Abstract
Objective:
The purpose of this study is to compare landmark position between cephalometric radiography and midsagittal plane projected images from 3 dimensional (3D) CT.
Methods:
Cephalometric radiographs and CT scans were taken from 20 patients for treatment of mandibular prognathism. After selection of landmarks, CT images were projected to the midsagittal plane and magnified to 110% according to the magnifying power of radiographs. These 2 images were superimposed with frontal and occipital bone. Common coordinate system was established on the base of FH plane. The coordinate value of each landmark was compared by paired t test and mean and standard deviation of difference was calculated.
Results:
The difference was from −0.14 ± 0.65 to −2.12 ± 2.89 mm in X axis, from 0.34 ± 0.78 to −2.36 ± 2.55 mm (6.79 ± 3.04 mm) in Y axis. There was no significant difference only 9 in X axis, and 7 in Y axis out of 20 landmarks. This might be caused by error from the difference of head positioning, by masking the subtle end structures, identification error from the superimposition and error from the different definition.
REFERENCES
1.Broadbent BH. A new x-ray technique and its application to orthodontia. Angle Orthod. 1931. 1:45–66.
2.Baumrind S., Frantz RC. The reliability of head film measurements. 1. Landmark identification. Am J Orthod. 1971. 60:111–27.
3.Ahlqvist J., Eliasson S., Welander U. The effect of projection errors on cephalometric length measurements. Eur J Orthod. 1986. 8:141–8.


4.Midtgard J., Bjork G., Linder-Aronson S. Reproducibility of cephalometric landmarks and errors of measurements of cephalometric cranial distances. Angle Orthod. 1974. 44:56–61.
5.Tng TT., Chan TC., Hägg U., Cooke MS. Validity of cephalometric landmarks. An experimental study on human skulls. Eur J Orthod. 1994. 16:110–20.


6.Houston WJ., Maher RE., McElroy D., Sherriff M. Sources of error in measurements from cephalometric radiographs. Eur J Orthod. 1986. 8:149–51.


7.Grayson BH., McCarthy JG., Bookstein F. Analysis of craniofacial asymmetry by multiplane cephalometry. Am J Orthod. 1983. 84:217–24.


8.Baumrind S., Moffitt FH., Curry S. Three-dimensional x-ray stereometry from paired coplanar images: a progress report. Am J Orthod. 1983. 84:292–312.


9.Grayson B., Cutting C., Bookstein FL., Kim H., McCarthy JG. The three-dimensional cephalogram: theory, technique, and clinical application. Am J Orthod Dentofacial Orthop. 1988. 94:327–37.
10.Kusnoto B., Evans CA., BeGole EA., de Rijk W. Assessment of 3-dimensional computer-generated cephalometric measurement. Am J Orthod Dentofacial Orthop. 1999. 116:390–9.
11.Rousset MM., Simonek F., Dubus JP. A method for correction of radiographic errors in serial three-dimensional cephalometry. Dentomaxillofac Radiol. 2003. 32:50–9.


12.Mozzo P., Procacci C., Tacconi A., Martini PT., Andreis IA. A new volumetric CT machine for dental imaging based on the cone-beam technique: preliminary results. Eur Radiol. 1998. 8:1558–64.


13.Christiansen EL., Thompson JR., Kopp S. Intra- and inter-observer variability and accuracy in the determination of linear and angular measurements in computed tomography. An in vitro and in situ study of human mandibles. Acta Odontol Scand. 1986. 44:221–9.


14.Hildebolt CF., Vannier MW., Knapp RH. Validation study of skull three-dimensional computerized tomography measurements. Am J Phys Anthropol. 1990. 82:283–94.


15.Matteson SR., Bechtold W., Phillips C., Staab EV. A method for three-dimensional image reformation for quantitative cephalometric analysis. J Oral Maxillofac Surg. 1989. 47:1053–61.


