Abstract
Medical therapies and mechanical interventions for the treatment of myocardial infarction (MI) and ischemic heart failure have seen great progress. However, current therapies only slow the progression to heart failure, but do not stimulate regeneration to recover the loss of functional myocytes. Stem cell-based therapy is a novel modality that can potentially be used for the treatment of ischemic cardiac injury and heart failure wherein cardiac tissue is regenerated thereby improving cardiac function and reducing the morbidity and mortality of patients. Many preclinical and clinical trials have employed various types of stem cells including bone marrow-derived mononuclear cells, skeletal myoblasts, resident cardiac stem cells, mesenchymal stem cells, and endothelial progenitor cells, and these studies have suggested great potential for the clinical application of stem cell therapy for cardiac disease. Here we will review and summarize the current evidence indicating the potential of stem cell therapy, focusing on the clinical trials conducted to date.
Acute myocardial infarction (AMI) is a leading cause of morbidity, mortality, and disability worldwide [1]. Although advances in mechanical interventions for reperfusion and optimal medical therapies have contributed to a dramatic improvement in clinical outcomes, heart failure after AMI remains a major challenge. Although prompt reperfusion therapy could restore the blood flow of an infarct-related coronary artery and reduce the infarct size, the cardiomyocytes lost during the blockage of blood supply cannot be regenerated. Many patients who survive from myocardial infarction (MI) are often left with a significant impairment of left ventricular systolic function due to ischemic cardiomyopathy triggering the remodeling of the left ventricle and fibrosis. Ischemic cardiomyopathy can lead to a poor quality of life and a poor prognosis with high mortality of more than 25% within the first year of diagnosis [23]. Current treatment strategies, including optimal pharmacotherapy, cardiac resynchronization therapy, and implantable cardioverter-defibrillators, slow progression of the MI to heart failure, improve systolic function, and prevent fatal arrhythmias that can cause sudden cardiac death [4567]; however, they cannot stimulate cell regeneration to recover the loss of functional myocytes. Heart transplantation has evolved as the final treatment option for end-stage heart failure patients who have severe symptoms despite several medical therapies, but this option is available to only a few patients because of the lack of donors and the prohibitively high cost [8]. Hence, there is an unmet need to develop methods to reduce damage to cardiomyocytes and regenerate functional myocardium.
While it was previously believed that the heart has negligible intrinsic regenerative capacity and undergoes limited renewal, over the past decades several experimental studies have shown that cardiomyocytes may be able to undergo mitosis [910] and initiate limited regeneration through the recruitment of residential or circulating stem cells to the damaged site in the heart [11]. Clinical studies have supported the use of stem cells as a potential therapeutic modality to regenerate damaged myocardium and restore cardiac function (Table 1)[12]. The stem cells that have been transplanted into damaged myocardium show successful engraftment and generate new cardiomyocytes by transdifferentiation or cell fusion [13]; in addition, the injected stem cells also have paracrine effects [14]. Thus, cell-based therapy using stem cells, which is also known as cellular cardiomyoplasty, has emerged as a strategy to prevent and reverse myocardial injury and to promote myocardial regeneration. Here we review the status of research on cell-based therapy for cardiovascular diseases, focusing on the relevant clinical trials conducted so far.
Stem cells are undifferentiated and primitive cells that can differentiate into multiple cell lineages and have the capacity of renewing themselves through cell division. Precursor or progenitor cells are a type of partially differentiated, usually unipotent, cells that can differentiate into only one cell type, and divide only a limited number of times. These cells have been widely used to regenerate various damaged tissues in animal models [1516]. The basic concept of cell-based therapy for cardiovascular diseases is that stem cells extracted from the source are delivered to the damaged tissue, where they grow and differentiate into cardiomyocytes to heal the damaged heart. The mechanism of myocardial regeneration by stem cells is as follows: 1) Cardiomyocyte regeneration resulting from the transdifferentiation of stem cells [17], 2) Proliferation of endogenous cardiomyocytes by cell fusion with stem cells [18], 3) Paracrine signaling effects of the stem cells [14] and 4) Reduced cardiomyocyte apoptosis by neovascularization (Fig. 1)[19]. Human embryonic stem cells (ESCs) are derived from the inner cell mass of the embryo in the blastocyte stage; ESCs are considered the most pluripotent cells and can differentiate into many cell lineages. The potential for using differentiated human embryonic stem cells to treat damaged heart has long been evident bases on the results of preclinical studies [2021]. However, no human clinical study has been performed so far because of the ethical issues concerning the use of embryos and the risk of teratoma formation. To date, the major cell types used for cell-based cardiomyoplasty are bone marrow-derived mononuclear cells (BMMNCs), skeletal myoblasts, resident cardiac stem cells (CSCs), mesenchymal stem cells, circulating blood-derived progenitor cells, and induced pluripotent stem cells (iPSCs) (Fig. 2).
