Abstract
The density of intestinal endocrine cells, in Balb/c mice with colon 26 (CT-26) carcinoma cells, were examined immunohistochemically at 28 days after implantation. After CT-26 cell administration there was a significant decrease in most of the intestinal endocrine cells (p < 0.01) compared with the control group. The significant quantitative changes in the intestinal endocrine cell density might contribute to the development of the gastrointestinal symptoms commonly encountered in cancer patients.
There has been extensive research on gastrointestinal (GI) endocrine cells in mouse strains, including normal Balb/c mice [3,10-12]. Changes in GI endocrine cells with some diseases have been demonstrated [1,5,14,15]. Although almost 50% of the most common distressing symptoms reported in cancer patients are GI in nature [9], studies on changes in the GI endocrine cells have been limited to the region of endocrine carcinoid tissues or nonneoplastic mucosa around the carcinoids [15,18]. In addition, there is no experimental data on changes in the GI endocrine cell profile after subcutaneous administration of tumor cells with the exception of 3LL cells. After introducing 3LL cells, the intestinal endocrine cells, particularly those related to the intestinal motility, decreased markedly. [12] In addition, a marked decrease in chromogranin cells, a common marker for endocrine cells [2], was detected after administration of CT-26 cells [7]. However, there are no reports on changes of individual GI endocrine cells in the intestines of Balb/c mice after subcutaneous implantation of CT-26 cells.
The aim of this study was to clarify the changes, in individual endocrine cells, in the intestines of Balb/c mice after the subcutaneous implantation of CT-26 cells by specific immunohistochemistry using antisera against serotonin, somatostatin, glucagons, gastrin, cholecystokinin (CCK)-8, and human pancreatic polypeptide (hPP).
Twenty adult female Balb/c mice (6-weeks old, 21-26 g of body weight upon receipt) were purchased from the Charles River Laboratories (Japan) and used after allowing 1 week for acclimatization. The animals were placed 5 per autoclaved filter-top cage (Nalgene, USA) in a temperature (20-25℃) and humidity (50-55%) controlled room during the acclimatization period. The light:dark cycle was 12 h : 12 h and sterilized feed (Samyang, Korea) and autoclaved water were supplied ad libitum. The animals were divided into two groups containing 10 mice each: a CT-26 cell implanted group and a control sham group. The experimental protocols were carried out in accordance with internationally-accepted principles for laboratory animal use and care, as found in the Korea Food and Drug Administration guidelines.
The CT-26 cells were maintained as a subcutaneous tumor mass. The subcutaneous tumor mass was excised under sterile conditions and single cell suspensions were prepared using collagenase type IV and Dnase I (Sigma-Aldrich, USA) in phosphate-buffered saline (PBS) followed by filtration of the resulting tumor cell suspension through a cell strainer (Costar, USA). After counting and adjusting the number of cells (1 × 105 cells/mouse), the viable CT-26 cells were implanted under the abdominal skin. PBS only was injected subcutaneously at the same site of the sham control group.
Twenty-eight days after implantation (mean lengths of long axis of tumor cell masses in CT-26 cell implanted group were 1.35 ± 0.22 cm), the animals were fasted for approximately 24 h. After phlebotomy, samples from six regions of the intestinal tract, the duodenum, jejunum, ileum, cecum, colon, and rectum were removed and fixed in Bouin's solution.
After paraffin embedding, 3-4 µm serial sections were prepared. Representative sections of each tissue were stained with hematoxylin and eosin for optical microscopy examination of the normal intestinal architecture.
Each representative section was deparaffinized, rehydrated and immunostained using the peroxidase anti-peroxidase (PAP) method [17]. The sections were incubated with normal goat serum for 1 hr at room temperature in a humidity chamber to block nonspecific reactions. After rinsing 3 times in 0.01 M PBS, pH 7.4, the sections were incubated with the specific primary antisera listed in Table 1 for 18 h at 4℃ in a humidity chamber. After rinsing in PBS, the sections were incubated in secondary antiserum (Sigma, USA) for 1 hr at room temperature in a humidity chamber. The sections were then washed in PBS buffer and incubated with the PAP complex (Sigma, USA) for 1 hr at room temperature in a humidity chamber. The peroxidase reaction was carried out in a 3,3'-diaminobenzidine tetrahydrochloride (Sigma, USA) solution containing 0.01% H2O2 in 0.05 M Tris-HCl buffer (pH 7.6). After immunostaining, the sections were lightly counterstained with Mayer's hematoxylin and the immunoreactive (IR) cells were observed by optical microscopy.
The specificity of each immunohistochemical reaction was determined using the method recommended by Sternberger [17], which included the replacement of specific antiserum by the same antiserum that had been preincubated with its corresponding antigen.
IR cells showing immunoreactivity against each antiserum were counted in the restricted view fields on a computer monitor using an automated image analysis process (Soft Image System, Germany) that was coupled to an optical microscope; at least 1,000 epithelial and intestinal acinar cells per slide were evaluated. In each animal, the density of the IR cells was calculated as the mean ± SD of 10 fields per each intestinal region.
In this study, four types of IR endocrine cells were detected in the intestines of Balb/c mice; against serotonin, gastrin, hPP, and CCK-8. Serotonin- and CCK-8-IR cells were identified in the CT-26 implanted- and sham groups, while the hPP- and gastrin-IR cells were observed only in the sham group. On the other hand, the somatostatin- and glucagon-IR cells were not detected in any of the intestinal samples evaluated in this study. According to the location in the intestine, different regional distributions and densities of these IR cells were observed, as shown in Tables 2 and 3. Most of the IR cells in the epithelial regions were generally spherical or spindle-shaped, while round cells were occasionally found in the regions of intestinal glands.
