Abstract
Galectin-3, a member of the β-galactoside-binding protein family, has been implicated in mammalian sperm maturation. We examined galectin-3 expression in the testis and epididymis of sexually mature and immature bulls. Western blot analysis showed varying levels of galectin-3 in the bull testis and epididymis, and galectin-3 immunoreactivity was higher in the mature testis and epididymis than in immature organs. Galectin-3 was primarily localized in interstitial cells of the immature bull testis and in the peritubular myoid and interstitial cells of the mature testis. In the immature epididymis head, galectin-3 was primarily in the principal and basal cells of the epithelium. In the mature epididymis head, moderate levels of galectin-3 were detected in the sperm, while low levels were found in the stereocilia, epithelium and connective tissue. In the immature epididymis body, moderate protein levels were detected in the principal cells, while lower levels were found in the basal cells. The mature epididymis body showed moderate levels of galectin-3 immunostaining in the stereocilia and epithelium, but low levels in the connective tissue. In the immature epididymis tail, only low levels of galectin-3 staining were found in the epithelium, whereas the mature epididymis tail showed high levels of galectin-3 in the principal cells, moderate levels in the basal cells and low levels in connective tissue. These findings suggest that galectin-3 expression plays a role in the maturation and activation of sperm in bulls.
Galectin-3, also known as Mac-2, eBP, IgE-binding protein, CBP35, CBP30, L-29 and L-34, is a β-galactoside-binding protein that has been highly conserved throughout animal evolution [13,16]. There are approximately 14 members of the galectin family [12], and each contains at least one domain of about 130 amino acids. This carbohydrate recognition domain is responsible for carbohydrate-binding activity. Galectin-3 plays critical roles in cell growth [2], regulation of apoptotic activity [6], mRNA splicing [13], metastasis [18], angiogenesis [14], inflammation and adhesion of leukocytes [1] and regulation of leukocyte viability and cytokine secretion [17].
Male reproductive potential is based on the ability to deliver mature spermatozoa to the female genital tract. The tubular structure of the male reproductive system is well suited for the generation (in the testis), maturation (in the epididymis) and the transport of spermatozoa [15]. The epididymis is a dynamic accessory sex organ that is macroscopically divided into a head, body and tail. It is generally accepted that ducts in the head and body of the epididymis are associated with the maturation of spermatozoa, and that the tail serves as a storage reservoir for spermatozoa. Spermatozoa that leave the epididymis have attained motility and fertility potential.
Galectin-3 is expressed in the urothelium and excretory tubes of the kidney during the first trimester of human embryogenesis [20], the retina [19], and the bull respiratory and digestive tracts during fetal development [10]. With regard to reproductive organs, recent studies show that galectin-3 is differentially expressed in the horse testis [8] and in the boar testis and epididymis [11]. In addition, galectin-3 expression has been reported in pig, rat and human Sertoli cells [4]. However, little is known about the expression or distribution of galectin-3 in the bull testis and epididymis.
The aim of this study was to determine the distribution of galectin-3 expression in the testis and epididymis of sexually immature and mature bulls.
Testis and epididymis samples (n = 4 samples/group) were collected from immature (5-month-old) and mature (24-month-old) bulls (Hanwoo, Korean native cattle) at a local animal farm and slaughterhouse. Both testes and epididymis were fixed in 10% formalin in phosphate buffered saline, gradually dehydrated in ethanol, cleared in xylene and finally embedded in paraffin wax. The paraffin sections were used in all immunostaining experiments. The opposite testis and the 3 parts of the epididymis were snap-frozen and stored at -70℃ for biochemical analysis.
Histological examination confirmed that no pathological features were present, including inflammation, in the testis or epididymis tissue. The 3 distinct regions of the epididymis, the head, body and tail, were examined histologically. The epithelium of the epididymis head was thick, and gradually became thinner in the body and tail. These samples were used for Western blot and immunohistochemical analyses.
A rat anti-galectin-3 monoclonal antibody (1 mg/ml) was purified from the supernatants of hybridoma cells (TIB-166; ATCC, USA). Biotinylated isolectin B4 (IB4) derived from Griffonia simplicifolia (Sigma-Aldrich, USA) was used to label macrophages and mucus-secreting epithelial cells [11], as IB4 has a strong affinity for terminal αD-galactosyl residues that are abundant in macrophages [9] and some epithelial cells [7]. Mouse monoclonal anti-β-actin antibody (Sigma-Aldrich, USA) was used to detect β-actin. β-actin was used as an internal control to ensure that the amounts of protein loaded in Western blot lanes were comparable [11].