Bone marrow elements have been shown to contribute to cardiac repair in the infarcted heart in animal studies [22]. Orlic et al. have reported that lineage-negative, c-kit-positive bone marrow-derived cells can differentiate into new cardiomyocytes after MI [11]. In 2002, the first clinical trial of intracoronary, autologous BMMNC transplantation for improving cardiac function and myocardial perfusion in patients after acute MI was reported [23]. After standard therapy for AMI, the BMMNCs aspirated from the ilium of the patients were transplanted during balloon dilatation through a balloon catheter placed into the infarct-related artery. The transplanted BMMNCs led to the repair of the infarcted tissue, likely by improving myocardial perfusion. After this trial, BMMNC transplantation became the most widely studied cell-based therapy for human applications. The Bone Marrow Transfer to Enhance ST-Elevation Infarct Regeneration (BOOST) trial further reported relative improvement in the left ventricular ejection fraction (LVEF) estimated by cardiac magnetic resonance imaging (cMRI) after the transplantation of BMMNCs at 6 months [24]. The Reinfusion of Enriched Progenitor Cells and Infarct Remodeling in Acute Myocardial Infarction (REPAIR-AMI) trial is the largest study on cardiac cell therapy, which was a multicenter double-blind trial of the intracoronary infusion of BMMNCs after successful percutaneous coronary intervention (PCI) for patients with AMI. At 4 months, the absolute improvement in global LVEF was significantly greater in the BMC transplantation group than in the placebo group (mean increase, 5.5% vs 3.0%, P=0.01) and at 1 year, the clinical outcomes including death, recurrence of MI, and revascularization were better in the study group than in the placebo group (23% vs 39%, P=0.01) [25]. The STAR-heart study showed that intracoronary BMC therapy improves ventricular performance, quality of life, and survival not only in the acute phase of MI, but also in chronic ischemic cardiomyopathy [26]. The long-term efficacy and safety of BMMNC transplantation have also been established in many clinical trials. Cao et al. reported that the functional improvement of LV function is maintained during a 4-year follow up in STEMI patients who underwent intracoronary BMMNC transplantation [27]. The long-term clinical outcomes of REPAIR-AMI were also better in the cell therapy group than in the placebo group in terms of death, MI, and re-hospitalization for heart failure in 5 years after the transplantation [28]. However, it is important to note that some recent studies have shown negative results for BMMNC therapy in AMI patients. The Autologous Stem-Cell Transplantation in Acute Myocardial Infarction (ASTAMI) study, a randomized clinical trial (RCT), could not prove the effect of an intracoronary injection of autologous BMMNCs on the global left ventricular function in short-term and long-term follow up [29]. Surder et al. also reported that intracoronary infusion of BMMNCs at either 5 to 7 days or 3 to 4 weeks after AMI did not improve LV function at 4 months of follow-up [30]. Recent RCTs including the FOCUS-CCTRN trial [31], late TIME trial [32], and TIME trial [33] could not show any benefits of cell therapy.
Despite the debates on the efficacy of BMMNC transplantation for short-term or long-term improvement of cardiac function and clinical outcomes, several meta-analyses have documented that the transplantation of BMMNCs was more efficient at improving LV function and decreasing infarct size and remodeling in patients with ischemic heart disease compared to standard therapy [34]; these effects resulted in improved clinical outcomes during long-term follow up in the patients [3536]. A recently published meta-analysis [37] including 48 RCTs enrolling 2,602 patients has shown that BMMNC transplantation resulted in better LVEF (2.92%; 95% confidence interval [CI], 1.91 to 3.92; P<0.00001), smaller infarct size (-2.25%; 95% CI, -3.55 to -0.95; P=0.0007) and left ventricular end-systolic volume (LVESV) (-6.37 mL; 95% CI, -8.95 to -3.80; P<0.00001), and lower left ventricular end-diastolic volume (LVEDV) (-2.26 mL; 95% CI, -4.59 to 0.07; P=0.06), compared to standard therapy. BMMNC therapy was safe and improved the clinical outcomes by reducing the incidence of death, recurrent MI, ventricular arrhythmia, and cerebrovascular accident (CVA) during follow-up. It is possible that definite conclusions about BM-MNC transplantation after MI can be drawn after the ongoing BAMI trial (NCT01569178), which is a multinational, randomized controlled trial with a large study population of up to 3,000 patients.