16.Kragskov J., Bosch C., Gyldensted C., Sindet-Pedersen S. Comparison of the reliability of craniofacial anatomic landmarks based on cephalometric radiographs and three-dimensional CT scans. Cleft Palate Craniofac J. 1997. 34:111–6.


17.Adams GL., Gansky SA., Miller AJ., Harrell WE Jr., Hatcher DC. Comparison between traditional 2-dimensional cephalometry and a 3-dimensional approach on human dry skulls. Am J Orthod Dentofacial Orthop. 2004. 126:397–409.


18.Park JW., Kim NK., Chang YI. Formulation of a reference coordinate system of three-dimensional (3D) head and neck images: Part I. Reproducibility of 3D cephalometric landmarks. Korean J Orthod. 2005. 35:388–97.
Fig 2.
Acquirement of projected images from CT data. A, Segmentation of skull and skin part using V works; B, projected image to midsagittal plane. By reducing the transparency of the skin part, the projected image looks more like a cephalometric radiograph.
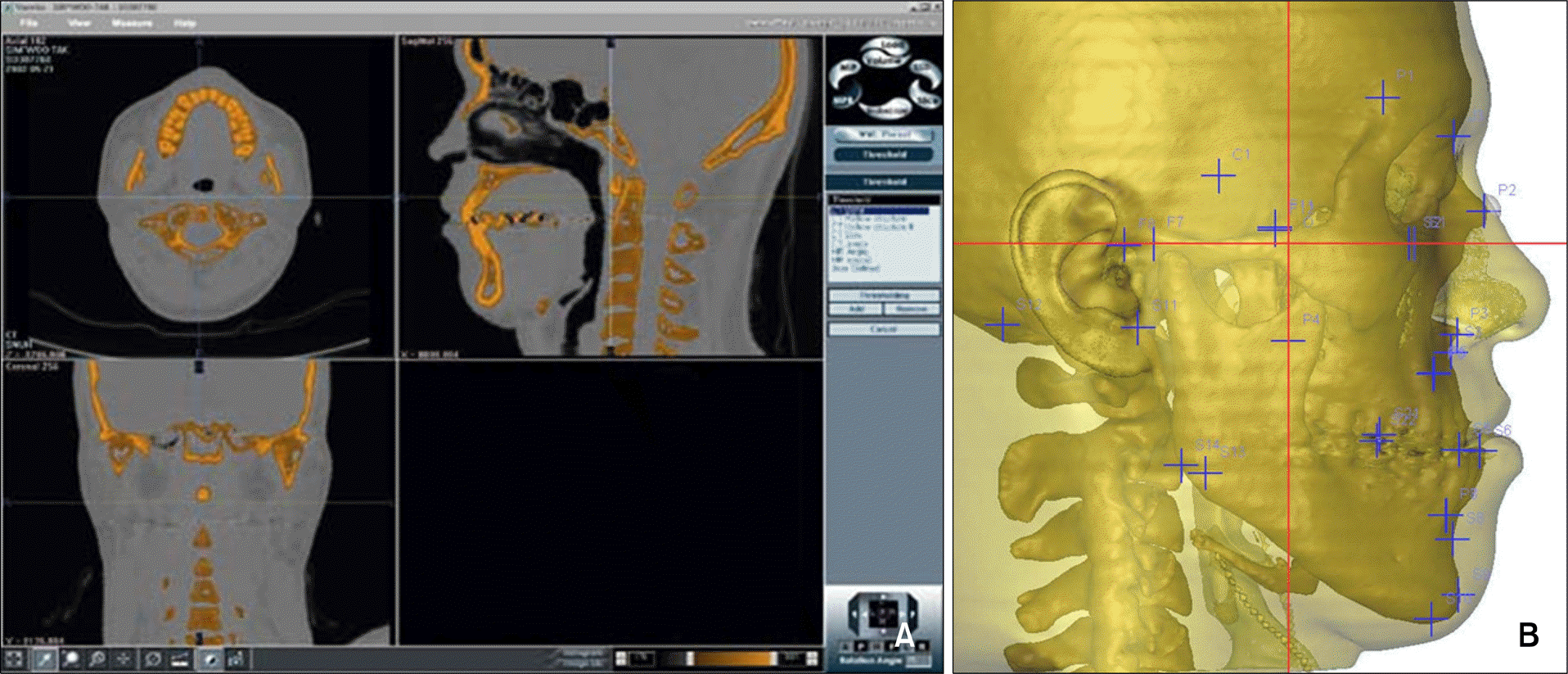
Fig 3.
