Abstract
Purpose:
The purpose of this study is to evaluate the efficacy of mesenchymal stem cell (MSC) isolation by the magnetic-activated cell sorting (MACS) method in tendon tissue-derived cells compared to the colony picking method for isolation of MSCs by picking colony-forming cells.
Materials and Methods:
Human tendon-derived cells were isolated by enzyme digestion using normal tendon tissues from three donors. We used the magnetic kit and well-known MSC markers (CD90 or CD105) to isolate MSCs in tendon-derived cells using MACS. Cloning cylinders were used to isolate colony-forming cells having MSC characteristics in tendon-derived cells. Colony-forming unit-fibroblast (CFU-F) assay was used to evaluate the self-renewal capacity of cells isolated using the colony picking method or MACS. For comparison of differentiation potentials into osteogenic or adipogenic lineage between two groups, alizarin red S and oil red O staining were performed at 14 days after induction of differentiation in vitro.
Results:
Flow cytometry results showed that early passage tendon-derived cells expressed CD44 in 99.13%, CD90 in 56.51%, and CD105 in 86.19%. In the CFU-F assay, CD90+ or CD105+ cells isolated with MACS showed larger colony formation in size than cells isolated using the colony picking method. We also observed that CD90+ or CD105+ cells were constantly differentiated into both osteogenic and adipogenic lineages in cells from all donors, whereas cells isolated using the colony picking method were heterogeneous in differentiation potentials to the osteogenic and adipogenic lineages.
REFERENCES
1.Butler DL., Juncosa N., Dressler MR. Functional efficacy of tendon repair processes. Annu Rev Biomed Eng. 2004. 6:303–29.


2.Rees JD., Wilson AM., Wolman RL. Current concepts in the management of tendon disorders. Rheumatology (Oxford). 2006. 41:108–21.


3.Riley G. The pathogenesis of tendinopathy. A molecular perspective. Rheumatology (Oxford). 2004. 43:131–42.


4.Yao L., Bestwick CS., Bestwick LA., Maffulli N., Aspden RM. Phenotypic drift in human tenocyte culture. Tissue Eng. 2006. 12:1843–9.


5.Awad HA., Boivin GP., Dressler MR., Smith FN., Young RG., Butler DL. Repair of patellar tendon injuries using a cell-collagen composite. J Orthop Res. 2003. 21:420–31.


6.Chen X., Song XH., Yin Z., Zou XH., Wang LL., Hu H, et al. Stepwise differentiation of human embryonic stem cells promotes tendon regeneration by secreting fetal tendon matrix and differentiation factors. Stem Cells. 2009. 27:1276–87.


7.Harris MT., Butler DL., Boivin GP., Florer JB., Schantz EJ., Wenstrup RJ. Mesenchymal stem cells used for rabbit tendon repair can form ectopic bone and express alkaline phosphatase activity in constructs. J Orthop Res. 2004. 22:998–1003.


8.Bi Y., Ehirchiou D., Kilts TM., Inkson CA., Embree MC., Sonoyama W, et al. Identification of tendon stem/progenitor cells and the role of the extracellular matrix in their niche. Nat Med. 2007. 13:1219–27.


9.Tan Q., Lui PP., Rui YF., Wong YM. Comparison of potentials of stem cells isolated from tendon and bone marrow for musculoskeletal tissue engineering. Tissue Eng Part A. 2012. 18:840–11.


10.Zhang J., Li B., Wang JH. The role of engineered tendon matrix in the stemness of tendon stem cells in vitro and the promotion of tendon-like tissue formation in vivo. Biomaterials. 2011. 32:6972–81.
11.Lui PP., Wong OT. Tendon stem cells: experimental and clinical perspectives in tendon and tendon-bone junction repair. Muscles Ligaments Tendons J. 2012. 2:163–8.
12.Zhang J., Wang JH. Characterization of differential properties of rabbit tendon stem cells and tenocytes. BMC Musculoskelet Disord. 2010. 11:10.