MSCs are another potential candidate for cell-based cardiomyo-plasty. MSCs are a heterogeneous group of multipotent cells; they have the ability of self-renewal and can also help replenish a variety of specific cell types to aid in tissue repair. MSCs can be found in various tissues such as the bone marrow (BM), muscle, skin, and adipose tissues [38]. They can be obtained from an autologous origin or maybe used from an allogenic origin due to their proposed hypoimmunogenic character in cell transplantation. The efficacy of intravenous allogenic transplantation of human MSCs was suggested by Hara et al. [39]. According to this study, LVEF and pulmonary function were improved in patients who received MSC therapy. Two recently published studies, the POSEIDON trial and the TAC-HFT trial, which used MSCs cultured from the BM aspirate, demonstrated that MSC transplantation improved LV function, ventricular remodeling, and the functional capacity and quality of life of the patients [4041]. Another feasible source of MSCs is the adipose tissue; stem cells from this tissue are called adipose tissue-derived stem cells (ADSCs). The APOLLO trial, the first human trial conducted using ADSCs for the treatment of patients with STEMI showed a trend toward improving cardiac function, accompanied by a significant improvement of the perfusion defect and a 50% reduction in myocardial scar formation after stem cell infusion [42]. However, MSC preparations comprise subsets of cells with different phenotypes and functions, and it is not yet fully elucidated how this heterogeneity could affect therapeutic efficacy and safety. Most recently, the Cardiopoietic Stem Cell Therapy in Heart Failure trial, named as the C-CURE trial, was conducted in patients with ischemic heart failure using lineage-specific MSCs; these MSCs were pre-primed using cardiogenic cocktails, which can trigger the expression and nuclear translocation of cardiac transcription factors, before endomyocardial injection of the MSCs into areas of the dysfunctional myocardium [43]. This trial showed significantly improved cardiac function and exercise tolerance in the treated patients compared to the patients that received conventional treatment. MSCs thus present advantages over the other types of stem cells. Further, in the case of MSCs, it is possible to use an allogenic graft. The allogenic cell product, that can be made available as "off the shelf," would be more cost effective and easier to administer and could potentially allow the delivery of greater numbers of cells than autologous cell therapy.
For decades, the human heart was recognized as a terminally differentiated organ; however, several studies have reported that a population of stem cells within the myocardium can differentiate into cardiac myocytes [444546]. Despite the unclear origin of CSCs, they can be isolated from the heart tissue and expanded ex vivo for use in cell-based therapy. In the Stem Cell Infusion in Patients with Ischemic Cardiomyopathy (SCIPIO) trial, autologous CSCs were isolated from the right appendage during cardiac surgery and re-infused intracoronarily. No adverse effect was observed and the LVEF increased in the treated patients along with an improvement in the functions and quality of life at 4 months after therapy [47]. In addition, the Cardiosphere-Derived Autologous Stem Cells to reverse ventricular dysfunction (CADUCEUS) study, in which the stem cells were obtained by percutaneous endomyocardial biopsies, showed that viable heart mass and regional contractility, analyzed by cMRI, increased in the treated patients [4849].
Circulating blood-derived progenitor cells, which are mainly composed of endothelial progenitor cells, are suitable for use in cell-based therapy. Granulocyte colony stimulating factor (G-CSF) is usually used for the mobilization of stem cells and stem cell factors; these cells and factors are then harvested from peripheral blood without invasive BM cell collection. In the MAGIC cell trial, Kang et al. reported that G-CSF therapy with intracoronary infusion of peripheral blood stem cells improved cardiac function and promoted angiogenesis in patients with MI in short-term and long-term follow up [5051]. Next, the efficacy and safety between BM-MNCs and circulating blood-derived progenitor cells were compared in the TOPCARE-AMI trial. Both the cell therapies significantly improved LVEF and there was no difference between the two groups [52].
