Abstract
The primary merit of a 3 tesla (T) magnetic resonance (MR) scanner is the increase in the signal-to-noise ratio (SNR). It can offer high spatial and temporal image resolution and its diagnostic potential for brain lesions can be improved at the magnetic strength of 3T. In addition to the increased SNR, strong prolongation of T1 relaxation time at high field MR leads to overall improvements in enhancing lesions versus non-enhancing tissue on contrast-enhanced T1-weighted images and blood versus tissue contrast on time-of-flight MR angiography. Increased chemical shift and susceptibility can improve the spectral resolution in MR spectroscopy and the sensitivities in the micro-hemorrhage detection of gradient echo image, the perfusion change of perfusion MRI, and the blood oxygen level-dependent effect of functional magnetic resonance imaging (MRI). The short acquisition time of diffusion MRI at 3T can decrease motion artifacts in irritable stroke patients and it can be easier to estimate anisotrophy and to increase the efficiency of tractography in diffusion tensor imaging with high numbers of gradient directions. On the other hand, the regulation of the specific absorption rate due to increased radio-frequency energy deposition and the controls for signal loss and increased artifacts at 3T are the main clinical problems. If the drawbacks can be addressed by parallel imaging or pulse sequence changes, 3T MRI can be a useful diagnostic tool and increase the diagnostic accuracy in various brain lesions, such as stroke, trauma, epilepsy, multiple sclerosis, dementia, and brain tumors.
Figures and Tables
Figure 1
Brain T2-weighted magnetic resonance imaging (MRI) at 1.5 tesla (T) (A) and 3T (B) were performed in about 3.5 minutes of same scan time and 5 mm of same slice thickness. The spatial resolution of brain MRI at 3T could be increased with high signal-to-noise ratio compared to brain MRI at 1.5T. The matrix size of 3T is 0.4×0.5 mm, whereas the matrix size of 1.5T is 0.6×0.8 mm (courtesy of Philips Healthcare Korea).
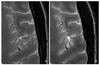
Figure 2
Sixty-six-year-old-male with acute infarction. Diffusion-weighted image (DWI) at 1.5 tesla (T) (A) could not show the acute infarction. However, DWI at 3T (B) shows typical small hyperintense acute infarction at right insula and frontal operculum and apparent diffusion coefficient (ADC) map at 3T (B) shows decreased ADC.
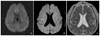
Figure 3
Twenty-four-year-old-male with diffuse axonal injury. Fluid attenuated T2-weighted image at 3 tesla (T) (A) shows small patchy hyperintense edema at the subcortical white matter of right superior frontal gyrus. Susceptibility-weighted image at 3T (B) shows multiple tiny dark microhemorrhage.
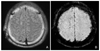
Figure 4
Thirty-nine-year-old-female with left hippocampal sclerosis. Coronal T2-weighted image at 3 tesla (A) shows small and hyperintense left hippocampus. Normal three-layered structure is appeared at normal right hippocampus. Spoiled gradient 3D images show the decreased left hippocampal volume (C) compared with the normal right hippocampal volume (B).
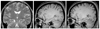
Figure 5
Sixty-six-year-old-female with anaplastic astrocytoma. The left frontal mass is hyperintense on T2-weighted image (A) and well enhanced on contrast-enhanced T1-weighted image (B). The transverse (C) and coronal tractography (D) at 3 tesla show the relationship between the mass and left pyramidal tract. Therefore, they may help to plan the surgical margin.
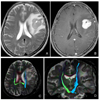
References
1. Willinek WA, Kuhl CK. 3.0 T neuroimaging: technical considerations and clinical applications. Neuroimaging Clin N Am. 2006. 16:217–228.


2. Willinek WA, Schild HH. Clinical advantages of 3.0 T MRI over 1.5 T. Eur J Radiol. 2008. 65:2–14.
5. Pruessmann KP. Parallel imaging at high field strength: synergies and joint potential. Top Magn Reson Imaging. 2004. 15:237–244.
6. Krautmacher C, Willinek WA, Tschampa HJ, Born M, Träber F, Gieseke J, Textor HJ, Schild HH, Kuhl CK. Brain tumors: full- and half-dose contrast-enhanced MR imaging at 3.0 T compared with 1.5 T. Initial Experience. Radiology. 2005. 237:1014–1019.


7. Causes of death statistics, 2009 [Internet]. cited 2010 Sep 9. Daejeon: Statistics Korea;Available from: http://kostat.go.kr.
8. Hoggard N, Wilkinson ID, Griffiths PD. The imaging of ischaemic stroke. Clin Radiol. 2001. 56:171–183.


