Abstract
Objective
The aim of this study was to determine if human PDL cells can produce osteoclastogenic mRNA and examine how compressive stress affects the expression of osteoclastogenic mRNA in human PDL cells.
Methods
Human PDL cells were obtained from biscupids extracted for orthodontic treatment. The compressive force was adjusted by increasing the number of cover glasses. PDL cells were subjected to a compressive force of 0.5, 1.0, 2.0, 3.0 or 4.0 g/cm2 for 0.5, 1.5, 6, 24 or 48 hours. Reverse transcription polymerase chain reaction (RT-PCR) analysis was performed to examine levels of M-CSF, IL-1β, RANKL, OPG mRNA expression.
Results
Human PDL cells could produce M-CSF mRNA. Human PDL cells under compressive stress showed increased M-CSF, IL-1β and RANKL mRNAs expression in a force (up to 2 g/cm2) and time-dependent manner. However, OPG mRNA expression was constant regardless of the level and duration of stress.
Conclusions
Continuous compressive stress induced the mRNA expression of osteoclastogenic cytokines including M-CSF, RANKL, IL-1β in PDL cells. Together with an unchanged OPG mRNA level, these results suggest that compressive stress-induced osteoclastogenesis in vivo is partly controlled by M-CSF, RANKL and IL-1β expression in PDL cells.
Orthodontic tooth movement occurs during the sequential periodontal tissue remodeling, especially alveolar bone, induced by therapeutic mechanical stress.1 It is a generally accepted that the periodontal ligament tissue plays a key role in tooth movement as a response to an applied mechanical stress, due to paradental tissue remodeling including bone resorption and formation. "Ankylosed teeth", in which the cementum of the tooth root is connected directly to the alveolar bone, cannot be moved by therapeutic mechanical stress due to the lack of a periodontal ligament.2
Osteoclastogenesis has been an important subject in the field of bone cell biology for a long time. Recently, the molecular determinants of osteoclastogenesis were identified. Membrane-bound proteins, receptor activator of nuclear factor κB ligand (RANKL), and soluble macrophage colony-stimulating factor (M-CSF) are considered essential factors for osteoclastogenesis produced by osteoblasts and bone marrow stromal cells.3-6 In contrast, osteoprotegerin (OPG), a soluble tumor necrosis factor (TNF) receptor homolog, was found to inhibit osteclastogenesis by competing with the binding of RANKL to the RANK (receptor of RANKL).7
Cultured cells derived from the PDL, mostly fibroblasts, can express and produce the cytokines associated with osteoclastogenesis. Hasegawa et al8 reported that PDL cells derived from deciduous and permanent teeth synthesized both RANKL and OPG, and could regulate the differentiation of osteoclasts. It was also reported that PDL cells secrete M-CSF in response to TNF-α stimulation.9 Wada et al10 showed that human PDL fibroblastic cells have the capacity to produce and secrete OPG. Furthermore, it was reported that inflammatory cytokines, such as PGE2, IL-1α, IL-1β, IL-6 and TNF-α, are produced by mechanically stimulated PDL cells.11-13
Previous reports showed that PDL cells can participate in osteoclastogenesis but the production of the related cytokines in PDL cells has not been fully characterized. In particular, for M-CSF and IL-1β, their production in mechanically stimulated PDL cells has not been documented. This study examined the mode of osteoclastogenetic cytokine production including MCSF, RANKL, IL-1β and OPG in PDL cells in response to compressive mechanical stimulation.
Biscupids extracted from 5 patients for orthodontic reasons were used in this study. Informed consent was obtained from all volunteers. Immediately after extraction, the teeth were placed in α-MEM containing 15% FBS (Sigma-aldrich, St. Louis, MO, USA) and 3-fold-reinforced antibiotics (Antibiotics and Antimycotics, Gibco BRL, Grand Island, NY, USA) in a 50 ml conical tube (Corning, NY, USA). Using a No. 15 surgical blade, a piece of PDL was obtained exclusively from the middle of the tooth roots in order to exclude the intermixture of gingivae and dental pulp.9,10 The PDL tissue obtained was treated with 1.10 unit/ml dispase (Gibco BRL, Grand Island, NY, USA) and 264 unit/ml collagenase (Collagenase Type II; Gibco BRL, Grand Island, NY, USA) for 1 hour at 37℃. After washing with α-MEM, ligament samples were cultured on a 100 mm primary culture dish (Corning, NY, USA) in α-MEM containing 15% FBS and antibiotics. The cells proliferating from the extracts were passaged. For all experiments, PDL cells from the 4th to 8th passages were used.
