Abstract
Hip dysplasia (HD) is one of the most important bone and joint diseases in dogs. Making the radiographic diagnosis is sometime possible when the disease has markedly progressed. Chondroitin sulfate (CS) and hyaluronan (HA) are the most important cartilage biomolecules that are elevated in the serum taken from dogs with osteoarthritis. The serum CS and HA can be detected by an ELISA technique, with using monoclonal antibodies against CS epitope 3B3 and WF6 and the HA chain as the primary antibodies. The aim of this study was to compare the levels of serum CS (both epitopes) and HA in non-HD and HD dogs. All 123 dogs were categorized into 2 groups. The non-HD group was composed of 98 healthy dogs, while the HD group was comprised of 25 HD dogs. Blood samples were collected for analyzing the serum CS and HA levels with using the ELISA technique. The results showed that the average serum level of the CS epitope WF6 in the HD group (2,594 ± 3,036.10 ng/ml) was significantly higher than that in the non-HD group (465 ± 208.97 ng/ml) (p < 0.01) while the epitope 3B3 in the HD group (105 ± 100.05 ng/ml) was significantly lower than that in the non-HD group (136 ± 142.03 ng/ml) (p < 0.05). The amount of serum HA in the HD group (134.74 ± 59.71 ng/ml) was lower than that in the non HD group (245.45 ± 97.84 ng/ml) (p < 0.05). The results indicate that the serum CS and HA levels might be used as biomarkers for osteoarthritis in HD dogs.
Many studies have been done to understand the mechanism of cartilage degradation in joint disease and to assess changes in the cartilage metabolism in vivo. In vitro studies have enormously increased our understanding of how cytokines or growth factors influence cartilage metabolism, but it is obviously important to develop the means of studying and understanding cartilage metabolism in vivo to determine how the cartilage metabolism changes in a disease state. Moreover, an in vivo approach may also help us determine whether therapeutic interventions have beneficial or negative effects on cartilage metabolism.
Articular cartilage is a metabolically-active structure that is specifically designed to accommodate the tensile and compressive forces generated within the joint. This cartilage is composed of cells named chondrocytes, and these cells produce the extracellular matrix (ECM). The biochemical properties of cartilage and the physical function of joints are critically dependent on the integrity of the matrix. The ECM molecules in cartilage include proteoglycan (PG), hyaluronan (HA), glycoprotein and type II collagen. Proteoglycans are a family of glycoconjugates with a central core protein to which one or more glycosaminoglycan (GAG) side chains are covalently linked post-translationally [62]. In addition, most of the PGs exist as aggregates that are formed by the non-covalent association of proteoglycan with HA and linked protein [22]. Among the PGs in cartilage, the most crucial for the proper functioning of articular cartilage is aggrecan, which is one of the large aggregating chondroitin sulfates (CSs) [28]. CS consists of an alternating sequence of D-glucoronate and N-acetyl-D-galactosamine-4/6-sulfate residues that are linked through alternating bonds [56]. Although the CSs are often referred to as if they were a homogenous substance, their polysaccharide chains are comprised of several unique, but structurally similar disaccharides; the most abundant are CSs, which are typically chondroitin-4-sulfate and chondroitin-6-sulfate. The CS is a heterogenous group of compounds that have different molecular masses (15,000-25,000 kDa) and electric change densities [27], and CSs are an essential component of the connective tissue ECM, including the hyaline cartilage, and the CSs provide elasticity and other functions.
The HA is a ubiquitous component of the ECM of most animal tissues. A high molecular weight (300-2,000 kDa) member of the polysaccharides group is termed GAG [39]. HA is a linear macromolecule that is composed of a repeating disaccharide units: β-1,4-glucuronic acid-β-1,3-N-acetyl-D-glucosamine [16]. HA is mainly produced by fibroblasts and other specialized connective tissue cells. Although HA is widely distributed throughout the body (umbilical cord, nasal cartilage, vitreum, cutis or lymph of the thorax), the highest concentration is found in synovial fluid and also connective tissue such as the synovial membrane [31]. Its production has been linked to a variety of diseases [30].
