in various shapes of sensory detachment such as tear, dumbbell, or flask [3151920]. Atrophic tract connecting posterior pole and inferior sensory detachment can be observed in some chronic cases, which can be more pronounced in FA and fundus autofluorescence (FAF) [213233].
FA has been the most widely used imaging modality for the diagnosis of retinal diseases. FA shows one or more characteristic leaking points in the RPE which is observed in approximately 95% of all CSC patients. The leaking points are mainly located within 500-1500 µm from the center of the fovea and 10% of the cases are located in the extrafoveal area. The most common leaking pattern is an ‘ink blot’ pattern described by an early hyperfluorescent spot that gradually enlarges. The second most common leaking pattern (7-25%) is a ‘smoke stack appearance’ which is formed when dye passes into the subretinal space and ascends vertically to highest points and spreads laterally like umbrella or mushroom configuration until the entire area of serous detachment is filled (Fig 1) [3233]. In acute forms, most cases had a single leakage site, rarely two or more [34]. In contrast, chronic CSC cases have diffuse RPE decompensation secondary to choroidal hyperpermeability, which differs from the focal RPE change of acute CSC and show multifocal leakages in mid- and late phases as patches of granular hyperfluorescence (Fig 2) [293031]. Finally, old and inactive CSC cases with sequelae appear as hyperfluorescent areas (window defect) due to increased transmission by RPE atrophy [3233]. Focal laser photocoagulation can be performed for treatment of CSC using FA findings.
Indocyanine green angiography (ICGA) has been used as a gold standard for the detection of choroidal vascular abnormalities. In early phase, filling delays and hypofluorescent areas are observed resulting from decreased choriocapillaris filling [35]. In mid-phase, multiple geographical hyperfluorescent areas with dilating choroidal vessels appear as ‘choroidal vascular hyperpermeability’ which is one of the hallmark findings of CSC imaging. In late-phase, hyperfluorescent areas may either persist, wash-out or form hyperfluorescent rings. The mid-phase ICGA findings (hyperfluorescent areas) account for a key role of choroidal hyperpermeability in CSC pathogenesis (Fig 2). These ICGA findings shown in affected eyes are also observed in more than half of asymptomatic fellow eyes [36]. The hyperfluorescent areas are significantly associated with increased choroidal thickness on optical coherence tomography (OCT) [22].
OCT is a noninvasive modality evaluating microstructural abnormalities of the retina in detail. Since time-domain OCT was first commercially available, OCT technology has evolved remarkably and spectral-domain OCT (SD-OCT), which provides high-definition images, is now widely used in current clinical practice. In addition, recently, the advents of enhanced-depth imaging OCT (EDI-OCT) [37] and swept-source OCT have allowed the visualization of the choroid, evaluating the morphologic changes of choroidal vessels and choroidal thickness [38].
Many studies have reported the detailed morphologic alterations occurring in eyes with CSC, particularly the changes of the RPE and the outer retina [20233940]. In the RPE layer, PED presents in most cases of CSC and is associated with leaking site. Other abnormal RPE findings such as microrip of the RPE, RPE hypertrophy and RPE atrophy have been reported. In the site of serous retinal detachment, elongated photoreceptor outer segments or thickened posterior surface of detached retina is observed, particularly in acute CSC. Subretinal hyper-reflective multiple deposits corresponding to fundus yellow-white precipitates and fibrinous exudates at the leaking sites can be observed. In the retinal layer, intraretinal hyper-reflective deposits, disruptions of photoreceptor layer, foveal atrophy and/or cystoid macular degeneration appear according to the chronicity of CSC. In the choroidal layer, the most distinct feature is thickened subfoveal choroid and dilated choroidal vessels which are comparable to hyperfluorescent areas in mid-phase of ICGA [22]. Theses RPE abnormalities and thickened choroidal layer shown in affected eyes are also observed in asymptomatic fellow eyes [41].
Abnormal SD-OCT findings of CSC do not appear at the same time. Recent SD-OCT studies suggest that the chronicity of CSC can be identified through detailed OCT interpretation [40]. In acute CSC, bullous serous retinal detachment, thickened posterior surface of the detached retina and retinal dragging are usually observed. As CSC becomes chronic, shallow serous retinal detachment and thinned posterior surface of the detached retina are more observed on SD-OCT.