All PDL cells were cultured in α-MEM containing 15% FBS and antibiotics at 37℃ in a 5% CO2 incubator. The culture medium was changed twice a week throughout the experiment.
After sufficient cultivation, PDL cells from each patient were transferred to 6-well plates. Each well of a 6-well plate contained 1 × 106 PDL cells. Two days later, the PDL cells were allowed to adhere to the well plate base, and PDL cells were compressed continuously using the uniform compression method illustrated in Fig 1.
Briefly, round-shaped cover glasses (30 mm diameter, Marienfeld, Louda-Könlgshofen, Germany) were placed over a confluent cell layer in each well of a 6-well plate. Each cover glass weighed 0.245 gm. The compressive force was adjusted by increasing or decreasing the number of cover glasses. Because the diameter of a well in a 6-well culture plate is approximately 35 mm, the periphery of each well was not fully covered by the cover glass. However, the uncovered area was minor and did not appear to affect the results of the study. The PDL cells were subjected to a compressive force of 0.5, 1, 2, 3 or 4 g/cm2 (total given force was 3.43, 7.015, 14.21, 21.315, 28.175 gm, which was 0.49, 1.01, 2.01, 3.01, 3.99 g/cm2) for 0.5, 1.5, 6, 24 or 48 hours. The PDL cells without compressive stimulation served as the control group.
After each culture period, the total RNA was extracted from each culture. After removing the culture medium, the PDL cells from each well were homogenized using Trizol reagent (Invitrogen Co., Carlsbad, CA, USA). After homogenization, 0.2 ml of chloroform (Sigma-aldrich, St. Louis, MO, USA) per 1 ml of Trizol reagent was added. The samples were centrifuged at 4℃, 12,000 rpm for 15 minutes. An aqueous phase was transferred to a fresh tube, and 0.5 ml of isopropyl alcohol (Sigma-aldrich, St. Louis, MO, USA) per 1 ml of Trizol reagent was added. The samples were centrifuged again under the same conditions. Removing the supernatant, the RNA pellets were washed with 75% alcohol (Sigma-aldrich, St. Louis, MO, USA), and dried for 5 to 10 minutes. The RNA was dissolved in 0.1% diethyl pyrocarbonate (DEPC) water (Fermentas, Glen Burnie, MD, USA).
For complementary DNA synthesis, a mixture of 500 ng mRNA, 2µl of 10µM Oligo dT (Fermentas, Glen Burnie, MD, USA) and 3µl DEPC water was incubated at 80℃ for 3 minutes, and chilled on ice for 2 minutes. Subsequently, 4µl of 5X RT buffer (Fermentas, Glen Burnie, MD, USA), 20 units of RNase inhibitor (Fermentas, Glen Burnie, MD, USA), 200 units of RevertAid™ M-MuLV RT (Fermentas Inc., Glen Burnie, MD, USA) and 4µl of 2.5 mM dNTP Mix (Fermentas, Glen Burnie, MD, USA) were added to the mixture and incubated at 42℃ for 90 minutes.
First-stranded complementary DNA was subjected to polymerase chain reaction (PCR) amplification using gene specific PCR primers. PCR for M-CSF and IL-1β was carried out using a GeneXP™ kit (Seegene, Seoul, Korea). Each 10µl reaction mixture contained 2µl of 5X Human CYTO-X DSO™ primer, 5µl of 2X master mix and 20 ng of cDNA. Each cycle consisted of the following: heat denaturation at 94℃ for 30s, annealing at 63℃ for 90s and extension at 72℃ for 90s. PCR amplification can only start if all two parts of the DSO™ (Dual Specificity Oligonucleotide; Seegene, Seoul, Korea) primer bind to cDNA, which result in higher specificity and sensitivity than when using the usual primer.
In PCR for RANKL and OPG, 2X PCR Master Mix (Fermentas, Glen Burnie, MD, USA) was used for the reaction. Each 50µl reaction mixture contained 20 pmol of the sense and antisense PCR primers, 200 ng of cDNA and 25µl of 2X PCR Master Mix. Each cycle consisted of the following: denaturation at 94℃ for 30s, annealing at a temperature optimized for each primer pair (Table 1) for 90s and extension at 74℃ for 90s.
The PCR products were electrophoresed and visualized on a 2% agarose gel containing ethidium bromide with UV light illumination. The relative intensity of the gel bands was measured using Scion Image (Scion, Frederick, MD, USA) for Windows XP.