9. The National Institute of Neurological Disorders and Stroke rt-PA Stroke Study Group. Tissue plasminogen activator for acute ischemic stroke. N Engl J Med. 1995. 333:1581–1587.
10. Hacke W, Kaste M, Fieschi C, Toni D, Lesaffre E, von Kummer R, Boysen G, Bluhmki E, Höxter G, Mahagne MH, Hennerici M. ECASS Study Group. Intravenous thrombolysis with recombinant tissue plasminogen activator for acute hemispheric stroke. The European Cooperative Acute Stroke Study (ECASS). JAMA. 1995. 274:1017–1025.


11. Kuhl CK, Textor J, Gieseke J, von Falkenhausen M, Gernert S, Urbach H, Schild HH. Acute and subacute ischemic stroke at high-field-strength (3.0-T) diffusion-weighted MR imaging: intraindividual comparative study. Radiology. 2005. 234:509–516.


12. Kim HJ, Choi CG, Lee DH, Lee JH, Kim SJ, Suh DC. High-b-value diffusion-weighted MR imaging of hyperacute ischemic stroke at 1.5T. AJNR Am J Neuroradiol. 2005. 26:208–215.
13. Toyoda K, Kitai S, Ida M, Suga S, Aoyagi Y, Fukuda K. Usefulness of high-b-value diffusion-weighted imaging in acute cerebral infarction. Eur Radiol. 2007. 17:1212–1220.


14. Manka C, Träber F, Gieseke J, Schild HH, Kuhl CK. Three-dimensional dynamic susceptibility-weighted perfusion MR imaging at 3.0 T: feasibility and contrast agent dose. Radiology. 2005. 234:869–877.


15. Osborn A, Blaser S, Salzman K, Provenzale J, Castillo M, Sutton D, Hedlund G, Ilner A, Harnsberger H, Cooper J. Diagnostic imaging: brain. 2004. Salt Lake City (UT): Amirsys.
16. Sehgal V, Delproposto Z, Haacke EM, Tong KA, Wycliffe N, Kido DK, Xu Y, Neelavalli J, Haddar D, Reichenbach JR. Clinical applications of neuroimaging with susceptibility-weighted imaging. J Magn Reson Imaging. 2005. 22:439–450.


17. Sawaishi Y, Sasaki M, Yano T, Hirayama A, Akabane J, Takada G. A hippocampal lesion detected by high-field 3 tesla magnetic resonance imaging in a patient with temporal lobe epilepsy. Tohoku J Exp Med. 2005. 205:287–291.


18. Vermathen P, Laxer KD, Schuff N, Matson GB, Weiner MW. Evidence of neuronal injury outside the medial temporal lobe in temporal lobe epilepsy: N-acetylaspartate concentration reductions detected with multisection proton MR spectroscopic imaging-initial experience. Radiology. 2003. 226:195–202.


19. Wattjes MP, Barkhof F. High field MRI in the diagnosis of multiple sclerosis: high field-high yield? Neuroradiology. 2009. 51:279–292.


20. Bachmann R, Reilmann R, Schwindt W, Kugel H, Heindel W, Krämer S. FLAIR imaging for multiple sclerosis: a comparative MR study at 1.5 and 3.0 Tesla. Eur Radiol. 2006. 16:915–921.


21. Kuhl CK, Träber F, Gieseke J, Drahanowsky W, Morakkabati-Spitz N, Willinek W, von Falkenhausen M, Manka C, Schild HH. Whole-body high-field-strength (3.0-T) MR imaging in clinical practice. Part II. Technical considerations and clinical applications. Radiology. 2008. 247:16–35.


22. Whitwell JL, Przybelski SA, Weigand SD, Knopman DS, Boeve BF, Petersen RC, Jack CR Jr. 3D maps from multiple MRI illustrate changing atrophy patterns as subjects progress from mild cognitive impairment to Alzheimer's disease. Brain. 2007. 130(Pt 7):1777–1786.


23. Whitwell JL, Petersen RC, Negash S, Weigand SD, Kantarci K, Ivnik RJ, Knopman DS, Boeve BF, Smith GE, Jack CR Jr. Patterns of atrophy differ among specific subtypes of mild cognitive impairment. Arch Neurol. 2007. 64:1130–1138.


25. Chételat G, Desgranges B, De La Sayette V, Viader F, Eustache F, Baron JC. Mapping gray matter loss with voxel-based morphometry in mild cognitive impairment. Neuroreport. 2002. 13:1939–1943.