Fundus autofluorescence (FAF) is non-invasive method to evaluate metabolic activity of RPE cells. FAF signal mostly originates from lipofuscin in the RPE. Various FAF signals are observed according to the chronicity of CSC [42]. In eyes with acute CSC, hypo-FAF is initially seen due to masking effect of serous retinal detachment and elongated photoreceptor outer segments [42]. Another retrospective study [43] suggests that most cases with acute CSC showed relatively homogenous hyper-FAF or minimal changes in the area of serous retinal detachment, while more heterogeneous patterns of hyper-FAF are more frequently observed in cases with chronic CSC than in those with acute CSC (Fig 2). Especially, characteristic descending tracts with decreased FAF, often originating from the macular and the optic disc, present in cases with chronic CSC. In fellow eyes of unilateral cases, various FAF changes can be also seen, which may reflect previous unnoticed CSC episodes or focal RPE changes.
Acute CSC is a self-limited disease and spontaneous resolves within 3-4 months in the majority of cases. Therefore, observation is the first-line treatment of acute CSC [44]. The modification of risk factors is also important such as discontinuation of steroid usage, reduction of stress level or psychological therapies [45]. However, in cases with long-standing CSC, other treatment should be considered because long-standing serous retinal detachment can lead to permanent visual disturbance [39].
Since the 1970s, focal photocoagulation has been used for CSC if the leaking sites are located outside the fovea [46]. The mechanism has been thought that focal photocoagulation destroys abnormal RPEs and allows the adjacent RPE to take over their function, thereby activating RPE pump function to move fluid and proteins from the subretinal area to choroidal space. The final visual outcome and recurrence after focal photocoagulation for CSC has still been a subject of controversy. Robertson and Listrup [47] reported that focal photocoagulation showed quicker resolution of subretinal fluid and prevention of recurrence of CSC compared to untreated group. However, it did not show the effect of reducing recurrence of CSC by the longest available follow-up studies (6-12 years). Other studies have shown consistent results in hastening the resolution of subretinal fluid, but no statistical difference in final visual outcome was reported between untreated and laser treated groups [4648]. Focal photocoagulation is generally known to be safe but can induce adverse effects such as paracentral or central scotoma and secondary CNV, particularly if performed near the foveal area [2748].
Focal photocoagulation cannot be sufficient treatment for CSC because it does not solve the choroidal pathology. Photodynamic therapy (PDT) is a treatment approach directed toward causative pathology such as choroidal hyperpermeability. The mechanism of PDT is that verteporfin (Visudyne®; Novartis, Switzerland) after intravenous administration releases free radicals under laser stimulation at 693 nm in the choroidal circulation, leading to the endothelial vascular damage. The therapeutic effect of PDT in chronic CSC is attributed to the occlusion of choriocapillaris, subsequently leading to a decrease in choroidal hyperpermeability and resorption of SRF [50].
Because ICGA shows the area of choroidal hyperpermeabitliy, Yannuzzi and other investigators have performed ICGA-guided PDT for chronic CSC [750]. The research used standard PDT protocol for chronic CSC suggested by the treatment of age-related macular degeneration with photodynamic therapy (TAP) study. Studies have found that choroidal thickness and choroidal hyperfluorecence were reduced on EDI-OCT and ICGA respectively, while no choroidal changes were observed after focal photocoagulation [51]. However, approved PDT protocol (a dose of 6 mg/m2 with a laser fluence of 50 J/cm2) for CNV can cause some complications such as RPE atrophy, choriocapillary hypoperfusion and secondary CNV, subsequently leading to poor visual outcome [52]. Therefore, standard PDT protocol has been modified for CSC to minimize side effects of PDT. Two modified protocols (half-dose PDT and half-fluence PDT) have been investigated comparing them to standard PDT. According to recent studies, both half-dose PDT and half-fluence PDT were as effective as standard PDT and reduced PDT-related complications [5354]. However, whether protocol (half-dose vs. half-fluence) is superior for CSC is still controversial [5556].