The expression of M-CSF and IL-1β mRNAs in human PDL cells was assessed after applying various mechanical compressive stresses for various durations. PDL cells under compression showed an increase in M-CSF and IL-1β mRNAs expression in a force-dependent manner up to 2 g/cm2, but the expression decreased after applying 3 g/cm2. The expression of M-CSF and IL-1β mRNAs in the experimental groups reached a maximum of 1.7- and 1.5-fold, respectively, at a compressive load of 2 g/cm2, and were significantly different from those of the control group which was without compressive stress (0 g/cm2) (Fig 2). M-CSF and IL-1β mRNAs expression was similar to that of the control group at 0.5 hour of 2 g/cm2 compression but a time-dependent increase was evident after 6 and 24 hours, respectively, for up to 48 hours (Fig 3).
Expression of RANKL and OPG mRNA in human PDL cells was assessed after applying various compressive mechanical stresses for various durations. PDL cells under compression showed increased RANKL mRNA expression in a force-dependent manner up to 2 g/cm2, but expression was decreased after 3 g/cm2. The expression of RANKL mRNA in the experimental groups reached a maximum of 2.1-fold at 2 g/cm2, and was significantly different from those of the control group (Fig 4). RANKL mRNA expression was similar to that of the control group after 0.5 hours of 2 g/cm2 compression. However, an increase was evident after 6 hours, and it increased in a time-dependent manner for up to 48 hours (Fig 5). In contrast, OPG mRNA expression did not change, regardless of the amount of compressive force applied and the duration of compression (Figs 4 and 5).
In this study, human PDL cells were cultured under various levels of compressive stress for different periods to determine the effects of mechanical stress on the expression of M-CSF mRNA in PDL cells. A RT-PCR assay was used to analyze target mRNA expression.
Generally, PDL includes multipopulation cells consisting mainly of fibroblasts with high alkaline phosphatase activity,14 and fibroblasts from PDL share many physiological characteristics with osteoblasts.15,16 Some studies already described methods to isolate PDL cells from PDL, and an almost identical method was used in this study. It is possible that osteoblastic cells in the PDL subpopulation transduce mechanical stress. However, the effects of other types of PDL cells were negligible in this experiment because most cells in the population in this culture system were spindle-shaped fibroblastic cells.
Many researchers have already examined the effects of mechanical stress on PDL cells. Various methods have been used to impart mechanical stress to cultured cells in the manner of tension, compression and fluid shear stress, etc.17-26 Among the manner of mechanical stress, compressive stress was used to confirm the role of PDL cell in the tissue remodeling process of the compressive side during orthodontic tooth movement with particular focus on osteoclastogenesis. Hydrostatic compression,22,23 reverse-tension compression,24 and direct contact compression methods19,25,26 were used to provide compressive stress on cultured cells. The direct contact compression method appeared to mimic most in vivo systems in orthodontic treatment because a static compressive force is applied to the tooth in daily orthodontic practice and that PDL tissue is compressed directly between two hard structures, bone and cementum. Therefore, this study adopted the direct contact compression method, which provides a compression force by squeezing the cells between two hard surfaces, the culture dish surface and cover glass.
Kanzaki et al.19 used glass cylinder and lead granules to provide compression stimulation. They placed a glass cylinder over a confluent cultured PDL cell layer and adjusted the compressive force by adding lead granules to the cylinder. Similarly, Yamaguchi et al.25 applied compressive stimulation by placing a 30 mm cell disk over a confluent cell layer followed by a glass cylinder on top of it. They also used lead granules to control the amount of compressive force by placing them into the glass cylinder. The present method used only cover glasses in order to simplify the equipment. In addition, by stacking same diameter glasses, a more uniform force could be delivered to the underlying cells than the lead granules, which can roll around in the glass cylinder. In addition, the cover glass weight was small enough (0.245 g) to control the force in a more accurate manner.