Superimposition of projected images with cephalometric tracings and establishment of common Cartesian coordinates. A, Two reference points were created parallel to the FH plane in the projected images; B, cephalometric radiograph was traced; C, projected image and tracing were superimposed on to frontal bone and occipital bone, and then the two reference points were copied to the tracing for reprinting of a common coordinate system.
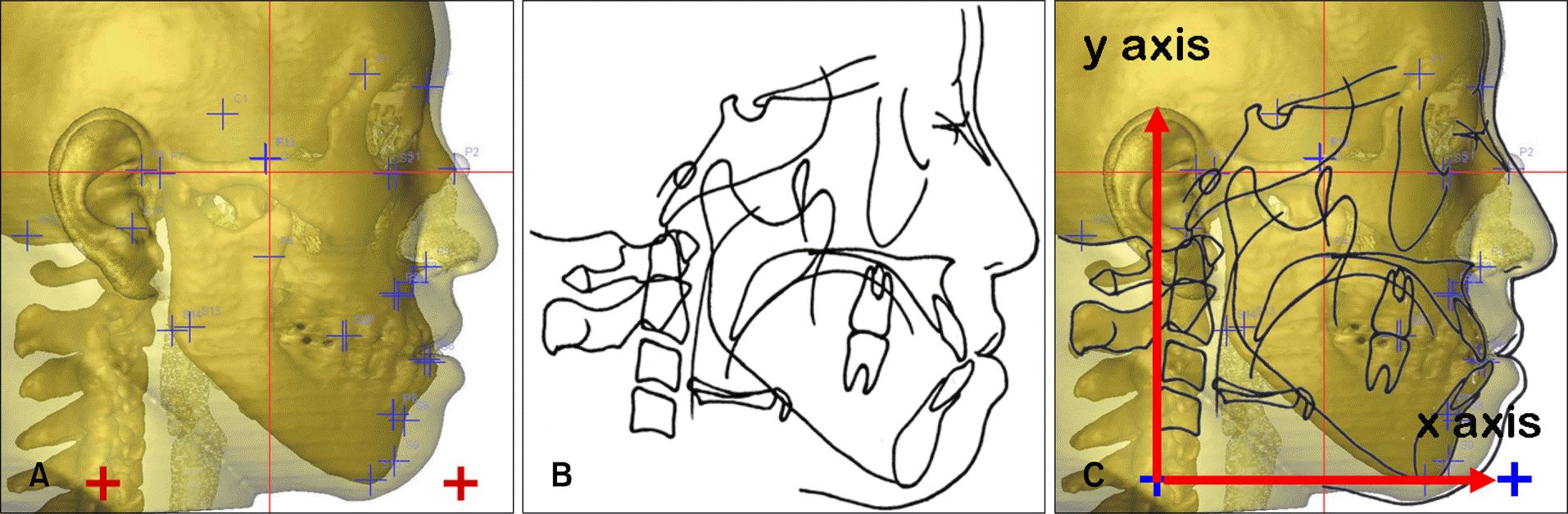
Fig 4.
Difference of landmark position; arrow head is the position in the radiograph, base of arrow is the position in the projected image, and the size of arrow corresponds to the amount of error.