Hip dysplasia (HD) is the abnormal development of the coxofemoral joint [38]. The disorder has been reported in humans and most domestic animals. The first report of HD in dogs was published in 1935. This disorder has become one of the most commonly diagnosed orthopedic diseases in dogs [25]. A study in 2003 showed that the prevalence of HD was 19.3% in the general population of pet dogs. The percentage of dysplasia for these breeds in that study was 35.4% for Rottweilers, 32.9% for German Shepherds, 30.3% for Golden Retrievers and 27% for Labrador Retrievers [53]. Moreover, the majority of the HD dogs (80%) had osteoarthritis (OA) [59]. So far, the initiating factors are unknown, and the rate and extent of the development of HD disease are variable, but the risk factors are both genetic [33] and environmental [11]. As mentioned above, most of the dogs with HD in the above mentioned study also had OA, but the standardized diagnostic protocol consists of the clinical sign, a physical examination and evaluation of the radiographic results, which can not detect OA in its early stages [42,45]. Moreover, many factors influence the radiographic diagnosis, such as the dog's position during X-ray, the beam direction, the film quality, the development process or the severity of the disease [45] and most cases require contrast medium for making the final diagnosis [1].
The OA is defined as a non-inflammatory, degenerative joint disease that's characterized by the loss of articular cartilage, subchondral osteosclerosis and marginal hypertrophy of bone; this is accompanied with pain and soft tissues stiffness that's aggravated by prolonged activity [41]. The collagen framework becomes disrupted, and the PG content of the articular cartilage diminishes, particularly near the articular surface. PG fragments that contain CS and keratin sulfate (KS), as well as the breakdown products of type II collagen, are liberated in increased concentrations to reach the synovial fluid and ultimately the serum [5,21,34].
Biomarkers have been widely used to monitor disease activity, to predict disease progression and to study the effects of novel therapeutic interventions in a variety of joint diseases [9,10,36,37,40,48,61]. Biomarkers have also been used in a variety of species such as dogs [10,21,51,52], horses [17,44,47], mice [19] and rabbits [29]. Most of the biomarkers used in joint disease are the articular cartilage components such as CS [5,10,13], KS [5], HA [6,10,19,34] or collagen type II [23]. It is well recognized that the early stages of cartilage degradation and osteoarthritis are difficult or impossible to define diagnostically. In normal cartilage metabolism, the balance between catabolism and anabolism is necessary for maintenance of the cartilage's function [12]. This metabolism changes when the joint environment is interrupted by factors such as chemical or physical effects. The biomolecules are upregulated via synthesis from the chondrocytes to restore the balance between catabolism and anabolism in the initial phase. For this process, many biomarkers such as matrix metalloprotease-3 or tissue inhibitor of metalloprotease-1 [21] and CS epitope 3B3 [43] were found to be at higher concentrations. In this disease state, the articular cartilage is highly degraded, and the joint develops OA if the balance is not recovered in the last phase [48,54]. Some biomolecules were elevated in this phase: KS [51] and CS epitope WF6 [43]. Those biomarkers are first released into the synovial fluid and then into the blood stream via the lymph system [63]. In the studies concerned with joint clearance, a considerable amount of the labeled radioactive GAG that was injected into the joint cavity was released into the blood within a few hours [3]. Thus, the determination of those bio-molecular levels in the serum may allow assessment of the joint tissue metabolism. Regarding the GAG levels in OA, it was noted that horses with OA showed high GAG levels in the synovial fluid as well as in the serum [2]. The advantage of serum measurement is the ease of collecting the sample, and especially in small animals such as dogs or cats.
The diagnosis of OA is generally based on the clinical and radiographic changes that occur in the later stages of the disease. In the present study, we sought to investigate aggrecan (CS epitope 3B3 and WF6) and HA metabolism in HD dogs. We report here on the use of CS (3B3 epitope and WF6) and HA as biomarkers for OA in HD dogs. A novel monoclonal antibody (WF6), which recognizes a native epitope in CS chains, was evaluated together with using a monoclonal antibody 3B3, which recognizes unsaturated terminal chondroitin 6-sulfate after chondroitinase ABC digestion [13,15,49].