The important issue to recognize is that there are no long-term follow-up studies regarding visual outcome, the rates of recurrence and complications after single or multiple PDT. Further research is needed to confirm the effect and safety of PDT.
More recently, the subthreshold diode micropulse laser (810 nm) was proposed for CSC [57]. This laser can penetrate deeper tissue and approach within the foveal area. Studies have found the subthreshold diode micropulse laser showed similar therapeutic effect without complications compared to PDT [4957]. But the set of clinical end points and dose titration are still problems to be solved.
Intravitreal anti-vascular endothelial growth factor (anti-VEGF) has been widely used for treatment of various retinal diseases such as age-related macular degeneration, diabetic macular edema and macular edema secondary to retinal vein occlusion. The rationale of anti-VEGF for CSC is that the reduction of subretinal fluid is expected by decreasing intravitreal VEGF concentration, which has a role in vascular hyerpermeability.
The effect of anti-VEGF treatment (bevacizumab and ranibizumab) for chronic CSC has been controversial. Several studies have reported hopeful results, but they have limitations in that most are short-term studies [58596061]. Recent randomized clinical trials compared the therapeutic effects of low-fluence PDT versus intravitreal ranibizumab for chronic CSC [62]. This study reported that half-fluence PDT produced better anatomical results in completely resolving subretinal fluid than did intravitreal ranibizumab for chronic CSC. Lim et al. reported that the level of VEFG in both the plasma and aqueous humor of patients with CSC was not increased compared with healthy subjects [51]. Recently, intravitreal aflibercept has been used to manage neovascular age-related macular degeneration, macular edema secondary to diabetic retinopathy and retinal vein occlusion. Potent and longer pharmacologic effects have been expected because aflibercept has some characteristics compared to previous anti-VEGF drugs as followings : more potent VEGF receptor binding effect, the inhibition of additional VEGF subtype (VEGF-A) and placental growth factor (PIGF) as well as VEGF-A. A prospective pilot study suggested that intravitreal aflibercept was tolerated and safe for short-term period (6-month), but large controlled studies are necessary [49].
Corticosteroid antagonist [63] and adrenergic receptor inhibitors [64] have been proposed for treatment of CSC because of their role in pathogenesis of CSC. Acetazolamide (carbonic anhydrase inhibitor) has also been suggested as a means of rapid resorption of subretinal fluid [65]. However, no sufficient evidence has been reported to confirm the effectiveness of these drugs.
With the evolution of imaging technique, understanding of CSC has become deeper than in the past and new treatment has emerged, making it possible to treat more actively than before. Cochrane and authors who performed a systematic review commented that the overall level of evidence of previous published literatures regarding interventions for CSC was low or very low, and there was not enough evidence of which treatments are better [49]. Future research is still warranted to acquire high level of evidence.
Figures and Tables
Fig. 1
Representative case of acute central serous chorioretinopathy. Top left, fundus photography shows discrete, clear and serous elevation in central area. Green arrow indicates the area of section on optical coherence tomography. Bottom left, optical coherence tomography shows subretinal fluid, thickened posterior surface of detached retina and semicircular pigment epithelial detachment. Right, fluorescein angiography shows smoke-stack like dye leakage (Top; early phase, bottom; late phase).
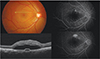
Fig. 2
Representative case of chronic central serous chorioretinopathy. Upper left, fundus photography shows subtle serous elevation in central area. Green arrow indicates the area of section on optical coherence tomography. Middle left, optical coherence shows shallow subretinal fluid, thinned posterior surface of detached retina, subretinal hype-reflective foci. Top right, fundus autofluorscence shows heterogeneous patterns of hyper-fluorescence. Bottom left, fluorescein angiography (mid-phase) shows multifocal leakages as patches of granular hyperfluorescence. Bottom right, indocyanine green angiography (late phase) shows hyperfluorescent areas with dilating choroidal vessels (choroidal vascular hyperpermeability).
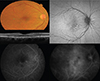
References
1. Spaide RF, Campeas L, Haas A, Yannuzzi LA, Fisher YL, Guyer DR, et al. Central serous chorioretinopathy in younger and older adults. Ophthalmology. 1996; 103:2070–2079.