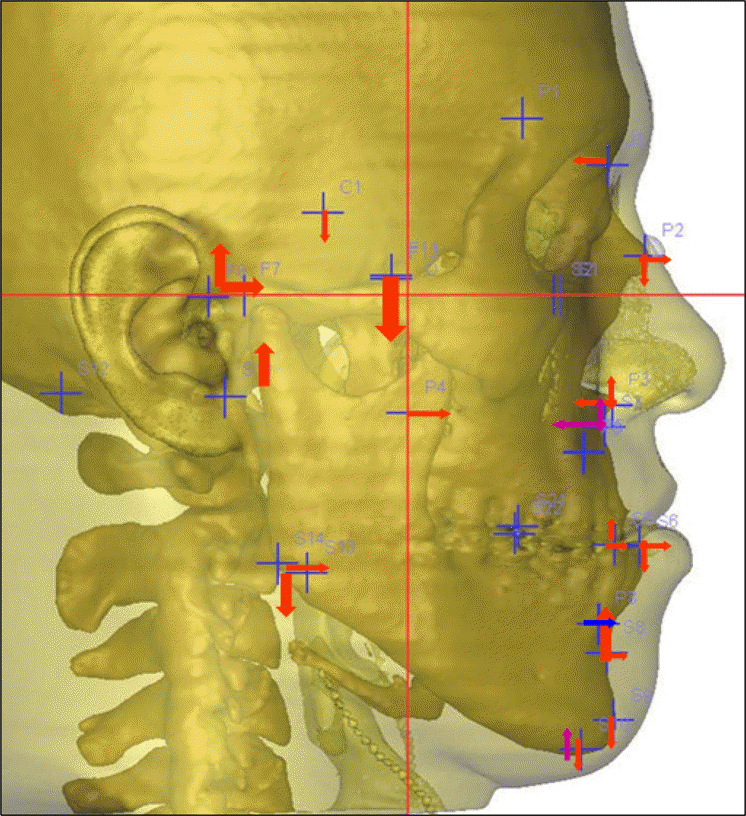
Fig 5.
Errors from differences in body posture. Projected image showed backward downward movement of the mandible.
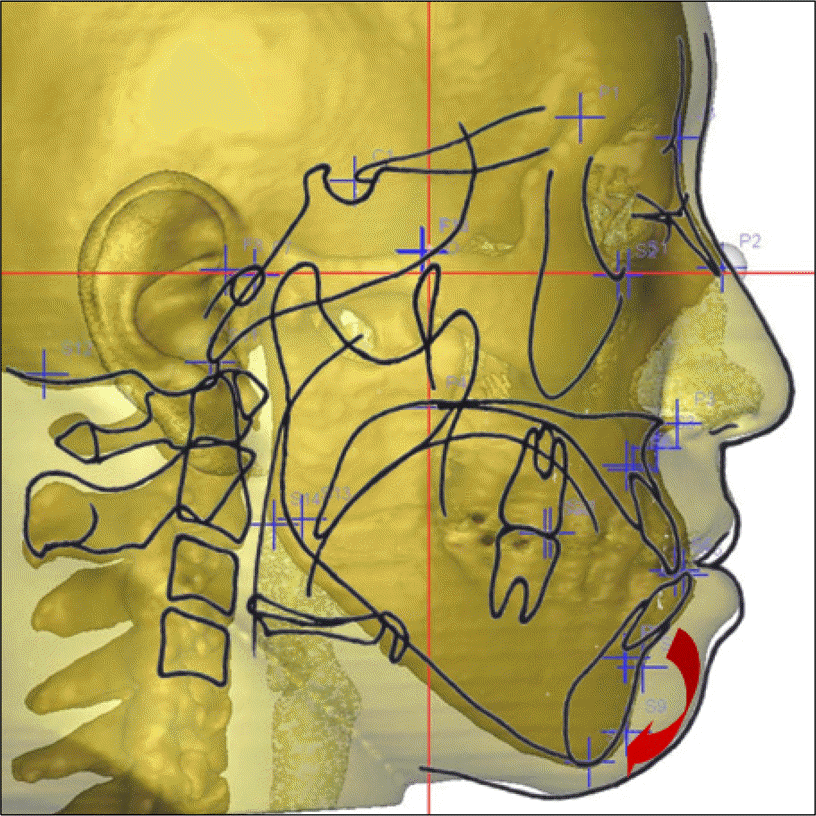
Fig 6.