13.Zhang J., Wang JH. Human tendon stem cells better maintain their stemness in hypoxic culture conditions. PLoS One. 2013. 8:e61424.


14.Bühring HJ., Battula VL., Treml S., Schewe B., Kanz L., Vogel W. Novel markers for the prospective isolation of human MSC. Ann N Y Acad Sci. 2007. 1106:262–71.
15.Gothard D., Dawson JI., Oreffo RO. Assessing the potential of colony morphology for dissecting the CFU-F population from human bone marrow stromal cells. Cell Tissue Res. 2013. 312:237–47.


16.Anzalone R., Lo Iacono M., Corrao S., Magno F., Loria T., Cappello F, et al. New emerging potentials for human Wharton’s jelly mesenchymal stem cells: immunological features and hepatocyte-like differentiative capacity. Stem Cells Dev. 2010. 19:423–38.


17.Liu F., Akiyama Y., Tai S., Maruyama K., Kawaguchi Y., Muramatsu K, et al. Changes in the expression of CD106, osteogenic genes, and transcription factors involved in the osteogenic differentiation of human bone marrow mesenchymal stem cells. J Bone Miner Metab. 2008. 26:312–20.


Figure 1.
Identification of mesenchymal stem cell surface marker CD44, CD90, and CD105 in tendon-derived cells. Flow cytometry analysis of the expression of cell surface markers related to mesenchymal stem cells such as CD44, CD90, and CD105 was performed in tendon-derived cells at passage 2.