Serotonin-IR cells were observed in the entire intestines of the sham and CT-26 implanted groups. The serotonin-IR cells were dispersed throughout the intestinal mucosa, mainly among the surface epithelium and intestinal glands in both the sham and implanted groups (Fig. 1). They showed the highest density in the duodenum in both groups. The number of serotonin-IR cells in the entire intestines of the CT-26 implanted group was significantly (p < 0.01) lower than in the sham group except for the ileum, where there was similar cell numbers (Tables 2 and 3). Approximately 51.6, 65.1, 6.5, 70.7, 45.0, and 60.0% of the serotonin-IR cells in the duodenum, jejunum, ileum, cecum, colon, and rectum of the CT-26 implanted group compared with the sham, respectively. No somatostatin-IR cells in the intestines of the CT-26 implanted or sham group were observed (Tables 2 and 3). No glucagon-IR cells were detected in the intestines of the CT-26 implanted and non-implanted groups (Tables 2 and 3). A few hPP-IR cells were found in the intestinal glands of the duodenum of the sham (Fig. 2A), but no cells were observed in the CT-26 implanted group (Tables 2 and 3). A few gastrin-IR cells were detected in the intestinal glands of the duodenum of the sham group (Fig. 2B) but no gastrin-IR cells were observed in the CT-26 implanted group (Tables 2 and 3). CCK-8-IR cells were restricted to the duodenum of both groups, and they were dispersed over the surface epithelium or the intestinal glands of the intestinal mucosa regardless of implantation of CT-26 cells (Figs. 3A-C). The number of CCK-8-IR cells in the duodenum of the CT-26 implanted group was similar to the sham group (about 8.3% was decreased) (Tables 2 and 3).
CT-26 cells are rectal carcinoma cells that are established by administrating N-methylmorpholine N-oxide to Balb/c mice. These cells are transplantable to Balb/c mice and are widely used tumor cells for studies of antitumor agents [4]. CT-26 cell implantation resulted in a significant decrease of the number of IR cells (p < 0.01) in the intestinal tract. The most significant changes were noted in the cecum and the greatest changes were detected with serotonin-IR cells. These changes might be responsible for inducing some of the GI abnormalities observed in cancer patients [9]. The changes observed were similar to those previously detected after implantation of 3LL cells [12].
Serotonin inhibits the secretion of gastric acids, and induces smooth muscle contractions in the GI tract [6]. The marked decrease in the number of serotonin-IR cells, in the CT-26 bearing mice, might be responsible for some of the GI problems in cancer patients, particularly those related to gastric motility and gastric acid secretion. The decrease in the number of gastrin-IR cells detected in this study may cause digestive problems, particularly with regard to gastric acid secretion since gastrin secreted by the intestinal G cells promotes gastric acid secretion [16]. CCK secretion by intestinal I cells stimulates pancreatic enzyme secretion [16]. However, there was no change in the number of CCK-8-IR cells observed in this study. Although the precise function of PP is unknown, it is generally accepted that their action is related to exocrine pancreatic functions [11]. Therefore, the absence of these IR cells, detected in this study, may cause abnormalities in exocrine pancreatic digestive functions.
Somatostatin inhibits the secretion of other neuroendocrine hormones [8]. It is well known that somatostatin-IR cells have the widest distribution throughout the GI tract, except for the large intestine, among all vertebrate species examined using serotonin-IR cells [11]. However, somatostatin-IR cells were not detected in this study; this finding may be a strain-specific characteristic of Balb/c mice [10].
In conclusion, endocrine cells are the anatomical units responsible for the production of GI hormones. Any change in the density of these cells may result in the alteration of the production of important GI hormones. The results of this study showed that the implantation of a tumor cell mass (CT-26) induced severe quantitative changes in the intestinal endocrine cell density. This alteration of endocrine cell density might contribute to the development of gastrointestinal symptoms such as anorexia and indigestion, which are frequently encountered in cancer patients.
Figures and Tables
Fig. 1
Serotonin-IR cells in the duodenum (A, D), jejunum (B, E), ileum (C, F), cecum (G, J), colon (H, K) and rectum (I, L) of the non-implanted sham group (A-C, G-I) and CT-26 implanted group (D-F, J-L). In the CT-26 implanted group, serotonin-IR cells were markedly decreased in the intestinal regions except for the ileum. PAP methods. Scale bars = 80 µm.
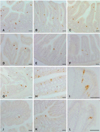
Fig. 2
HPP-(A) and gastrin-(B) IR cells in the duodenum of the non-implanted sham group. These cells were not detected in the CT-26 implanted group. PAP methods. Scale bars = 80 µm.
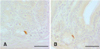
Fig. 3
CCK-8-IR cells of the duodenum of the non-implanted sham group (A, B) and CT-26 implanted group (C). No meaningful changes of CCK-8-IR cells were detected after implantation of CT-26 in the present study. PAP methods. Scale bars = 80 µm.
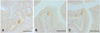
Table 2
Regional distribution and density of the endocrine cells in the small intestine of Balb/c mice with and without implantation of CT-26
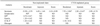
Acknowledgments
This research was supported by research grants from Catholic University of Daegu in 2006.
References
1. Buchan AM, Grant S, Brown JC, Freeman HJ. A quantitative study of enteric endocrine cells in celiac sprue. J Pediatr Gastroenterol Nutr. 1984. 3:665–671.