Skeletal myoblasts are known as satellite cells, which are reservoirs of regenerative cells for skeletal muscle tissues. These myoblasts can differentiate into myotubes and myocytes in response to injury. Experimental studies have shown that skeletal myoblasts can engraft in post-infarction scars in the heart, differentiate into myotubes, and improve LV function [53]. Dib et al. performed a phase I nonrandomized pilot study that demonstrated survival, feasibility, and safety of autologous myoblast transplantation into the infarcted myocardium [54]. However, the early clinical studies of myoblast transplantation in the post-infarct myocardium suggested the possibility of a proarrhythmic effect [5556]. Two trials, the MAGIC trial [57] and the SEISMIC trial [58], showed no significant changes in terms of global and regional LV function in skeletal myoblast-treated patients, and the MARVEL trial was prematurely terminated due to the occurrence of sustained ventricular tachycardia [59]. These multiple negative results and concerns about fatal arrhythmia make it unlikely that myoblast-based cell therapy will be further pursued.
As mentioned above, embryonic stem cells from the human embryo are pluripotent cells that can differentiate into cardiomyocytes, but their use is limited due to ethical issues and the risk of teratoma formation. One possible solution to these problems is the use of induced pluripotent stem cells. In 2006, Takahashi and Yamanake demonstrated the induction of pluripotent stem cells from adult human fibroblasts by introducing four factors (Oct3/4, Sox2, c-Myc, and Klf4) [60]. The generation of pluripotent cells from different adult somatic cell types is referred to as reprogramming. Currently, the reprogramming of somatic cells is an inefficient process that needs to be refined before it can be applied in clinical trials. Therefore, nowadays, iPSCs represent a novel source of cells for regeneration therapy of the heart, but are not ready for application in human hearts yet.
Current concepts of cell-based therapies are focused on the facilitation of endogenous repair processes by transplanted stem cells rather than through the actual regeneration of the lost cardiac cells [61]. The observation of paracrine effects of stem cells, which have been shown to trigger angiogenesis, prevent apoptosis of myocardial cells, induce the proliferation of endogenous cardiomyocytes and recruit resident CSCs, has stimulated an emerging concept of myocardial regeneration therapy [14]. In situ activation of paracrine signaling to initiate effective endogenous myocardial repair without stem cell transplantation would help avoid some major concerns associated with stem cell transplantation, such as delayed injection after acute injury for stem cell preparation, the high cost of the procedure, issues concerning the most effective route of delivery, and insufficient retention of the transplanted cells in damaged tissue. The paracrine effects of several growth factors such as the vascular endothelial growth factor, fibroblast growth factor, granulocyte colony stimulating factor, granulocyte-macrophage colony-stimulating factor, erythropoietin, insulin-like growth factor-1, and hepatocyte growth factor have been studied for regeneration of damaged myocardium. Ellison et al. reported that the intracoronary injection of a combination of insulin-like growth factor-1 and hepatocyte growth factor in the infarct-related coronary artery reduced ventricular remodeling and improved myocardial regeneration in an animal study [62]. Other growth factors can also be used to promote cardiac regeneration and improve cardiac function. However, to date, no randomized clinical trial confirming the clinical benefits of growth factor injection for the treatment of ischemic heart failure has been published.
Stem cell therapy is a novel and promising therapeutic modality for patients with significant cardiac dysfunction. Although many experimental studies and clinical trials have proven the feasibility, safety, and efficacy of cell-based cardiomyoplasty, the mechanisms underlying the regeneration of damaged cardiac tissues by stem cells are not fully understood. There are also many issues related to the use of stem cells, including the eligibility of patients, optimal timing of cell transfer, dosage, method of cell administration, and selection of the correct type of cells, that remain to be solved. Therefore, further research in the form of preclinical studies and large randomized clinical trials is required to optimize cell-based therapy for cardiovascular diseases.
Figures and Tables
![]() | Fig. 1Mechanism of stem cell therapy for myocardial regeneration and improvement of cardiac function. |
Table 1
Summary of clinical trials on stem cell therapy
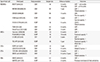
BMMNCs, bone marrow derived stem cells; AMI, acute myocardial infarction; LVEF, left ventricular ejection fraction; CHF, congestive heart failure; MSCs, mesenchymal stem cells; ICMP, ischemic cardiomyopathy; CSCs, cardiac stem cells; CPCs, circulating blood derived progenitor cells; SMs, skeletal myoblasts.
ACKNOWLEDGMENTS
The author is grateful to Hyung-Woo Chang (Cardiac surgeon in Sejong General Hospital) for his assistance in the illustration of Fig. 2.
References
1. Mozaffarian D, Benjamin EJ, Go AS, Arnett DK, Blaha MJ, Cushman M, et al. Heart disease and stroke statistics--2015 update: a report from the American Heart Association. Circulation. 2015; 131:e29–e322.
2. Alter DA, Ko DT, Tu JV, Stukel TA, Lee DS, Laupacis A, et al. The average lifespan of patients discharged from hospital with heart failure. J Gen Intern Med. 2012; 27:1171–1179.