The M-CSF and RANKL evaluated in this study are essential factors for osteoclastogenesis produced by osteoblasts and bone marrow stromal cells.3-6 M-CSF is essential for not only the proliferation of osteoclast progenitors, but also for differentiation into mature osteoclasts and survival in vitro.27 In contrast, OPG was reported to inhibit osteclastogenesis competing with the binding of RANKL to the RANK. IL-1β is a proinflammatory cytokine that stimulates M-CSF and PGE2 production,28 and up-regulated PGE2 mediates RANKL expression in PDL cells under a static compressive stress.19
However, there is some controversy regarding the effects of M-CSF in osteoclastogenesis. Although M-CSF is an essential factor for the formation of osteoclasts, it also has been reported that the addition of M-CSF to a culture inhibits osteoclast formation. Udagawa et al.3 reported that M-CSF, while stimulating murine osteoclast survival, did not induce pit-forming activity. Perkins et al.29 showed that the addition of exogenous M-CSF to a co-culture of mouse spleen cells and ST2 cells caused a dose-dependent decrease in the number of osteoclasts formed, and was accompanied by an increase in the number of macrophages. Recently, Yasuda et al.30 reported that a high concentration of M-CSF (> 40 ng/ml) suppressed osteoclast formation in murine spleen cell cultures stimulated with soluble RANKL. Considering that these studies examined osteoclast formation in animal models, species differences and different requirements for M-CSF between the different culture systems can account for these divergent findings.
Through RT-PCR assays, PDL cells under a compressive stress showed increased M-CSF, IL-1β and RANKL mRNAs expression in a force- (up to 2 g/cm2) and time-dependent manner, but OPG mRNA expression was constant regardless of the amount of compressive stress or duration of compression. This can be explained by the fact that PDL cells under compressive stress regulate bone remodeling through an increase in the production of osteoclastogenetic cytokines but not OPG. In addition, compressive stress-induced intracellular changes are not involved in OPG mRNA expression. However, it was reported that tension type stimulation up-regulates OPG mRNA expression.31,32 Therefore compression and tension stimulation appears to have separate signal transduction pathways.
These results partly coincide with previous reports. Kanzaki et al.19 reported that compressive stress up to 2 g/cm2 up-regulated RANKL mRNA in a time-dependent manner. They also measured the level of OPG and reported no change. Nishijima et al.26 examined levels of RANKL and OPG in gingival crevicular fluid during orthodontic tooth movement and found that the RANKL level was elevated while the OPG level was decreased. They also performed an in vitro study and reported that compression stressed PDL cells increased the secretion of RANKL in a time-dependent manner but decreased the secretion of OPG in a time-dependent manner. Nakajima et al.33 carried out a similar compressive stimulation study on PDL cells. They also reported that RANKL production was elevated in a time and force-dependent manner up to 4 g/cm2, the maximal force that they used. On the other hand, they reported an increase in OPG production in compression-stressed PDL cells. Overall, it appears that compressive stress up-regulates RANKL mRNA and increases the production of RANKL in PDL cells. However, for OPG, there are discrepancies between studies, which will need more investigation to establish the mode of OPG production in PDL cells.
With the exception of Nakajima et al.'s33 study, other studies examining compressive stress effects on PDL cells reported that a 2 g/cm2 force is the optimal level of force that can elicit a maximal PDL cell response.19,23,26 These results also support the optimal force level of 2 g/cm2. At an applied force magnitude of 3 g/cm2, the level of M-CSF, IL-1β and RANKL mRNA expression decreased. This is probably due to the 3 g/cm2 applied force being too heavy making cell survival difficult. Indeed, a microscopic observation after applying 4 g/cm2 showed that the compressive stress was so heavy that PDL cells were partially damaged and?the number of cells decreased (data not shown). Nakao et al.23 suggested that 2 g/cm2 was suitable but 7.0 g/cm2 was too heavy for cell survival. This in vitro result can be applied clinically. Although the applied orthodontic force cannot be distributed uniformly on the desired tooth root surface, the area of root surface that faces a compressive orthodontic force according to the type of tooth movement can be estimated and the desirable amount of force can be calculated by multiplying 2 g/cm2. The validity of this assumption may be proven by another systemized clinical trial investigation.