One hundred and twenty three native Thai dogs, 2-5 years old, were categorized into 2 groups. Ninety eight dogs were non-HD group consisting of 43 male and 55 female. They were 43.9 ± 11.2 months old and their body weight were 16.8 ± 6.1 kg. The other group had 25 dogs which was HD group consisting of 15 male and 10 female. They were 45.8 ± 10.2 months old and their weight were 17.8 ± 7.2 kg. The relationship between body weight and the serum biomarker was examined by dividing, the non-HD group into 5 subgroups according to their body weight: group 1 (less than 10 kg, n = 15), group 2 (from 10 kg to less than 15 kg, n = 30), group 3 (from 15 kg to less than 20 kg, n = 28), group 4 (from 20 kg to less than 25 kg, n = 15) and group 5 (greater than or equal to 25 kg, n = 10), respectively. However, HD group did not divide into subgroups and investigate the relationship between gender, weight and serum biomarker. Because of the biomarkers in all animals were changed according to the progress of disease.
All the animals had their age and weight recorded. In the non-HD group, 98 non-HD dogs were diagnosed based on signalments, physical examination and gait analysis [48], and they were radiographed using the standard position [15]; the phenotypic evaluation of the hips was done according to the Orthopedic Foundation for Animals (USA), in which the animals fall into seven different categories. Those categories are normal (excellent, good, fair), borderline, and dysplastic (mild, moderate, severe). The HD group consisted of 25 HD dogs that were diagnosed according to their clinical signs. Radiography was used for the final diagnosis. Briefly, for the radiographic evaluation, the dogs underwent radiographic examinations, and a ventrodorsal projection of the coxofemoral joints was retrieved. Evaluation for the proper radiographic technique was conducted. The diagnostic studies we considered were those in which the entire well-positioned pelvis was included. For these diagnostic studies, the obturator foramina were symmetrical and the femora were positioned to allow for accurate assessment of the femoral head and neck area. All the dogs in the HD group were classified as the severe grade. Briefly, for the radiographic findings of moderate to severe grade HD, there is significant subluxation present, where the femoral head is barely seated into the shallow socket, and this causes joint incongruence. There are secondary arthritic bone changes, usually along the femoral neck and head (termed remodeling), acetabular rim changes (termed osteophytes or bone spurs) and various degrees of trabecular bone pattern changes, and this is called sclerosis. For a severe grade of HD, there is significant subluxation present, where the femoral head is partly or completely dislocated from the shallow socket. There are also large amounts of secondary arthritic bone changes along the femoral neck and head, acetabular rim changes and large amounts of abnormal bone pattern changes.
Ten milliliter blood samples were collected from the cephalic vein of each dog. All the blood samples were taken in the morning before feeding the dogs. Two milliliters of the blood samples from each dog were kept in anticoagulant (100 IU/ml heprin; APS Finechem, Australia) for the complete blood count (CBC). Eight milliliters of the blood samples were centrifuged at 10,000 × g for 15 min to obtain the serum and this was kept frozen at -20℃ until blood chemical tests and biomarker assay were performed.
The biochemical analyses, CBCs and blood chemistry tests were conducted at the Small Animal Hospital, Faculty of Veterinary Medicine, Chiang Mai University, Chiang Mai, Thailand. The blood samples were analyzed for the CBC, including the hematocrit, the haemoglobin level, the red blood cell count and white blood cell count (WBC) and the platelet count. Two milliliters of serum were analyzed for blood chemicals, including aspartate aminotransferase, alanine aminotranferase, blood urea nitrogen and creatinine.
The biomarker assay that uses ELISA follows a previous study that was done by our research group [46,49,50]. Prior to performing competitive immunoassay with monoclonal antibody 3B3 (Seikagaku, Japan), the samples (175 µl of serum) were digested with using chondroitinase ABC (Sigma-Aldrich, USA) [an equal volume of 0.1 U/ml (in chondroitinase ABC buffer: 0.1 M sodium acetate, 1.0 M Tris-HCl, pH 7.3)]; they were incubated at 37℃ overnight, followed by heating at 100℃ for 10 min. The digested samples were spun in a microcentrifuge for 10 min to remove any precipitated protein and the supernatants were then collected and analysed.