3. Lee DS, Schull MJ, Alter DA, Austin PC, Laupacis A, Chong A, et al. Early deaths in patients with heart failure discharged from the emergency department: a population-based analysis. Circ Heart Fail. 2010; 3:228–235.


4. Bardy GH, Lee KL, Mark DB, Poole JE, Packer DL, Boineau R, et al. Amiodarone or an implantable cardioverter-defibrillator for congestive heart failure. N Engl J Med. 2005; 352:225–237.


5. Abraham WT, Fisher WG, Smith AL, Delurgio DB, Leon AR, Loh E, et al. Cardiac resynchronization in chronic heart failure. N Engl J Med. 2002; 346:1845–1853.


7. McMurray JJ. Clinical practice. Systolic heart failure. N Engl J Med. 2010; 362:228–238.
8. Taylor DO, Edwards LB, Boucek MM, Trulock EP, Aurora P, Christie J, et al. Registry of the International Society for Heart and Lung Transplantation: twenty-fourth official adult heart transplant report--2007. J Heart Lung Transplant. 2007; 26:769–781.


9. Beltrami AP, Urbanek K, Kajstura J, Yan SM, Finato N, Bussani R, et al. Evidence that human cardiac myocytes divide after myocardial infarction. N Engl J Med. 2001; 344:1750–1757.


10. Kajstura J, Leri A, Finato N, Di Loreto C, Beltrami CA, Anversa P. Myocyte proliferation in end-stage cardiac failure in humans. Proc Natl Acad Sci U S A. 1998; 95:8801–8805.


11. Orlic D, Kajstura J, Chimenti S, Jakoniuk I, Anderson SM, Li B, et al. Bone marrow cells regenerate infarcted myocardium. Nature. 2001; 410:701–705.


12. Ptaszek LM, Mansour M, Ruskin JN, Chien KR. Towards regenerative therapy for cardiac disease. Lancet. 2012; 379:933–942.


13. Stamm C, Westphal B, Kleine HD, Petzsch M, Kittner C, Klinge H, et al. Autologous bone-marrow stem-cell transplantation for myocardial regeneration. Lancet. 2003; 361:45–46.


14. Gnecchi M, Zhang Z, Ni A, Dzau VJ. Paracrine mechanisms in adult stem cell signaling and therapy. Circ Res. 2008; 103:1204–1219.


15. Friedenstein AJ, Gorskaja JF, Kulagina NN. Fibroblast precursors in normal and irradiated mouse hematopoietic organs. Exp Hematol. 1976; 4:267–274.
16. Chen TH. Tissue regeneration: from synthetic scaffolds to self-organizing morphogenesis. Curr Stem Cell Res Ther. 2014; 9:432–443.


17. Kehat I, Kenyagin-Karsenti D, Snir M, Segev H, Amit M, Gepstein A, et al. Human embryonic stem cells can differentiate into myocytes with structural and functional properties of cardiomyocytes. J Clin Invest. 2001; 108:407–414.


18. Oh H, Bradfute SB, Gallardo TD, Nakamura T, Gaussin V, Mishina Y, et al. Cardiac progenitor cells from adult myocardium: homing, differentiation, and fusion after infarction. Proc Natl Acad Sci U S A. 2003; 100:12313–12318.