After 6 hours of 2 g/cm2 compressive mechanical stress, there was a significant increase in the level of M-CSF and RANKL mRNA expression compared to the control. However, the level of IL-1β mRNA expression increased significantly after 24 hours. This means that IL-1β mRNA up-regulation is delayed compared to M-CSF and RANKL. In addition, other cytokines might mediate signal transduction during a compressive mechanical stress. To the best of our knowledge, there are no reports on the effect of compressive stress on the expression of M-CSF and IL-1β mRNA. These results suggest that the expression of M-CSF and IL-1β mRNA increases in a time- and force-dependent manner (up to 2 g/cm2). In addition, it appears that a compressive force directly regulates M-CSF mRNA expression but indirectly regulates IL-1β mRNA expression. More signal transduction studies will be needed to confirm the mechanism of compressive force induced M-CSF and IL-1β mRNA expression.
Continuous compressive stress up-regulates M-CSF, IL-1β and RANKL mRNA expression in cultured hPDL cells in a force- and time-dependent manner. However, a compressive force does not regulate OPG mRNA expression in PDL cells. These results suggest that a compressive stimulated PDL cells regulate osteoclastogenesis by up-regulating the stimulatory cytokines but not down-regulating the OPG production. Therefore, in orthodontic tooth movement, PDL cells participate in bone resorption by up-regulating osteoclastogenetic cytokines, including M-CSF, IL-1β and RANKL.
Figures and Tables
Fig 1
Method used to apply a compressive stress. Pre-cultured PDL cells were compressed continuously using a different number of round-shaped cover glasses. Round-shaped cover glasses were placed over a confluent cell layer in each well of a 6-well plate. The amount of compressive force was adjusted by increasing or decreasing the number of cover glasses placed.
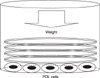
Fig 2
Compressive stress up-regulated M-CSF and IL-1β mRNAs expression in PDL cells. A, RT-PCR analysis of PDL cells. The PDL cells were loaded at different compressive stress (0, 0.5, 1, 2, 3 or 4 g/cm2) for 48 hours; B, Densitometry analysis. The results are expressed as the mean ratio to GAPDH expression of five independent experiments. M-CSF and IL-1β mRNAs expression at 2 g/cm2 was significantly different compared to those of the control.
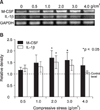
Fig 3
Compressive stress up-regulated M-CSF and IL-1β mRNAs expression in a time dependent manner. A, RT-PCR analysis of PDL cells. The PDL cells were loaded at a constant compressive stress (2 g/cm2) for 0, 0.5, 1.5, 6, 24 or 48 hours; B, Densitometry analysis. The results are expressed as the mean ratio to GAPDH expression of five independent experiments. M-CSF and IL-1β mRNAs expression was significantly different from the control after 6 hours and 24 hours, respectively.
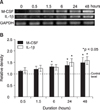
Fig 4
Compressive stress up-regulated RANKL mRNA expression in PDL cells. In contrast, OPG mRNA expression did not change. A, RT-PCR analysis of PDL cells. The PDL cells were loaded at different compressive stresses (0, 0.5, 1, 2, 3 or 4 g/cm2) for 48 hours; B, Densitometry analysis. The results are expressed as the mean ratio to GAPDH expression of five independent experiments. RANKL mRNA expression was significantly different from the control at 2 g/cm2. In contrast, OPG mRNA expression was constant throughout the experiment.
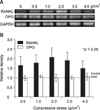
Fig 5
Compressive stress up-regulated RANKL mRNA expression in a time dependent manner. In contrast, OPG mRNA expression did not change. A, RT-PCR analysis of PDL cells. The PDL cells were loaded at a constant compressive stress (2 g/cm2) for 0, 0.5, 1.5, 6, 24 or 48 hours; B, Densitometry analysis. The results are expressed as the mean ratio to GAPDH expression of five independent experiments. RANKL mRNA expression was significantly different from the control after 6 hours. In contrast, OPG mRNA expression was constant throughout the experiment.
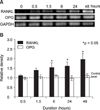
References
1. Roberts WE, Goodwin WC, Heiner SR. Cellular response to orthodontic force. Dent Clin North Am. 1981. 25:3–17.
2. Mitchell DL, West JD. Attempted orthodontic movement in the presence of suspected ankylosis. Am J Orthod. 1975. 68:404–411.