2. Cohn DV, Elting JJ, Frick M, Elde R. Selective localization of the parathyroid secretory protein-I/adrenal medulla chromogranin A protein family in a wide variety of endocrine cells of the rat. Endocrinology. 1984. 114:1963–1974.


3. D'Este L, Buffa R, Pelagi M, Siccardi AG, Renda T. Immunohistochemical localization of chromogranin A and B in the endocrine cells of the alimentary tract of the green frog, Rana esculenta. Cell Tissue Res. 1994. 277:341–349.
4. DeWys WD. Studies correlating the growth rate of a tumor and its metastases and providing evidence for tumor-related systemic growth-retarding factors. Cancer Res. 1972. 32:374–379.
5. El-Salhy M, Sitohy B. Abnormal gastrointestinal endocrine cells in patients with diabetes type I: relationship to gastric emptying and myoelectrical activity. Scand J Gastroenterol. 2001. 36:1162–1169.
6. Guyton AC. Guyton AC, John EH, editors. Secretory functions of the alimentary tract. Textbook of Medical Physiology. 2006. 11th ed. Philadelphia: Saunders;791–807.
7. Ham TS, Lee HS, Lee JH, Ku SK. Changes of gastrointestinal chromogranin-immunoreactive cells after implantation of Colon-26 murine carcinoma cells in Balb/c mouse. Lab Anim Res. 2005. 21:179–184.
8. Kitamura N, Yamada J, Calingasan NY, Yamashita T. Immunocytochemical distribution of endocrine cells in the gastro-intestinal tract of the horse. Equine Vet J. 1984. 16:103–107.


9. Komurcu S, Nelson KA, Walsh D, Ford RB, Rybicki LA. Gastrointestinal symptoms among inpatients with advanced cancer. Am J Hosp Palliat Care. 2002. 19:351–355.


10. Ku SK, Lee HS, Lee JH. An immunohistochemical study of gastrointestinal endocrine cells in the Balb/c mouse. Anat Histol Embryol. 2004. 33:42–48.


11. Ku SK, Lee HS, Lee JH. An immunohistochemical study of the gastrointestinal endocrine cells in the C57BL/6 mice. Anat Histol Embryol. 2003. 32:21–28.


12. Ku SK, Seong SK, Kim DY, Lee HS, Kim JD, Choi HY, Seo BI, Lee JH. Changes of the intestinal endocrine cells in the C57BL/6 mouse after implantation of murine lung carcinoma (3LL): an immunohistochemical quantitative study. World J Gastroenterol. 2005. 11:5460–5467.


13. Ravazzola M, Baetens D, Engerman R, Kovacevic N, Vranic M, Orci L. Endocrine cells in oxyntic mucosa of a dog 5 years after pancreatectomy. Horm Metab Res. 1977. 9:480–483.


14. Rode J, Dhillon AP, Papadaki L, Stockbrügger R, Thompson RJ, Moss E, Cotton PB. Pernicious anaemia and mucosal endocrine cell proliferation of the non-antral stomach. Gut. 1986. 27:789–798.


15. Shimamoto F, Tahara E, Yanaihara N. Gut endocrine cells in rat intestinal-tract carcinoma induced by 1, 2-dimethylhydrazine. J Cancer Res Clin Oncol. 1983. 105:221–230.


16. Solcia E, Usellini L, Buffa R, Rindi G, Villani L, Aguzzi A, Silini E. Polak JM, editor. Endocrine cells producing regulatory peptides. Regulatory Peptides. 1989. Basel: Birkhauser;220–246.


17. Sternberger LA. Sternberger LA, editor. The unlabeled antibody peroxidase-antiperoxidase (PAP) method. Immunocytochemistry. 1979. New York: John Wiley & Sons;104–169.