26. Chard DT, Griffin CM, McLean MA, Kapeller P, Kapoor R, Thompson AJ, Miller DH. Brain metabolite changes in cortical grey and normal-appearing white matter in clinically early relapsing-remitting multiple sclerosis. Brain. 2002. 125(Pt 10):2342–2352.


27. Dreha-Kulaczewski SF, Helms G, Dechent P, Hofer S, Gärtner J, Frahm J. Serial proton MR spectroscopy and diffusion tensor imaging in infantile Balo's concentric sclerosis. Neuroradiology. 2009. 51:113–121.


28. Kantarci K, Jack CR Jr, Xu YC, Campeau NG, O'Brien PC, Smith GE, Ivnik RJ, Boeve BF, Kokmen E, Tangalos EG, Petersen RC. Regional metabolic patterns in mild cognitive impairment and Alzheimer's disease: A 1H MRS study. Neurology. 2000. 55:210–217.


29. Kantarci K, Weigand SD, Petersen RC, Boeve BF, Knopman DS, Gunter J, Reyes D, Shiung M, O'Brien PC, Smith GE, Ivnik RJ, Tangalos EG, Jack CR Jr. Longitudinal 1H MRS changes in mild cognitive impairment and Alzheimer's disease. Neurobiol Aging. 2007. 28:1330–1339.


30. Modrego PJ, Fayed N, Pina MA. Conversion from mild cognitive impairment to probable Alzheimer's disease predicted by brain magnetic resonance spectroscopy. Am J Psychiatry. 2005. 162:667–675.


31. Scarabino T, Giannatempo GM, Popolizio T, Tosetti M, d'Alesio V, Esposito F, Di Salle F, Di Costanzo A, Bertolino A, Maggialetti A, Salvolini U. 3.0-T functional brain imaging: a 5-year experience. Radiol Med. 2007. 112:97–112.


32. Sperling RA, Bates JF, Chua EF, Cocchiarella AJ, Rentz DM, Rosen BR, Schacter DL, Albert MS. fMRI studies of associative encoding in young and elderly controls and mild Alzheimer's disease. J Neurol Neurosurg Psychiatry. 2003. 74:44–50.


33. Machulda MM, Ward HA, Borowski B, Gunter JL, Cha RH, O'Brien PC, Petersen RC, Boeve BF, Knopman D, Tang-Wai DF, Ivnik RJ, Smith GE, Tangalos EG, Jack CR Jr. Comparison of memory fMRI response among normal, MCI, and Alzheimer's patients. Neurology. 2003. 61:500–506.


34. Johnson SC, Schmitz TW, Moritz CH, Meyerand ME, Rowley HA, Alexander AL, Hansen KW, Gleason CE, Carlsson CM, Ries ML, Asthana S, Chen K, Reiman EM, Alexander GE. Activation of brain regions vulnerable to Alzheimer's disease: the effect of mild cognitive impairment. Neurobiol Aging. 2006. 27:1604–1612.


36. Murakami R, Hirai T, Sugahara T, Fukuoka H, Toya R, Nishimura S, Kitajima M, Okuda T, Nakamura H, Oya N, Kuratsu J, Yamashita Y. Grading astrocytic tumors by using apparent diffusion coefficient parameters: superiority of a one- versus two-parameter pilot method. Radiology. 2009. 251:838–845.


37. Higano S, Yun X, Kumabe T, Watanabe M, Mugikura S, Umetsu A, Sato A, Yamada T, Takahashi S. Malignant astrocytic tumors: clinical importance of apparent diffusion coefficient in prediction of grade and prognosis. Radiology. 2006. 241:839–846.


38. Seo HS, Chang KH, Na DG, Kwon BJ, Lee DH. High b-value diffusion (b = 3000 s/mm2) MR imaging in cerebral gliomas at 3T: visual and quantitative comparisons with b = 1000 s/mm2. AJNR Am J Neuroradiol. 2008. 29:458–463.


39. Law M, Yang S, Babb JS, Knopp EA, Golfinos JG, Zagzag D, Johnson G. Comparison of cerebral blood volume and vascular permeability from dynamic susceptibility contrast-enhanced perfusion MR imaging with glioma grade. AJNR Am J Neuroradiol. 2004. 25:746–755.
40. Calli C, Kitis O, Yunten N, Yurtseven T, Islekel S, Akalin T. Perfusion and diffusion MR imaging in enhancing malignant cerebral tumors. Eur J Radiol. 2006. 58:394–403.


41. Wolf RL, Wang J, Wang S, Melhem ER, O'Rourke DM, Judy KD, Detre JA. Grading of CNS neoplasms using continuous arterial spin labeled perfusion MR imaging at 3 Tesla. J Magn Reson Imaging. 2005. 22:475–482.