3. Udagawa N, Takahashi N, Jimi E, Matsuzaki K, Tsurukai T, Itoh K, et al. Osteoblasts/stromal cells stimulate osteoclast activation through expression of osteoclast differentiation factor/RANKL but not macrophage colony-stimulating factor. Bone. 1999. 25:517–523.


4. Suda T, Takahashi N, Martin TJ. Modulation of osteoclast differentiation. Endocrine Rev. 1992. 13:66–80.


5. Matsuzaki K, Udagawa N, Takahashi N, Yamaguchi K, Yasuda H, Shima N, et al. Osteoclast differentiation factor (ODF) induces osteoclast-like cell formation in human peripheral blood mononuclear cell cultures. Biochem Biophys Res Commun. 1998. 246:199–204.


6. Jimi E, Nakamura I, Amano H, Taguchi Y, Tsurukai T, Tamura M, et al. Osteoclast function is activated by osteoblastic cells through a mechanism involving cell-to-cell contact. Endocrinology. 1996. 137:2187–2190.


7. Simonet WS, Lacey DL, Dunstan CR, Kelley M, Chang MS, Luthy R, et al. Osteoprotegerin: a novel secreted protein involved in the regulation of bone density. Cell. 1997. 89:309–319.


8. Hasegawa T, Kikuiri T, Takeyama S, Yoshimura Y, Mitome M, Oguchi H, et al. Human periodontal ligament cells derived from deciduous teeth induce osteoclastogenesis in vitro. Tissue Cell. 2002. 34:44–51.


9. Yongchaitrakul T, Lertsirirangson K, Pavasant P. Human periodontal ligament cells secrete macrophage colony-stimulating factor in response to tumor necrosis factor-alpha in vitro. J Periodontol. 2006. 77:955–962.


10. Wada N, Maeda H, Tanabe K, Tsuda E, Yano K, Nakamuta H, et al. Periodontal ligament cells secrete the factor that inhibits osteoclastic differentiation and function: the factor is osteoprotegerin/osteoclastogenesis inhibitory factor. J Periodont Res. 2001. 36:56–63.


11. Lowney JJ, Norton LA, Shafer DM, Rossomando EF. Orthodontic forces increase tumor necrosis factor alpha in the human gingival sulcus. Am J Orthod Dentofacial Orthop. 1995. 108:519–524.


12. Bumann A, Carvalho RS, Schwarzer CL, Yen EH. Collagen synthesis from human PDL cells following orthodontic tooth movement. Eur J Orthod. 1997. 19:29–37.


13. Yamaguchi M, Shimizu N. Identification of factors mediating the decrease of alkaline phosphatase activity caused by tension-force in periodontal ligament cells. Gen Pharmacol. 1994. 25:1229–1235.


14. Lekic P, McCulloch CA. Periodontal ligament cell populations: the central role of fibroblasts in creating a unique tissue. Anat Rec. 1996. 245:327–341.


15. McCulloch CA, Melcher AH. Cell density and cell generation in the periodontal ligament of mice. Am J Anat. 1983. 167:43–58.