2. Von Graefe A. Ueber centrale recidivierende retinitis. Graefes Arch Clin Exp Ophthalmol. 1866; 12:211–215.
3. Maumenee A. Clinical manifestations (Symposium: macular diseases). Trans Am Acad Ophthalmol Otolaryngol. 1965; 69:605–613.
4. Donald J, Gass M. Pathogenesis of disciform detachment of the neuroepithelium: II. Idiopathic central serous choroidopathy. Am J Ophthalmol. 1967; 63:587/15–615/43.
5. Kitzmann AS, Pulido JS, Diehl NN, Hodge DO, Burke JP. The incidence of central serous chorioretinopathy in Olmsted County, Minnesota, 1980–2002. Ophthalmology. 2008; 115:169–173.


6. Haimovici R, Koh S, Gagnon DR, Lehrfeld T, Wellik S. Group CSCCCS. Risk factors for central serous chorioretinopathy: a case–control study. Ophthalmology. 2004; 111:244–249.
7. Chan WM, Lai TY, Tano Y, Liu DT, Li KK, Lam DS. Photodynamic therapy in macular diseases of Asian populations: when East meets West. Jpn J Ophthalmol. 2006; 50:161–169.


8. Balo K, Mihluedo H. [Idiopathic central serous chorioretinopathy: two case reports observed in Togo]. Med Trop (Mars). 1996; 56:381–383.
9. Desai UR, Alhalel AA, Campen TJ, Schiffman RM, Edwards PA, Jacobsen GR. Central serous chorioretinopathy in African Americans. J Natl Med Assoc. 2003; 95:553–559.
10. Liu B, Deng T, Zhang J. RISK FACTORS FOR CENTRAL SEROUS CHORIORETINOPATHY: A Systematic Review and Meta-Analysis. Retina. 2016; 36:9–19.
11. Carvalho-Recchia CA, Yannuzzi LA, Negrao S, Spaide RF, Freund KB, Rodriguez-Coleman H, et al. Corticosteroids and central serous chorioretinopathy. Ophthalmology. 2002; 109:1834–1837.


12. Khairallah M, Kahloun R, Tugal-Tutkun I. Central serous chorioretinopathy, corticosteroids, and uveitis. Ocul Immunol Inflamm. 2012; 20:76–85.


13. Chung H, Kim KH, Kim JG, Lee SY, Yoon YH. Retinal complications in patients with solid organ or bone marrow transplantations. Transplantation. 2007; 83:694–699.


14. Mayo GL, Tolentino MJ. Images in clinical medicine. Central serous chorioretinopathy in pregnancy. N Engl J Med. 2005; 353:e6.
15. Yannuzzi LA. Type A behavior and central serous chorioretinopathy. Trans Am Ophthalmol Soc. 1986; 84:799.


16. Tittl MK, Spaide RF, Wong D, Pilotto E, Yannuzzi LA, Fisher YL, et al. Systemic findings associated with central serous chorioretinopathy. Am J Ophthalmol. 1999; 128:63–68.


17. Mansuetta CC, Mason JO, Swanner J, Feist RM, White MF, Thomley ML, et al. An association between central serous chorioretinopathy and gastroesophageal reflux disease. Am J Ophthalmol. 2004; 137:1096–1100.


18. Cotticelli L, Borrelli M, d'Alessio A, Menzione M, Villani A, Piccolo G, et al. Central serous chorioretinopathy and Helicobacter pylori. Eur J Ophthalmol. 2006; 16:274–278.


19. Daruich A, Matet A, Dirani A, Bousquet E, Zhao M, Farman N, et al. Central serous chorioretinopathy: Recent findings and new physiopathology hypothesis. Prog Retin Eye Res. 2015; 48:82–118.


20. Ross A, Ross AH, Mohamed Q. Review and update of central serous chorioretinopathy. Curr Opin Ophthalmol. 2011; 22:166–173.


21. Framme C, Walter A, Gabler B, Roider J, Sachs HG, Gabel VP. Fundus autofluorescence in acute and chronic-recurrent central serous chorioretinopathy. Acta Ophthalmol Scand. 2005; 83:161–167.


22. Imamura Y, Fujiwara T, Margolis R, Spaide RF. Enhanced depth imaging optical coherence tomography of the choroid in central serous chorioretinopathy. Retina. 2009; 29:1469–1473.


23. Montero J, Ruiz-Moreno J. Optical coherence tomography characterisation of idiopathic central serous chorioretinopathy. Br J Ophthalmol. 2005; 89:562–564.


24. Yoshioka H, Katsume Y, Akune H. Experimental Central Serous Chorioretinopathy in Monkey Eyes: Fluorescein Angiographic Findings. Ophthalmologica. 1982; 185:168–178.