Errors from the discrepancy of midsagittal plane selected between x-ray taking and projected images. Anterior contour of radiograph was changed with increase in projection angle. A, Projected line was perpendicular to the midsagittal plane; B, projected line was tilted to the midsagittal plane.
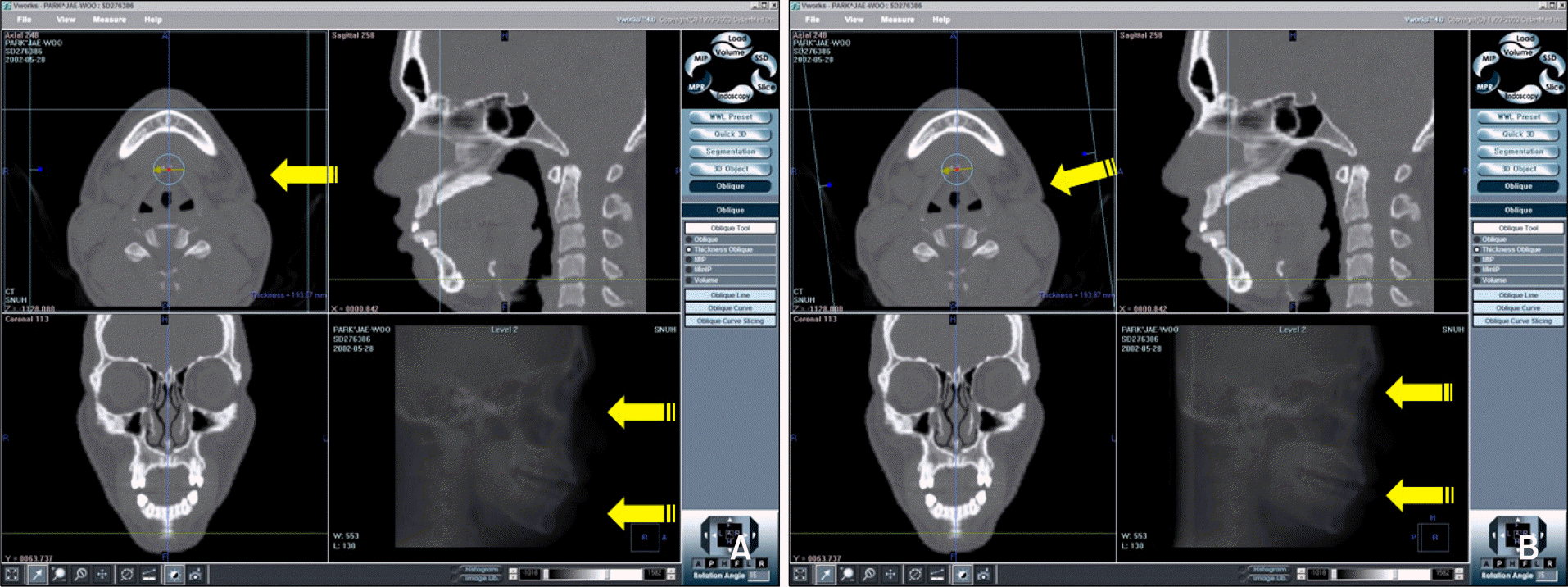
Fig 7.
Errors from the masking of bony projection. A, Ray sum images of the entire head made the position of ANS appear more backward; B, ray sum image around point ANS revealed its original position.