19. Kocher AA, Schuster MD, Szabolcs MJ, Takuma S, Burkhoff D, Wang J, et al. Neovascularization of ischemic myocardium by human bone-marrow-derived angioblasts prevents cardiomyocyte apoptosis, reduces remodeling and improves cardiac function. Nat Med. 2001; 7:430–436.


20. Vittet D, Prandini MH, Berthier R, Schweitzer A, Martin-Sisteron H, Uzan G, et al. Embryonic stem cells differentiate in vitro to endothelial cells through successive maturation steps. Blood. 1996; 88:3424–3431.


21. Yamashita J, Itoh H, Hirashima M, Ogawa M, Nishikawa S, Yurugi T, et al. Flk1-positive cells derived from embryonic stem cells serve as vascular progenitors. Nature. 2000; 408:92–96.


22. Jackson KA, Majka SM, Wang H, Pocius J, Hartley CJ, Majesky MW, et al. Regeneration of ischemic cardiac muscle and vascular endothelium by adult stem cells. J Clin Invest. 2001; 107:1395–1402.


23. Strauer BE, Brehm M, Zeus T, Kostering M, Hernandez A, Sorg RV, et al. Repair of infarcted myocardium by autologous intracoronary mononuclear bone marrow cell transplantation in humans. Circulation. 2002; 106:1913–1918.


24. Wollert KC, Meyer GP, Lotz J, Ringes-Lichtenberg S, Lippolt P, Breidenbach C, et al. Intracoronary autologous bone-marrow cell transfer after myocardial infarction: the BOOST randomised controlled clinical trial. Lancet. 2004; 364:141–148.


25. Schachinger V, Erbs S, Elsasser A, Haberbosch W, Hambrecht R, Holschermann H, et al. Intracoronary bone marrow-derived progenitor cells in acute myocardial infarction. N Engl J Med. 2006; 355:1210–1221.


26. Strauer BE, Yousef M, Schannwell CM. The acute and long-term effects of intracoronary Stem cell Transplantation in 191 patients with chronic heARt failure: the STAR-heart study. Eur J Heart Fail. 2010; 12:721–729.


27. Cao F, Sun D, Li C, Narsinh K, Zhao L, Li X, et al. Long-term myocardial functional improvement after autologous bone marrow mononuclear cells transplantation in patients with ST-segment elevation myocardial infarction: 4 years follow-up. Eur Heart J. 2009; 30:1986–1994.


28. Assmus B, Leistner DM, Schachinger V, Erbs S, Elsasser A, Haberbosch W, et al. Long-term clinical outcome after intracoronary application of bone marrow-derived mononuclear cells for acute myocardial infarction: migratory capacity of administered cells determines event-free survival. Eur Heart J. 2014; 35:1275–1283.


29. Beitnes JO, Hopp E, Lunde K, Solheim S, Arnesen H, Brinchmann JE, et al. Long-term results after intracoronary injection of autologous mononuclear bone marrow cells in acute myocardial infarction: the ASTAMI randomised, controlled study. Heart. 2009; 95:1983–1989.


30. Surder D, Manka R, Lo Cicero V, Moccetti T, Rufibach K, Soncin S, et al. Intracoronary injection of bone marrow-derived mononuclear cells early or late after acute myocardial infarction: effects on global left ventricular function. Circulation. 2013; 127:1968–1979.


31. Perin EC, Willerson JT, Pepine CJ, Henry TD, Ellis SG, Zhao DX, et al. Effect of transendocardial delivery of autologous bone marrow mononuclear cells on functional capacity, left ventricular function, and perfusion in chronic heart failure: the FOCUS-CCTRN trial. JAMA. 2012; 307:1717–1726.


32. Traverse JH, Henry TD, Ellis SG, Pepine CJ, Willerson JT, Zhao DX, et al. Effect of intracoronary delivery of autologous bone marrow mononuclear cells 2 to 3 weeks following acute myocardial infarction on left ventricular function: the LateTIME randomized trial. JAMA. 2011; 306:2110–2119.


33. Traverse JH, Henry TD, Pepine CJ, Willerson JT, Zhao DX, Ellis SG, et al. Effect of the use and timing of bone marrow mononuclear cell delivery on left ventricular function after acute myocardial infarction: the TIME randomized trial. JAMA. 2012; 308:2380–2389.
34. Lipinski MJ, Biondi-Zoccai GG, Abbate A, Khianey R, Sheiban I, Bartunek J, et al. Impact of intracoronary cell therapy on left ventricular function in the setting of acute myocardial infarction: a collaborative systematic review and meta-analysis of controlled clinical trials. J Am Coll Cardiol. 2007; 50:1761–1767.