25. Sakaue M, Hoffman BB. Glucocorticoids induce transcription and expression of the alpha 1B adrenergic receptor gene in DTT1 MF-2 smooth muscle cells. J Clin Invest. 1991; 88:385.


26. Caccavale A, Romanazzi F, Imparato M, Negri A, Morano A, Ferentini F. Central serous chorioretinopathy: a pathogenetic model. Clin Ophthalmol. 2011; 5:239.


27. Verma L, Purohit A, Tewari H, Biswas N, Talwar D, Jhingan S, et al. A study of endogenous cortisol profile in patients with central serous retinopathy with single and multiple leaks. Indian J Pharmacol. 2001; 33:96–99.
28. Gilbert CM, Owens SL, Smith PD, Fine SL. Long-term follow-up of central serous chorioretinopathy. Br J Ophthalmol. 1984; 68:815–820.


29. Yannuzzi LA, Shakin JL, Fisher YL, Altomonte MA. Peripheral retinal detachments and retinal pigment epithelial atrophic tracts secondary to central serous pigment epitheliopathy. Ophthalmology. 1984; 91:1554–1572.


30. Jalkh AE, Jabbour N, Avila MP, Trempe CL, Schepens CL. Retinal pigment epithelium decompensation. I. Clinical features and natural course. Ophthalmology. 1984; 91:1544–1548.
31. Iida T, Yannuzzi LA, Spaide RF, Borodoker N, Carvalho CA, Negrao S. Cystoid macular degeneration in chronic central serous chorioretinopathy. Retina. 2003; 23:1–7. quiz 137-8.


32. Gäckle HC, Lang GE, Freißler KA, Lang GK. Clinical, fluorescein angiographic and demographic aspects in central serous chorioretinopathy. Ophthalmologe. 1998; 95:529–533.


33. Yamada K, Hayasaka S, Setogawa T. Fluorescein-angiographic patterns in patients with central serous chorioretinopathy at the initial visit. Ophthalmologica. 1992; 205:69–76.


34. Burumcek E, Mudun A, Karacorlu S, Arslan MO. Laser photocoagulation for persistent central serous retinopathy: results of long-term follow-up. Ophthalmology. 1997; 104:616–622.


35. Prünte C. Indocyanine green angiographic findings in central serous chorioretinopathy. Int Ophthalmol. 1995; 19:77–82.


36. Iida T, Kishi S, Hagimura N, Shimizu K. Persistent and bilateral choroidal vascular abnormalities in central serous chorioretinopathy. Retina. 1999; 19:508–512.


37. Spaide RF. Enhanced depth imaging optical coherence tomography of retinal pigment epithelial detachment in age-related macular degeneration. Am J Ophthalmol. 2009; 147:644–652.


38. Hirata M, Tsujikawa A, Matsumoto A, Hangai M, Ooto S, Yamashiro K, et al. Macular choroidal thickness and volume in normal subjects measured by swept-source optical coherence tomography. Invest Ophthalmol Vis Sci. 2011; 52:4971–4978.


39. Nicholson B, Noble J, Forooghian F, Meyerle C. Central serous chorioretinopathy: update on pathophysiology and treatment. Surv Ophthalmol. 2013; 58:103–126.


40. Song IS, Shin YU, Lee BR. Time-periodic characteristics in the morphology of idiopathic central serous chorioretinopathy evaluated by volume scan using spectral-domain optical coherence tomography. Am J Ophthalmol. 2012; 154:366–375.


41. Maruko I, Iida T, Sugano Y, Ojima A, Sekiryu T. Subfoveal choroidal thickness in fellow eyes of patients with central serous chorioretinopathy. Retina. 2011; 31:1603–1608.


42. Eandi CM, Ober M, Iranmanesh R, Peiretti E, Yannuzzi LA. Acute central serous chorioretinopathy and fundus autofluorescence. Retina. 2005; 25:989–993.


43. Lee WJ, Lee JH, Lee BR. Fundus autofluorescence imaging patterns in central serous chorioretinopathy according to chronicity. Eye (Lond). 2016; 30:1336–1342.


44. Yannuzzi LA. Central serous chorioretinopathy: a personal perspective. Am J Ophthalmol. 2010; 149:361–363.e1.


45. Sharma T, Shah N, Rao M, Gopal L, Shanmugam MP, Gopalakrishnan M, et al. Visual outcome after discontinuation of corticosteroids in atypical severe central serous chorioretinopathy. Ophthalmology. 2004; 111:1708–1714.