The quantitative ELISA for the epitopes recognized by monoclonal antibody 3B3 was modified from an assay that was originally developed for synovial fluid [20]. The samples from the chondroitinase ABC-digested human serum were diluted in TE buffer (0.1 M Tris HCl (pH 7.4), 0.15 M sodium chloride, 0.1% Tween 20 and 0.1% BSA) and the samples were then were mixed with an equal volume of monoclonal antibody 3B3 (from ascites fluid, diluted 1:10,000 in TE buffer) in 1.5 ml plastic tubes; they were then incubated at 37℃ for 1 h. The samples were added to microplate wells that were previously coated with porcine laryngeal aggrecan core protein (100 µl/well: 77 ng/ml), and they were blocked with 1% BSA and then incubated at 37℃ for a further 1 h. The wells were washed 3 times with TE buffer, and peroxidase conjugated anti-mouse IgM antibody (Sigma-Aldrich, USA) was added (100 µl/well of a 1:1,000 dilution in TE buffer) and then the samples were allowed to incubate at 37℃ for a further 1 h. The bound peroxidase was detected by adding o-PD substrate (100 µl/well in citrate buffer, pH 5.0). The reaction was stopped with adding 50 µl/well of 4 M sulfuric acid and the absorbance was determined using a microplate reader at a dual wavelength of 492/690 nm. Measurement of the absorbance ratio at the two wavelengths reduced any well-to-well difference of non-specific interference, which can cause absorption at both wavelengths. The standard used was porcine aggrecan core protein (chondroitinase ABC-digested porcine laryngeal cartilage aggrecan) at various concentrations (4-2,000 ng/ml) The concentration of the 3B3(+) epitope in the supernatant samples was calculated from the standard curve.
A quantitative 2-step ELISA was developed based on the results from an initial study that characterised the epitopes recognized by monoclonal antibody WF6 [60]. Diluted human serum samples (1:5 in 6% BSA-TE buffer) were added to 1.5 ml plastic tubes that contained an equal volume of monoclonal antibody WF6 (cell culture supernatant, 1:200 dilution in the TE buffer). The standard we used was embryonic shark skeletal cartilage aggrecan (the A1D1 fraction) at different concentrations (19-10,000 ng/ml) of 6% BSA in the TE buffer. After incubation at 37℃ for 1 h, the samples (or standard) mixed with WF6 were added to the microtitre plate, which was previously coated with shark skeletal aggrecan (the A1 fraction) (100 µl/well: 10 ug/ml), and the samples were blocked with 1% BSA. The plates were incubated at 37℃ for 1 h and the wells were then washed with the TE buffer; peroxidase conjugated anti-mouse IgM antibody (Sigma-Aldrich, USA) was then added (100 ml/well; 1:2,000 dilution in the TE buffer). After incubation at 37℃ for a further 1 h, the amount of bound peroxidase was determined with using o-PD substrate (Sigma-Aldrich, USA) and the plates were read at 492/690 nm, as was described above. The concentration of the epitope WF6 in the samples was calculated from the standard curve.
An ELISA was developed for performing hyaluronan assay in serum, and this was based on previous work with HA binding proteins [32]. Human serum samples or standard HA (Healon; Pharmacia Pharmaceutical AB, Sweden) at various concentrations (19-10,000 ng/ml in 6% BSA-PBS pH 7.4) were mixed with an equal volume of bovine articular cartilage-biotinylated HABPs (1:200 in 0.05 M Tris-HCl buffer, pH 8.6). After incubation at room temperature for 1 h, the samples (100 µl) were added to microplate wells, which were previously coated with human umbilical cord HA (Sigma-Aldrich, USA) (100 µl/well of 10 µg/ml); they were then blocked with 1% BSA (150 µl/well). After further incubation at room temperature for 1 h, the wells were washed with PBS-Tween buffer, and peroxidase conjugated anti-biotin antibody (Zymed, USA) (1:2,000 dilution, 100 µl/well in PBS) was added next. The plate was incubated at room temperature for a further 1 h and the bound peroxidase was determined with using o-PD substrate. The plates were read at 492/690 nm, as was described above. The amount of HA in the samples was calculated from the standard curve.