35. Delewi R, Andriessen A, Tijssen JG, Zijlstra F, Piek JJ, Hirsch A. Impact of intracoronary cell therapy on left ventricular function in the setting of acute myocardial infarction: a meta-analysis of randomised controlled clinical trials. Heart. 2013; 99:225–232.


36. Jeevanantham V, Butler M, Saad A, Abdel-Latif A, Zuba-Surma EK, Dawn B. Adult bone marrow cell therapy improves survival and induces long-term improvement in cardiac parameters: a systematic review and meta-analysis. Circulation. 2012; 126:551–568.


37. Afzal MR, Samanta A, Shah ZI, Jeevanantham V, Abdel-Latif A, Zuba-Surma EK, et al. Adult bone marrow cell therapy for ischemic heart disease: evidence and insights from randomized controlled trials. Circ Res. 2015.
38. Pittenger MF, Martin BJ. Mesenchymal stem cells and their potential as cardiac therapeutics. Circ Res. 2004; 95:9–20.


39. Hare JM, Traverse JH, Henry TD, Dib N, Strumpf RK, Schulman SP, et al. A randomized, double-blind, placebo-controlled, dose-escalation study of intravenous adult human mesenchymal stem cells (prochymal) after acute myocardial infarction. J Am Coll Cardiol. 2009; 54:2277–2286.


40. Heldman AW, DiFede DL, Fishman JE, Zambrano JP, Trachtenberg BH, Karantalis V, et al. Transendocardial mesenchymal stem cells and mononuclear bone marrow cells for ischemic cardiomyopathy: the TAC-HFT randomized trial. JAMA. 2014; 311:62–73.


41. Hare JM, Fishman JE, Gerstenblith G, DiFede Velazquez DL, Zambrano JP, Suncion VY, et al. Comparison of allogeneic vs autologous bone marrow-derived mesenchymal stem cells delivered by transendocardial injection in patients with ischemic cardiomyopathy: the POSEIDON randomized trial. JAMA. 2012; 308:2369–2379.


42. Houtgraaf JH, den Dekker WK, van Dalen BM, Springeling T, de Jong R, van Geuns RJ, et al. First experience in humans using adipose tissue-derived regenerative cells in the treatment of patients with ST-segment elevation myocardial infarction. J Am Coll Cardiol. 2012; 59:539–540.


43. Bartunek J, Behfar A, Dolatabadi D, Vanderheyden M, Ostojic M, Dens J, et al. Cardiopoietic stem cell therapy in heart failure: the C-CURE (Cardiopoietic stem cell therapy in heart failure) multicenter randomized trial with lineage-specified biologics. J Am Coll Cardiol. 2013; 61:2329–2338.
44. Fazel S, Cimini M, Chen L, Li S, Angoulvant D, Fedak P, et al. Cardioprotective c-kit+ cells are from the bone marrow and regulate the myocardial balance of angiogenic cytokines. J Clin Invest. 2006; 116:1865–1877.


45. Tang YL, Wang YJ, Chen LJ, Pan YH, Zhang L, Weintraub NL. Cardiac-derived stem cell-based therapy for heart failure: progress and clinical applications. Exp Biol Med (Maywood). 2013; 238:294–300.


46. De Angelis A, Piegari E, Cappetta D, Marino L, Filippelli A, Berrino L, et al. Anthracycline cardiomyopathy is mediated by depletion of the cardiac stem cell pool and is rescued by restoration of progenitor cell function. Circulation. 2010; 121:276–292.


47. Bolli R, Chugh AR, D'Amario D, Loughran JH, Stoddard MF, Ikram S, et al. Cardiac stem cells in patients with ischaemic cardiomyopathy (SCIPIO): initial results of a randomised phase 1 trial. Lancet. 2011; 378:1847–1857.