16. Kawase T, Sato S, Miake K, Saito S. Alkaline phosphatase of human periodontal ligament fibroblastic cells. Adv Dent Res. 1988. 2:234–239.
17. Kanai K, Nohara H, Hanada K. Initial effects of continuously applied compressive stress to human periodontal ligament fibroblasts. J Jpn Orthod Soc. 1992. 51:153–163.
18. Watanabe K, Saito I, Hanada K. Effects of conditioned medium of continuously compressed human periodontal ligament fibroblasts on MC3T3-E1 cells. J Jpn Orthod Soc. 1998. 57:173–179.
19. Kanzaki H, Chiba M, Shimizu Y, Mitani H. Periodontal ligament cells under mechanical stress induce osteoclastogenesis by receptor activator of nuclear factor κB ligand up-regulation via prostaglandin E2 synthesis. J Bone Miner Res. 2002. 17:210–220.


20. Brown TD. Techniques for mechanical stimulation of cells in vitro: a review. J Biochem. 2000. 33:3–14.


21. Basso N, Heersche JNM. Characteristics of in vitro osteoblastic cell loading models. Bone. 2002. 30:347–351.


22. Nakao K, Goto T, Gunjigake KK, Konoo T, Kobayashi S, Yamaguchi K. Intermittent force induces high RANKL expression in human periodontal ligament cells. J Dent Res. 2007. 86:623–628.


23. Nakao K, Goto T, Gunjigake K, Konoo T, Kobayashi S, Yamaguchi K. Neuropeptides modulate RANKL and OPG expression in human periodontal ligament cells. Orthodontic Waves. 2007. 66:33–40.


24. Choi HS. Effects of tension and compression force on PGE2 of human periodontal ligament cells in vitro. [PhD thesis]. 2000. Seoul: Ewha Womans University.
25. Yamaguchi M, Ozawa Y, Nogimura A, Aihara N, Kojima T, Hirayama Y, et al. Cathepsins B and L increased during response of periodontal ligament cells mechanical stress in vitro. Connect Tissue Res. 2004. 45:181–189.


26. Nishijima Y, Yamaguchi M, Kojima T, Aihara N, Nakajima R, Kasai K. Levels of RANKL and OPG in gingival crevicular fluid during orthodontic tooth movement and effect of compression force on releases from periodontal ligament cells in vitro. Orthod Craniofac Res. 2006. 9:63–70.


27. Suda T, Udagawa N, Nakamura I, Miyaura C, Takahashi N. Modulation of osteoclast differentiation by local factors. Bone. 1995. 17:Suppl. 87–91.


28. Sato K, Fujii Y, Asano S, Ohtsuki T, Kawakami M, Kasono K, et al. Recombinant human interleukin 1 alpha and beta stimulate mouse osteoblast-like cells (MC3T3-E1) to produce macrophage-colony stimulating activity and prostaglandin E2. Biochem Biophys Res Commun. 1986. 141:285–291.


29. Perkins SL, Kling SJ. Local concentrations of macrophage colony-stimulating factor mediate osteoclastic differentiation. Am J Physiol. 1995. 269:E1024–E1030.


30. Yasuda H, Shima N, Nakagawa N, Yamaguchi K, Kinosaki M, Mochizukt S, et al. Osteoclast differentiation factor is a ligand for osteoprotegerin/osteoclastogenesis inhibitory factor and is identical to TRANCE/RANKL. Proc Natl Acad Sci USA. 1998. 95:3597–3602.


31. Tsuji K, Uno K, Zhang GX, Tamura M. Periodontal ligament cells under intermittent tensile stress regulate mRNA expression of osteoprotegerin and tissue inhibitor of matrix metalloprotease-1 and -2. J Bone Miner Metab. 2004. 22:94–103.


32. Lee KJ, Lee SI, Hwang CJ, Ohk SH, Tian YS. The effect of progressive tensional force on mRNA expression of osteoprotegerin and receptor activator of nuclear factor κB ligand in the human periodontal ligament cell. Korean J Orthod. 2005. 35:262–274.