The CS and HA data from the serum are reported as means ± SD. The non-parametric 2-sample Mann-Whitney procedure was used to test for differences between the non-HD and HD groups. Paired t-tests were performed to test the (one-tailed hypothesis that 1) the biomarker levels (CS and HA) depended on the dogs' weights in the non-HD group and 2) the biomarker levels in the males were different from that in the females. The relative data was analyzed using the Statistical Analysis System version 8.0 (SAS Institute, USA) software package. p values less than 0.05 were considered to be significant.
Table 1 shows the mean values for non-HD and HD group. Most of the values were not significantly different between the non-HD and HD group (p > 0.05). The WBC in the HD group was increased when compared to that of the non-HD groups (p < 0.05). This increase came from the total number of neutrophils, which was significantly higher in the HD group (p < 0.05), while the other cell numbers (lymphocytes, monocytes, eosinophils and basophils) were not different between the groups (p > 0.05).
All the dogs in the HD group were diagnosed as having hip dysplatic, but they were not categorized into different pathological stages (mild, moderate, severe). The level of CS epitope 3B3 in the HD group (105 ± 100.05 ng/ml) was significantly (p < 0.05) lower than that in the non-HD group (136 ± 142.03 ng/ml). Yet the level of CS epitope WF6 in the HD group (2,594 ± 3,036.10 ng/ml) was significantly (p < 0.01) higher than that in the non-HD group (465 ± 208.97 ng/ml). The level of HA in the HD group (134.74 ± 59.71 ng/ml) was lower than that in the non-HD group (245.45 ± 97.84 ng/ml) (p < 0.05), as is shown in Fig. 1.
The average body weight in each subgroup was significantly different (p < 0.05, data not shown). Most of the HD dogs (44%) were 20-25 kg for their body weight, and none were less than 10 kg. The level of all the biomarkers in the different weight subgroups was not significantly different (p > 0.05) among the 5 subgroups (Fig. 2). Moreover, those biomarker levels were also not significantly different (p > 0.05) between the male and female dogs (Fig. 3).
The present study showed that HD dogs have the highest level of serum CS epitopes WF6, which means the cartilage in the HD joint was degraded and it developed into OA. We found the level of CS epitope WF6 in the HD group to be 150% higher, while epitope 3B3 in the HD group was 30% lower than that of the non-HD group. In agreement with the review by Nganvongpanit and Ong-Chai [43] the levels of those biomarkers in chronic OA changed in the same way. The study that was done by Peansukmanee et al. [47] found that the level of CS epitope WF6 was higher in OA horses, but the CS epitope 3B3 was lower when compared with that of the non-OA horses. Moreover, the level of CS epitope 3B3 rose not only in the early phase of OA, but also in young animals as compared with the mature animals [47]. It can be stated that the CS epitope 3B3 responds to the cartilage anabolism. Coinciding with these previous results, the level of CS epitope 3B3 in the HD dogs was lower than that in the non-HD dogs. This shows that the metabolism of articular cartilage in HD doesn't involve the synthesis of new CS molecules. Hence, the cartilages become highly degraded and this then causes severe disease.
HA is one of the ECM molecules of the articular cartilage. Previous studies have shown that HA can be used as a biomarker in OA dogs [4,10,34] and rheumatoid disease [4,46]. Our study demonstrates for the first time the determination of the serum HA levels in HD dogs. We observed that the level of HA in the HD group was 45% lower than that in the non-HD group. This result is the same as the previous work on inflammatory joint disease: the HA concentration in the joint fluid and serum of animals with diseased joints has been reported to be lower than normal [14,35]. However, many studies have shown the relationship between the HA level in serum and liver disease [18,60] because the major site of metabolism for circulating HA is the liver. The lysosomes of the liver endothelial cells produce the enzymes hyaluronidase, β-glucuronidase and β-N-acetylglucosaminidase, and these are responsible for metabolizing HA to monosaccharides [57,58]. The level of serum HA was elevated when the function of the liver was interrupted by disease or chemicals [55]. To avoid these effects, we investigated the CBC and blood chemicals in both the HD and non-HD dogs, and abnormal liver signs were observed in both groups. The results indicated that the level of serum HA in our study is a direct effect of cartilage metabolism and is not a result of liver function.