48. Malliaras K, Makkar RR, Smith RR, Cheng K, Wu E, Bonow RO, et al. Intracoronary cardiosphere-derived cells after myocardial infarction: evidence of therapeutic regeneration in the final 1-year results of the CADUCEUS trial (cardiosphere-derived autologous stem cells to reverse ventricular dysfunction). J Am Coll Cardiol. 2014; 63:110–122.
49. Makkar RR, Smith RR, Cheng K, Malliaras K, Thomson LE, Berman D, et al. Intracoronary cardiosphere-derived cells for heart regeneration after myocardial infarction (CADUCEUS): a prospective, randomised phase 1 trial. Lancet. 2012; 379:895–904.


50. Kang HJ, Kim HS, Zhang SY, Park KW, Cho HJ, Koo BK, et al. Effects of intracoronary infusion of peripheral blood stem-cells mobilised with granulocyte-colony stimulating factor on left ventricular systolic function and restenosis after coronary stenting in myocardial infarction: the MAGIC cell randomised clinical trial. Lancet. 2004; 363:751–756.


51. Kang HJ, Kim MK, Lee HY, Park KW, Lee W, Cho YS, et al. Five-year results of intracoronary infusion of the mobilized peripheral blood stem cells by granulocyte colony-stimulating factor in patients with myocardial infarction. Eur Heart J. 2012; 33:3062–3069.


52. Assmus B, Schachinger V, Teupe C, Britten M, Lehmann R, Dobert N, et al. Transplantation of Progenitor Cells and Regeneration Enhancement in Acute Myocardial Infarction (TOPCARE-AMI). Circulation. 2002; 106:3009–3017.


53. Dowell JD, Rubart M, Pasumarthi KB, Soonpaa MH, Field LJ. Myocyte and myogenic stem cell transplantation in the heart. Cardiovasc Res. 2003; 58:336–350.


54. Dib N, Michler RE, Pagani FD, Wright S, Kereiakes DJ, Lengerich R, et al. Safety and feasibility of autologous myoblast transplantation in patients with ischemic cardiomyopathy: four-year follow-up. Circulation. 2005; 112:1748–1755.


55. Menasche P, Hagege AA, Vilquin JT, Desnos M, Abergel E, Pouzet B, et al. Autologous skeletal myoblast transplantation for severe postinfarction left ventricular dysfunction. J Am Coll Cardiol. 2003; 41:1078–1083.


56. Siminiak T, Kalawski R, Fiszer D, Jerzykowska O, Rzezniczak J, Rozwadowska N, et al. Autologous skeletal myoblast transplantation for the treatment of postinfarction myocardial injury: phase I clinical study with 12 months of follow-up. Am Heart J. 2004; 148:531–537.


57. Menasche P, Alfieri O, Janssens S, McKenna W, Reichenspurner H, Trinquart L, et al. The Myoblast Autologous Grafting in Ischemic Cardiomyopathy (MAGIC) trial: first randomized placebo-controlled study of myoblast transplantation. Circulation. 2008; 117:1189–1200.


58. Duckers HJ, Houtgraaf J, Hehrlein C, Schofer J, Waltenberger J, Gershlick A, et al. Final results of a phase IIa, randomised, open-label trial to evaluate the percutaneous intramyocardial transplantation of autologous skeletal myoblasts in congestive heart failure patients: the SEISMIC trial. EuroIntervention. 2011; 6:805–812.


59. Povsic TJ, O'Connor CM, Henry T, Taussig A, Kereiakes DJ, Fortuin FD, et al. A double-blind, randomized, controlled, multicenter study to assess the safety and cardiovascular effects of skeletal myoblast implantation by catheter delivery in patients with chronic heart failure after myocardial infarction. Am Heart J. 2011; 162:654–662.e1.


60. Takahashi K, Tanabe K, Ohnuki M, Narita M, Ichisaka T, Tomoda K, et al. Induction of pluripotent stem cells from adult human fibroblasts by defined factors. Cell. 2007; 131:861–872.


61. Chou SH, Lin SZ, Kuo WW, Pai P, Lin JY, Lai CH, et al. Mesenchymal stem cell insights: prospects in cardiovascular therapy. Cell Transplant. 2014; 23:513–529.


62. Ellison GM, Torella D, Dellegrottaglie S, Perez-Martinez C, Perez de Prado A, Vicinanza C, et al. Endogenous cardiac stem cell activation by insulin-like growth factor-1/hepatocyte growth factor intracoronary injection fosters survival and regeneration of the infarcted pig heart. J Am Coll Cardiol. 2011; 58:977–986.