46. Leaver P, Williams C. Argon laser photocoagulation in the treatment of central serous retinopathy. Br J Ophthalmol. 1979; 63:674–677.


47. Robertson DM. Argon laser photocoagulation treatment in central serous chorioretinopathy. Ophthalmology. 1986; 93:972–974.


48. Gilbert CM, Owens SL, Smith PD, Fine SL. Long-term follow-up of central serous chorioretinopathy. Br J Ophthalmol. 1984; 68:815–820.


49. Salehi M, Wenick AS, Law HA, Evans JR, Gehlbach P. Interventions for central serous chorioretinopathy: a network meta-analysis. Cochrane Database Syst Rev. 2015; CD011841.


50. Taban M, Boyer DS, Thomas EL, Taban M. Chronic central serous chorioretinopathy: photodynamic therapy. Am J Ophthalmol. 2004; 137:1073–1080.


51. Lim JW, Kang SW, Kim Y-T, Chung SE, Lee SW. Comparative study of patients with central serous chorioretinopathy undergoing focal laser photocoagulation or photodynamic therapy. Br J Ophthalmol. 2011; 95:514–517. DOI: bjo.2010.182121.


52. Inoue R, Sawa M, Tsujikawa M, Gomi F. Association between the efficacy of photodynamic therapy and indocyanine green angiography findings for central serous chorioretinopathy. Am J Ophthalmol. 2010; 149:441–446.e2.


53. Shin JY, Woo SJ, Yu HG, Park KH. Comparison of efficacy and safety between half-fluence and full-fluence photodynamic therapy for chronic central serous chorioretinopathy. Retina. 2011; 31:119–126.


54. Reibaldi M, Cardascia N, Longo A, Furino C, Avitabile T, Faro S, et al. Standard-fluence versus low-fluence photodynamic therapy in chronic central serous chorioretinopathy: a nonrandomized clinical trial. Am J Ophthalmol. 2010; 149:307–315.e2.


55. Nicoló M, Eandi CM, Alovisi C, Grignolo FM, Traverso CE, Musetti D, et al. Half-fluence versus half-dose photodynamic therapy in chronic central serous chorioretinopathy. Am J Ophthalmol. 2014; 157:1033–1037.e2.


56. Alkin Z, Ozkaya A, Agca A, Yazici AT, Demirok A. Early visual and morphologic changes after half-fluence photodynamic therapy in chronic central serous chorioretinopathy. J Ocul Pharmacol Ther. 2014; 30:359–365.


57. Roisman L, Magalhães FP, Lavinsky D, Moraes N, Hirai FE, Cardillo JA, et al. Micropulse diode laser treatment for chronic central serous chorioretinopathy: a randomized pilot trial. Ophthalmic Surg Lasers Imaging Retina. 2013; 44:465–470.


58. Lim SJ, Roh MI, Kwon OW. Intravitreal bevacizumab injection for central serous chorioretinopathy. Retina. 2010; 30:100–106.


59. Artunay O, Yuzbasioglu E, Rasier R, Sengul A, Bahcecioglu H. Intravitreal bevacizumab in treatment of idiopathic persistent central serous chorioretinopathy: a prospective, controlled clinical study. Curr Eye Res. 2010; 35:91–98.


60. Seong HK, Bae JH, Kim ES, Han JR, Nam WH, Kim HK. Intravitreal bevacizumab to treat acute central serous chorioretinopathy: short-term effect. Ophthalmologica. 2009; 223:343–347.


61. Lee ST, Adelman RA. The Treatment of Recurrent Central Serous Chorioretinopathy with Intravitreal Bevacizumab. J Ocul Pharmacol Ther. 2011; 27:611–614.


62. Bae SH, Heo JW, Kim C, Kim TW, Lee JY, Song SJ, et al. A randomized pilot study of low-fluence photodynamic therapy versus intravitreal ranibizumab for chronic central serous chorioretinopathy. Am J Ophthalmol. 2011; 152:784–792.e2.


63. Meyerle CB, Freund KB, Bhatnagar P, Shah V, Yannuzzi LA. Ketoconazole in the treatment of chronic idiopathic central serous chorioretinopathy. Retina. 2007; 27:943–946.