Tissue samples of the testis and the 3 regions of the epididymis were homogenized in lysis buffer (40 mM Tris, 120 mM NaCl, 0.1% Nonidet 40, 2 mM Na3VO4, 1 mM PMSF, 10 µg/ml aprotinin, 10 µg/ml leupeptin, pH 7.4). The homogenate was centrifuged at 14,000 rpm for 20 min and the supernatant was collected. For immunoblot assay, the supernatant protein concentration was determined using the Bradford protein assay (Bio-Rad, USA). Samples containing 20 µg/lane were loaded, subjected to sodium dodecyl sulfate polyacrylamide gel electrophoresis and blotted onto nitrocellulose membranes (Schleicher & Schuell, USA) using standard protocols. Lysates of B16F10 mouse melanoma cells were used as positive controls for galectin-3 (data not shown).
Residual binding sites on the membrane were blocked by incubation in 5% nonfat milk in Tris-buffered saline (TBS; 10 mM Tris-HCl, pH 7.4, and 150 mM NaCl) for 1 h. The membrane was then incubated for 2 h with rat anti-galectin-3 (1 : 10,000). The blots were washed 3 times in TBS containing 0.1% Tween 20, and then incubated with horseradish peroxidase-conjugated anti-rat IgG (Santa Cruz Biotechnology, USA) (stock concentration 400 µg/ml; working dilution, 1 : 2,000) for 1 h. Membranes were developed for 1 min in enhanced chemiluminescence reagents (Amersham, USA), prepared according to the manufacturer's instructions, and then exposed to AGFA medical X-ray film (Agfa-Gevaert, Belgium). After imaging, the membranes were stripped and re-probed using monoclonal anti-β-actin antibody (1 : 10,000; Sigma-Aldrich, USA) as the primary antibody for 2 h; otherwise, the protocol described above was followed. The density (OD/mm2) of each band was measured with a scanning laser densitometer (GS-700; Bio-Rad, USA) and was reported as the mean ± SE. The ratios of the density of the galectin-3 band to that of the β-actin band were compared using Molecular Analyst software (Bio-Rad, USA).
Sections (5-µm-thick) of paraffin-embedded bull testis and epididymis were deparaffinized using routine protocols, then immersed in citrate buffer (0.01 M, pH 6.0) and heated in an autoclave for 10 min. All subsequent steps were performed at room temperature. The sections were treated with 0.3% hydrogen peroxide in methyl alcohol for 20 min to block endogenous peroxidase activity. After 3 washes in PBS, the sections were blocked with 10% normal rabbit serum (ABC Elite Kit; Vector Laboratories, USA), immersed in PBS for 1 h, and then allowed to react with the rat anti-galectin-3 antibody (1 : 5,000) for 1 h. After 3 washes in PBS, the sections were reacted for 45 min with biotinylated rabbit anti-rat IgG (1 : 100; Vector Laboratories, USA). After 3 washes in PBS, the sections were incubated for 45 min with an avidin-biotin peroxidase complex (ABC Elite Kit, Vector Laboratories, USA), prepared according to the manufacturer's instructions. After 3 washes in PBS, the peroxidase reaction was developed for 3 min using a diaminobenzidine substrate (DAB Kit; Vector Laboratories, USA), prepared according to the manufacturer's instructions. As a control, the primary antibody was omitted for a few test sections in each experiment. After color development was complete, the sections were counterstained with Harris's hematoxylin for 5 sec, washed in running tap water for 20 min, dehydrated through a graded ethanol series, cleared with xylene, and mounted with Canada balsam (Sigma-Aldrich, USA).
To visualize the co-localization of galectin-3 and IB4 in the bull reproductive tissues, the sections were reacted with biotinylated IB4 (Sigma-Aldrich, USA), followed by TRITC-labeled streptavidin (Zymed Laboratories, USA). The sections were then reacted with the anti-galectin-3 antibody, followed by FITC-labeled goat anti-rat IgG (Zymed Laboratories, USA).
To reduce, or eliminate, lipofuscin autofluorescence, the sections were washed 3 times for 5 min each in PBS at room temperature, treated with 10 mM CuSO4 in 50 mM CH3COONH4 buffer (pH 5.0) for 20 min, and returned to PBS. The double-immunofluorescence-stained specimens were examined under a FV500 laser confocal microscope (Olympus, Japan).
Western blot analysis with the anti-galectin-3 antibody allowed for detection of changes in galectin-3 expression as a result of sexual maturation of the male reproductive organs. Galectin-3 was detected in both the mature and immature testis and all 3 parts of the epididymis (Fig. 1).
We found that the intensity of galectin-3 (molecular weight ~29 kDa) staining increased more than two-fold in the mature (Fig. 1, lane 2) compared to the immature testis (Fig. 1, lane 1). Galectin-3 was detected at high levels in all 3 regions of the epididymis. Similar to the results for the testis, galectin-3 levels in the epididymis head, body and tail were higher in mature (Fig. 1, lanes 4, 6 and 8) compared to immature bulls (Fig. 1, lanes 3, 5 and 7).
Galectin-3 did not immunostain the seminiferous tubules of both immature (Fig. 2A) and mature bull testes (Fig. 2B). However, galectin-3 showed intense immunostaining in some interstitial cells, possibly Leydig cells and macrophages, in the immature (Fig. 2A, arrow) and mature testes (Fig. 2B, arrows).
In the immature epididymis head (Figs. 2D-F), galectin-3 was detected in the principal (Fig. 2D, arrows) and basal cells (Fig. 2D, arrowheads), but not in the stereocilia or connective tissues. In the immature epididymis body (Fig. 2E), moderate levels of galectin-3 were detected in the principal cells (Fig. 2E, arrows), while staining in the basal cells was sparse, and no galectin-3 was found in the connective tissue. Only weak staining for galactin-3 was observed in the epithelium of the immature epididymis tail (Fig. 2F, arrows).