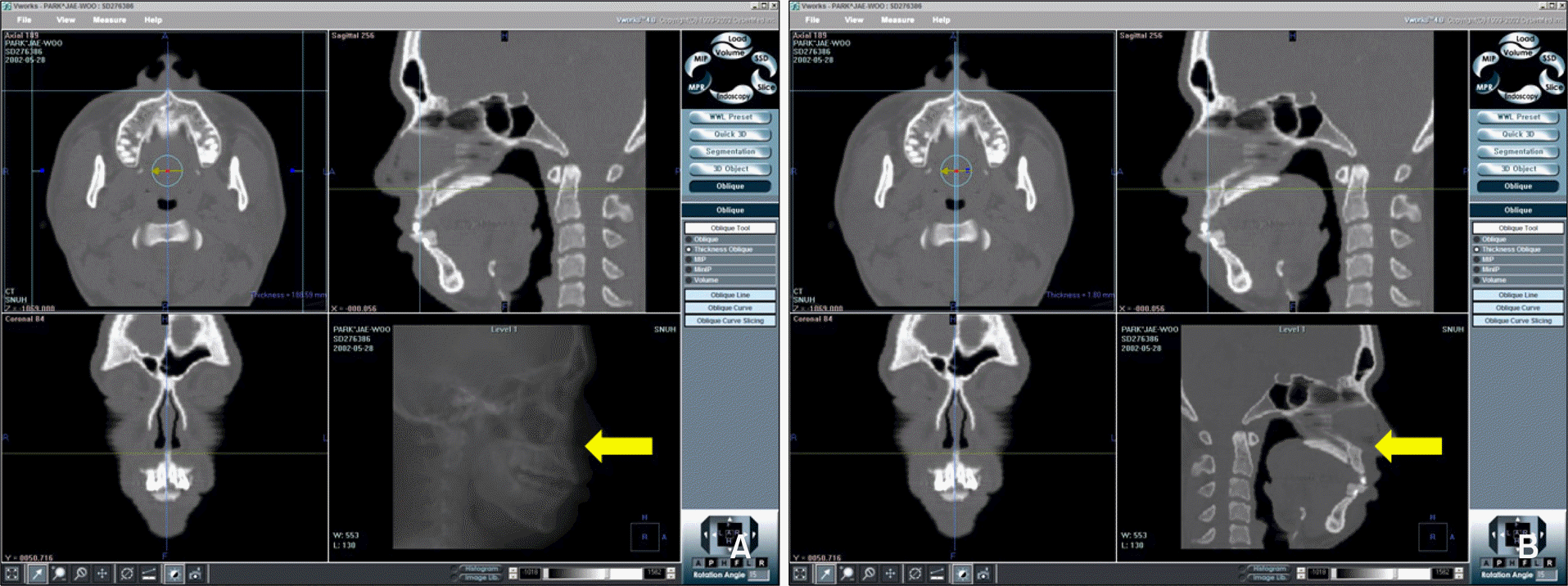
Fig 8.
Errors from the positional differences defined in the radiograph and CT scans. A, Ray sum image of entire head made the position of Ptm appear more downward and backward; B, ray sum image around point Ptm revealed its original position.
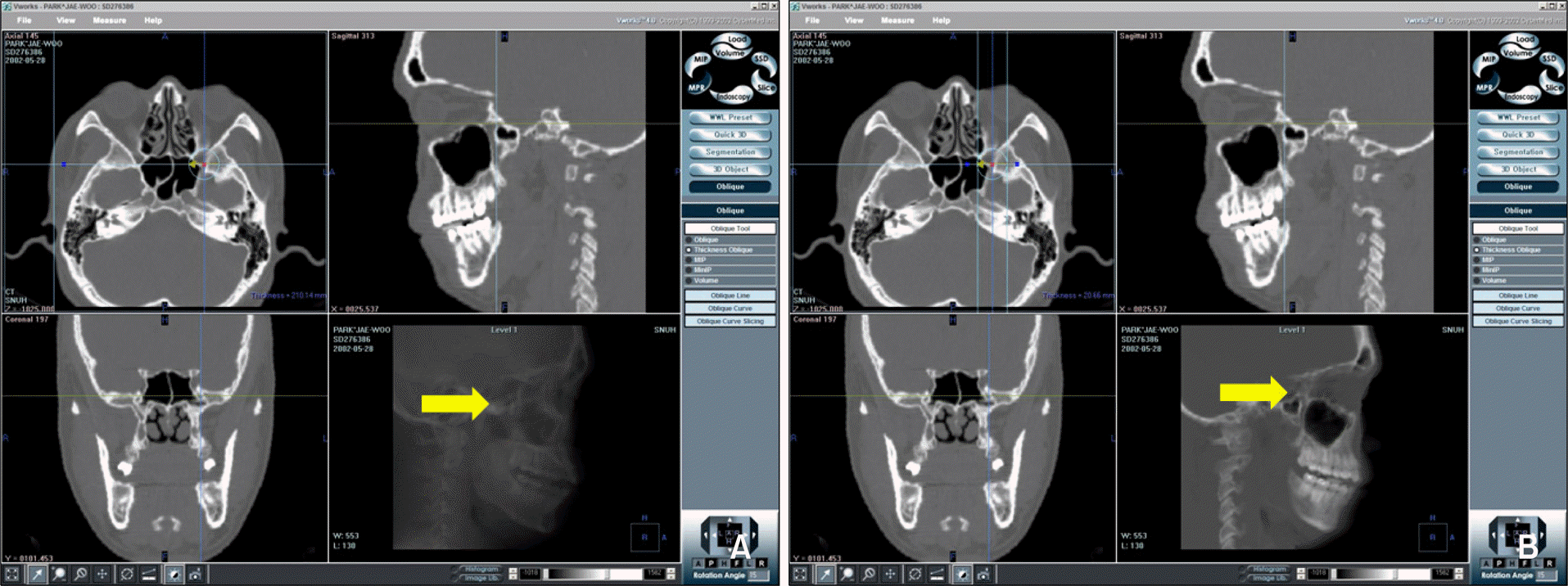
Table 1.
Definition of selected landmark
Table 2.
Intraobserver repeatability for landmark selection in cephalometric radiography and 3D CT (unit: mm)
Table 3.
Mean and standard deviation (SD) of coordinate value difference between cephalometric radiography and 3D CT (unit: mm)
Landmark | X-axis | Y-axis | ||
---|---|---|---|---|
Mean±SD | p-value | Mean±SD | p-value | |
Nasion | 1.01±1.02 | 0.00∗ | 0.47±2.60 | 0.43 |
Orbitale | 0.58±1.50 | 0.10 | 0.34±0.78 | 0.07 |
A point | 2.97±1.56 | 0.00∗ | −1.44±1.41 | 0.00∗ |
Incision superius | −0.60±0.92 | 0.01∗ | −0.71±0.97 | 0.00∗ |