From the serological results, the WBC in the HD group was significantly higher than that in the non-HD group. Based on our knowledge, interleukin (IL-1) is mainly produced by cells of the macrophage lineage such as the synovial A-cell. Moreover, the IL-1 from synovial A-cells and other macrophage cells stimulates chondrocytes to produce IL-1 [7]. An increasing of IL-1 stimulates the production of collagenase, stromelysin and prostaglandin E2 by the chondrocytes. This mechanism induces inflammation to proceed in the dysplatic joint [8,24] and it increases the WBC. So far, this study supports those previous publications that were mention above. Our study found that the mean value of the WBC in the HD group was significantly higher than that in the non-HD group. However, the biological significance of the WBC in HD-dog needs future study to expand our understanding of the interaction between the WBC and HD-dogs.
It is interesting to compare the results for the serum HA with the result for the CS epitopes (3B3 and WF6). The CS epitope 3B3 may provide a measure of the mobilization of the tissue proteoglycans that contain chondroitin 6-sulfate. The 3B3 epitope is a 6-sulfated terminal disaccharide of CS [15]. The 3B3 assay that was developed in this study with using monoclonal antibody recognizes the unsaturated terminal 6-sulfateed disaccharide structure that remains attached to the protein after chondroitinase ABC digestion. As the aggrecan degradation products from cartilage were likely to be present in the serum and to be polyvalent, the detection with 3B3 epitope may selectively provide a measure of anabolism in the cartilaginous tissues. The level of native CS epitope detected by monoclonal antibody WF6 in the HD group was increased above the level found in the non-HD group. An increase in the WF6 epitope may reflect a catabolic response and this may be helpful for making the diagnosis or predicting the prognosis of disease.
Moreover, this study shows the advantage of using these biomarkers. Body weight, which is known as a predisposing cause of OA [59], is not affected by the level of these biomarkers. This means that the changes of the biomarkers' levels in serum are dependent on the severity of disease. Also, a previous study from our group demonstrated that the levels of CS epitope WF6 and 3B3 were not different between different body weight groups of horses [47]. This shows that the change of biomarker levels in the serum is directly related to the cartilage metabolism. However, the relationship between the severity of disease and the change of those biomarkers or other factors (such as the disease management and breed of animal) needs to be investigated for enlightening our understanding of the pathogenesis of the articular cartilage in HD disease.
In conclusion, our study raises important questions concerning the alterations in cartilage aggrecan metabolism in HD dogs. We have demonstrated the potential for using measurements of aggrecan epitopes and HA in the serum as indicators of disease activity. Indeed, the results of the present study suggest that several target biomarker molecules of cartilage metabolism have the potential to provide clinically useful indices of the effects of isolated joint injury, the progression of the joint changes and the response to therapy. The levels of the CS epitopes 3B3 and WF6 in serum were increased, while the HA levels show a decrease in hip dysplatic dogs. This information may prove useful for making the differential diagnosis and monitoring joint disease.
Figures and Tables
Fig. 1
The levels of serum chondroitin sulfate epitope 3B3 (A) and WF6 (B) and hyaluronan (C) in the non-hip dysplasia (HD) group compared to the HD group. Data show the mean ± SD. *The significant difference (p < 0.05) between the non-HD and HD groups.
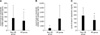
Fig. 2
The levels of serum chondroitin sulfate epitope 3B3 (A) and WF6 (B) and hyaluronan (C) in the non-hip dysplasia group. This group was categorized into 5 different subgroup due to body weight such as group 1 (less than 10 kg, n = 15), group 2 (from 10 kg to less than 15 kg, n = 30), group 3 (from 15 kg to less than 20 kg, n = 28), group 4 (from 20 kg to less than 25 kg, n = 15) and group 5 (greater than or equal to 25 kg, n = 10). No significant difference (p > 0.05) was observed among the subgroups.