In the mature epididymis head (Figs. 2G-I), moderate levels of galectin-3 were detected in the sperm (Fig. 2G, asterisks), while protein expression was low in the stereocilia (Fig. 2G, arrows), epithelium and connective tissue. The stereocilia and principal cells (Fig. 2H, arrows) of the mature body of the epididymis had moderate levels of galectin-3, but expression of the protein was low in the connective tissue, and no galectin-3 staining was observed in the basal cells and sperm (Fig. 2H). Intense immunoreactivity of galectin-3 was detected in the epithelium of the mature epididymis tail (Fig. 2I, arrows). Galectin-3 was found at moderate levels in the basal cells, but only low levels were detected in the connective tissue, and no galectin-3 was found in the stereocilia or sperm (Fig. 2I). The immunohistochemical findings are summarized in Table 1. These agreed with the localizations of galectin-3 expression shown by the Western blot analysis.
In the head (Figs. 3A-C), body (Figs. 3D-F) and tail (Figs. 3G-I) of the mature bull epididymis, IB4 was detected in some galectin-3-positive macrophages in the submucosa (Figs. 3A-F, arrows), as well as in some galectin-3-positive epithelial cells (Figs. 3A-I).
This is the first study to show that galectin-3 is differentially expressed in the testis and epididymis of immature and mature bulls.
Galectin-3 has previously been detected in pig, rat and human Sertoli cells [4], as well as in human and boar interstitial cells [5,11]. The variations of galectin-3 expressions in the immature and mature testis, depending on the cell maturation and differentiation status, might be associated with the hormone dependence of galectin genes; galectin-3 is specifically expressed in mature Leydig cells, but is absent from immature testes in humans [5]. In the present study, the levels of galectin-3 expression in the interstitial and peritubular myoid cells of the mature bull testes were higher than in the immature testes, suggesting that galectin-3 is associated with hormone-dependent cell activity.
In this study, we did not detect galectin-3 expression immunohistochemically in mature Sertoli cells, although galectin-3 is specifically expressed in Sertoli, as well as the Leydig cells of mature human, rat and porcine testes [4,5]. We can only speculate that this discrepancy may have resulted from the monoclonal anti-galectin-3 antibody used in this study, which may uniquely recognize galectin-3 in bovine testis. Also, we cannot exclude the possibility of species variation, which may cause differential galectin-3 immunoreactivities in the testes of mammals and/or the Sertoli cells that contain galectin-3 if these cells are stimulated by certain hormones [4].
In the 3 regions of the epididymis, galectin-3 was primarily detected in the sperm, stereocilia, and principal and basal cells. In addition, galectin-3 levels were higher in mature reproductive tissue compared to immature tissue. Recent studies indicate that galectin-3 is expressed in a variety of epithelial cells, including the mucosa of the mouse urinary system and the bull respiratory system [19]. Our study confirmed that galectin-3 is occasionally present in mucus-secreting (IB4-positive) epithelial cells in the epididymis (especially intensive expression in the epididymis tail). Galectin-3 plays an important role in the mucosal epithelium, including the maturation of sperm in the lumen in conjunction with epididymal secretory proteins in the boar [3,11]. We postulate that the sperm cells in the epididymis are influenced by galectin-3 secreted from the mucosal epithelium, and that this protein may play a role in the activation and maturation of sperm. Additional studies remain to be done to establish the role of galectin-3 during the course of sperm maturation in the epididymis.
In conclusion, we demonstrated that galectin-3 is present in the immature and mature bull reproductive organs, and that it may play a role in the maturation and activation of sperm in bulls.
Figures and Tables
Fig. 1
Western blot analysis of galectin-3 in bull reproductive tissue. Differential expressions of galectin-3 in the immature and mature testis and epididymis. Lower panel shows the expression of the internal control, β-actin, in the same membrane. T, testis; M, mature; IM, immature; E, epididymis.
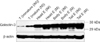
Fig. 2
Immunohistochemical staining of the immature (A, D-F) and mature (B, G-I) testis (A, B) and epididymis (D-I). C shows a negative control section in which the primary antibody was omitted. Sections were counterstained with hematoxylin. Scale bars = 50 µm.
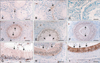
Fig. 3
Immunofluorescent co-localization of galectin-3 with isolectin B4. (A-C) The mature head, (D-F) body and (G-I) tail of the epididymis. (A-F) Arrows show galectin-3 immunoreactivity in isolectin B4-positive macrophages. (G-I) Arrowheads indicate galectin-3 immunoreactivity in isolectin B4-positive mucosal epithelium. C, F, and I are merged images. Scale bars = 20 µm.
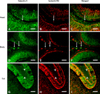
References
2. Barondes SH, Cooper DN, Gitt MA, Leffler H. Galectins. Structure and function of a large family of animal lectins. J Biol Chem. 1994. 269:20807–20810.