Basion | −0.30±1.86 | 0.47 | −0.06±1.48 | 0.86 |
U6Cr | 0.13±1.23 | 0.65 | −0.25±1.16 | 0.35 |
Porion | −2.12±2.89 | 0.00∗ | −2.36±2.55 | 0.00∗ |
Pterygomaxillary fissure | −0.44±1.71 | 0.26 | 6.79±3.04 | 0.00∗ |
Rhinion | −0.81±1.41 | 0.02∗ | 1.04±1.31 | 0.00∗ |
Anterior nasal spine | 1.58±1.63 | 0.00∗ | −1.07±0.86 | 0.00∗ |
Posterior nasal spine | −1.78±2.32 | 0.00∗ | −0.36±1.26 | 0.21 |
U1apex | −0.46±1.79 | 0.27 | 0.42±2.10 | 0.38 |
Sella | 0.23±0.92 | 0.28 | 1.15±1.04 | 0.00∗ |
Incision inferius | −1.07±1.08 | 0.00∗ | 1.04±0.84 | 0.00∗ |
Bpoint | −0.43±0.62 | 0.01∗ | −2.73±2.00 | 0.00∗ |
Pogonion | −0.14±0.65 | 0.33 | 1.24±1.70 | 0.00∗ |
Menton | 0.28±1.46 | 0.39 | 0.93±0.97 | 0.00∗ |
Menton’ | −0.02±1.38 | 0.96 | −0.48±0.63 | 0.00∗ |
Gonion | −1.45±1.30 | 0.00∗ | 2.41±1.62 | 0.00∗ |
L1apex | −0.87±1.17 | 0.00∗ | −0.84±1.80 | 0.05 |