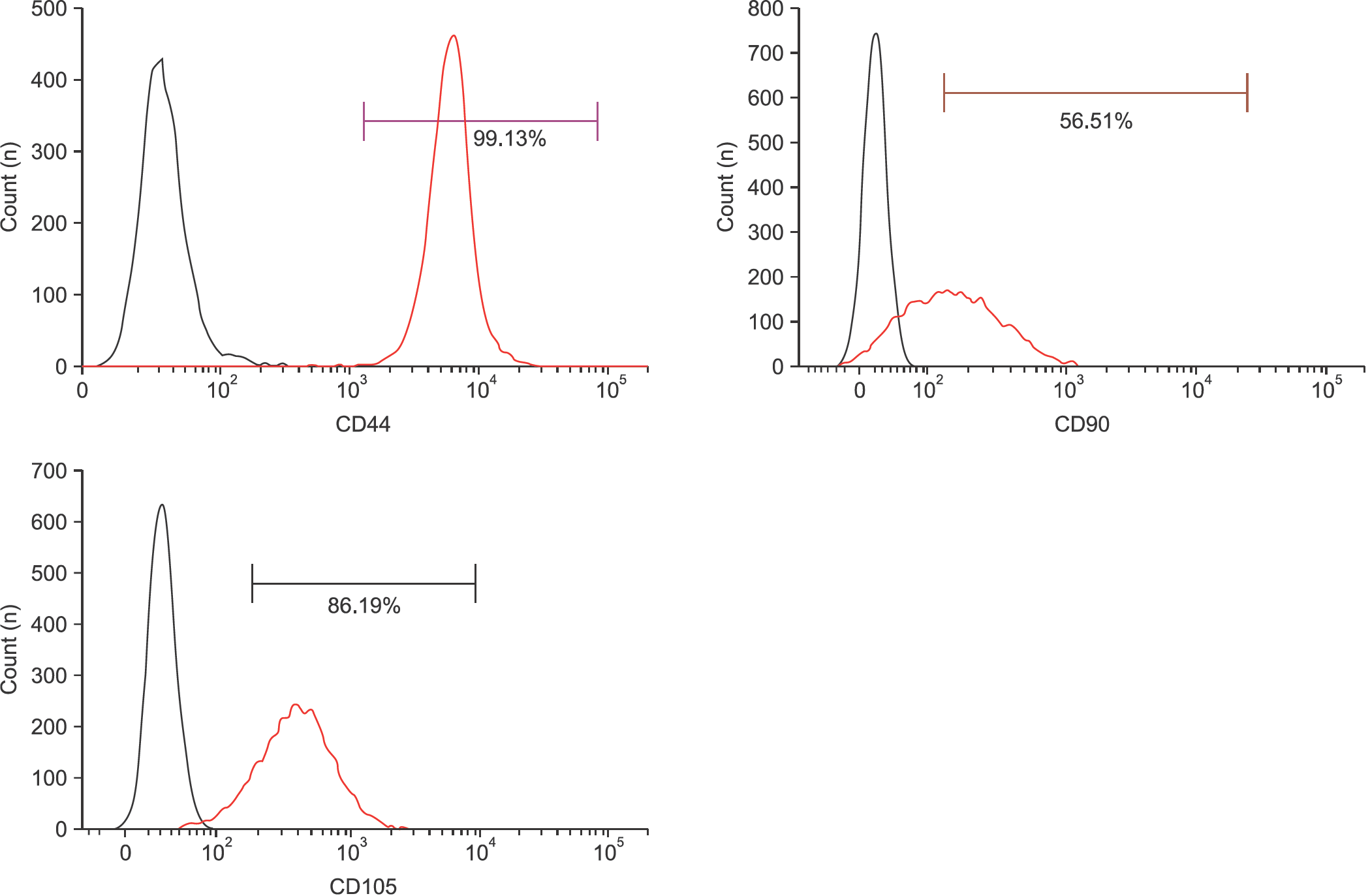
Figure 2.
Isolation methods for tendon-derived mesenchymal stem cells. (A) The cell colonies were dissociated by cloning cylinder and each colony was cultured in growth medium (upper). The isolation method using mesenchymal stem cell surface markers CD90 and CD105 was performed by magnetic kit (bottom). (B) The cells isolated by magnetic-activated cell sorting using CD90 and CD105 were analyzed by flow cytometry.
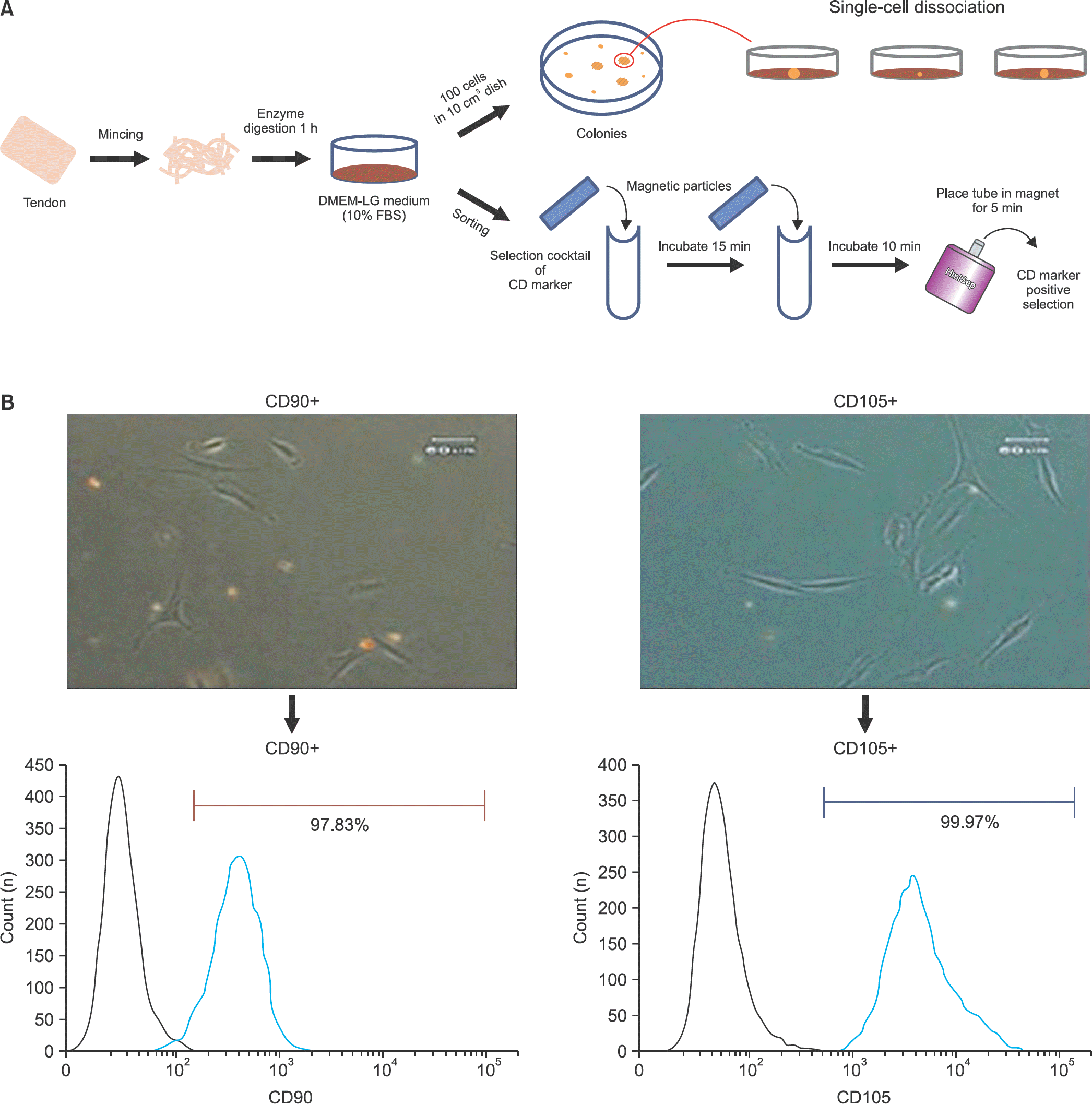
Figure 4.