3. Dacheux JL, Castella S, Gatti JL, Dacheux F. Epididymal cell secretory activities and the role of proteins in boar sperm maturation. Theriogenology. 2005. 63:319–341.


4. Deschildre C, Ji JW, Chater S, Dacheux F, Selva J, Albert M, Bailly M, Hatey F, Benahmed M. Expression of galectin-3 and its regulation in the testes. Int J Androl. 2007. 30:28–40.


5. Devouassoux-Shisheboran M, Deschildre C, Mauduit C, Berger G, Mejean-Lebreton F, Bouvier R, Droz JP, Fénichel P, Benahmed M. Expression of galectin-3 in gonads and gonadal sex cord stromal and germ cell tumors. Oncol Rep. 2006. 16:335–340.


6. Dumic J, Dabelic S, Flögel M. Galectin-3: an open-ended story. Biochim Biophys Acta. 2006. 1760:616–635.


7. Flint FF, Schulte BA, Spicer SS. Glycoconjugate with terminal alpha galactose. A property common to basal cells and a subpopulation of columnar cells of numerous epithelia in mouse and rat. Histochemistry. 1986. 84:387–395.
8. Ha TY, Ahn MJ, Lee YD, Yang JH, Kim HS, Shin TK. Histochemical detection of glycoconjugates in the male reproductive system of the horse. J Vet Sci. 2003. 4:21–28.


9. Judd WJ, Murphy LA, Goldstein IJ, Campbell L, Nichols ME. An anti-B reagent prepared from the alpha-D-galactopyranosyl-binding isolectins from Bandeiraea simplicifolia seeds. Transfusion. 1978. 18:274–280.


10. Kaltner H, Seyrek K, Heck A, Sinowatz F, Gabius H. Galectin-1 and galectin-3 in fetal development of bovine respiratory and digestive tracts. Comparison of cell type-specific expression profiles and subcellular localization. Cell Tissue Res. 2002. 307:35–46.


11. Kim H, Kang TY, Joo HG, Shin T. Immunohistochemical localization of galectin-3 in boar testis and epididymis. Acta Histochem. 2006. 108:481–485.


12. Leffler H, Masiarz FR, Barondes SH. Soluble lactose-binding vertebrate lectins: a growing family. Biochemistry. 1989. 28:9222–9229.


13. Liu FT, Patterson RJ, Wang JL. Intracellular functions of galectins. Biochim Biophys Acta. 2002. 1572:263–273.


14. Nangia-Makker P, Sarvis R, Visscher DW, Bailey-Penrod J, Raz A, Sarkar FH. Galectin-3 and L1 retrotransposons in human breast carcinomas. Breast Cancer Res Treat. 1998. 49:171–183.


15. Ong CN, Shen HM, Chia SE. Biomarkers for male reproductive health hazards: are they available? Toxicol Lett. 2002. 134:17–30.


16. Rabinovich GA, Baum LG, Tinari N, Paganelli R, Natoli C, Liu FT, Iacobelli S. Galectins and their ligands: amplifiers, silencers or tuners of the inflammatory response? Trends Immunol. 2002. 23:313–320.


17. Stowell SR, Qian Y, Karmakar S, Koyama NS, Dias-Baruffi M, Leffler H, McEver RP, Cummings RD. Differential roles of galectin-1 and galectin-3 in regulating leukocyte viability and cytokine secretion. J Immunol. 2008. 180:3091–3102.


19. Uehara F, Ohba N, Ozawa M. Isolation and characterization of galectins in the mammalian retina. Invest Ophthalmol Vis Sci. 2001. 42:2164–2172.