Quantification of colony-forming units. The number of large colonies was counted and the percentage of large colonies was measured.
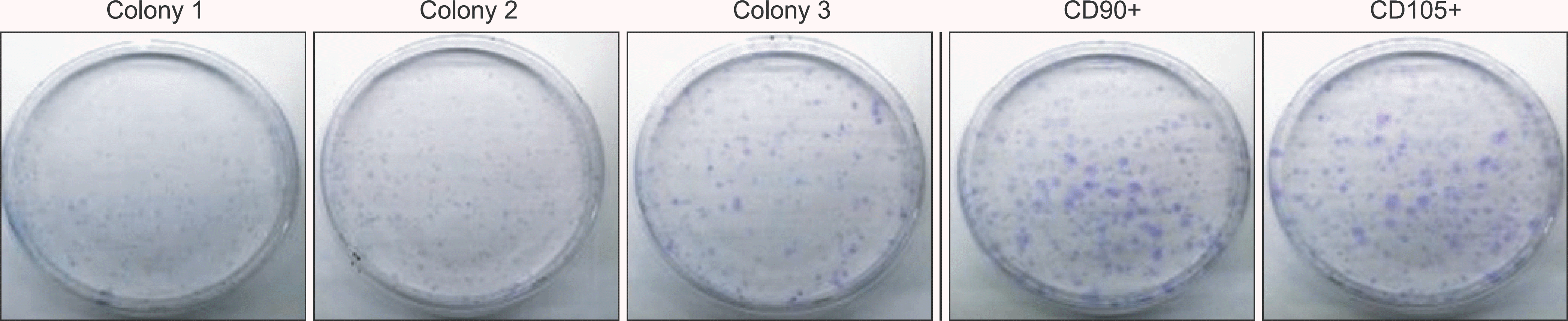
Figure 5.
Osteogenic differentiation capacity. Each cell was seeded on 12-well plates and differentiated for 14 days. (A) The cells isolated by each method were determined by alkaline phosphatase staining at 7 days. Scale bars=200 μm. (B) After induction of osteogenic differentiation for 14 days, alizarin red S staining was performed. (C) Absorbance at 595 nm was measured with a spectrophotometer for quantitative analysis of the stained mineralized nodules.
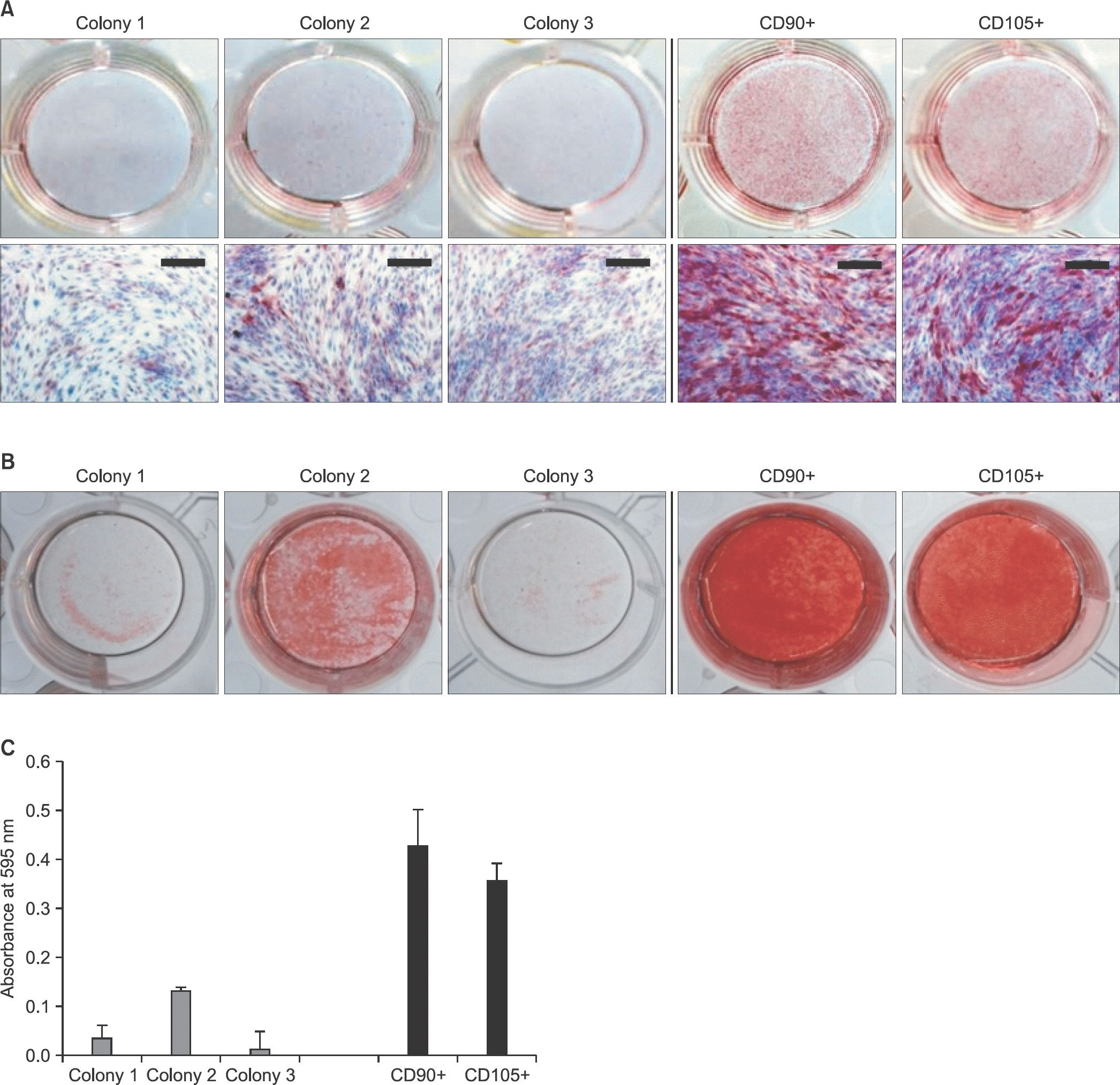