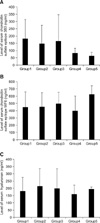
Fig. 3
The levels of serum chondroitin sulfate epitope 3B3 (A), WF6 (B) and hyaluronan (C) in the non-HD group. The animals of this group were categorized into the male and female group. Data show the mean ± SD. No significant difference (p > 0.05) was observed between the genders.
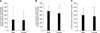
Table 1
Comparison of complete blood counts and blood chemistry between the non-hip dysplasia (HD) and HD groups
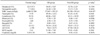
*The significant difference (p < 0.05) between the non-HD and HD groups. †Cited from Kaewsakorn et al. [26]. ‡The p-values between the HD and non-HD groups. AST: aspartate aminotransferase, ALT: alanine aminotranferase, BUN: blood urea nitrogen. Data are expressed as mean ± SD.
Acknowledgments
The authors express their gratitude and thanks to the veterinarians and technician assistants at the Small Animal Hospital, Faculty of Veterinary Medicine, Chiang Mai University, for collecting the samples. This project was supported by The Thailand Research Fund (TRF Advanced Research Scholar, Grant # BRG 4780018 to PK), The National Research Council of Thailand (Research program for drugs, chemicals, medical materials and equipment) and the Faculty of Veterinary Medicine, Chiang Mai University, Thailand.
References
1. Allan G. Thrall DE, editor. Radiographic signs of joint disease. Textbook of Veterinary Diagnostic Radiology. 1994. 2nd ed. Philadelphia: Saunders;130–150.
2. Alwan WH, Carter SD, Bennett D, Edwards GB. Glycosaminoglycans in horses with osteoarthritis. Equine Vet J. 1991. 23:44–47.
3. Antonas KN, Fraser JR, Muirden KD. Distribution of biologically labelled radioactive hyaluronic acid injected into joints. Ann Rheum Dis. 1973. 32:103–111.


4. Arican M, Carter SD, May C, Bennett D. Hyaluronan in canine arthropathies. J Comp Pathol. 1994. 111:185–195.


5. Belcher C, Yaqub R, Fawthrop F, Bayliss M, Doherty M. Synovial fluid chondroitin and keratan sulphate epitopes, glycosaminoglycans, and hyaluronan in arthritic and normal knees. Ann Rheum Dis. 1997. 56:299–307.


6. Björk J, Kleinau S, Tengblad A, Smedegård G. Elevated levels of serum hyaluronate and correlation with disease activity in experimental models of arthritis. Arthritis Rheum. 1989. 32:306–311.


7. Blom AB, van der Kraan PM, van den Berg WB. Cytokine targeting in osteoarthritis. Curr Drug Targets. 2007. 8:283–292.


8. Bondeson J, Wainwright SD, Lauder S, Amos N, Hughes CE. The role of synovial macrophages and macrophage-produced cytokines in driving aggrecanases, matrix metalloproteinases, and other destructive and inflammatory responses in osteoarthritis. Arthritis Res Ther. 2006. 8:R187.
9. Bruyere O, Collette JH, Ethgen O, Rovati LC, Giacovelli G, Henrotin YE, Seidel L, Reginster JY. Biochemical markers of bone and cartilage remodeling in prediction of longterm progression of knee osteoarthritis. J Rheumatol. 2003. 30:1043–1050.
10. Budsberg SC, Lenz ME, Thonar EJ. Serum and synovial fluid concentrations of keratan sulfate and hyaluronan in dogs with induced stifle joint osteoarthritis following cranial cruciate ligament transection. Am J Vet Res. 2006. 67:429–432.


11. Cardinet GH 3rd, Kass PH, Wallace LJ, Guffy MM. Association between pelvic muscle mass and canine hip dysplasia. J Am Vet Med Assoc. 1997. 210:1466–1473.
12. Caterson B, Flannery CR, Hughes CE, Little CB. Mechanisms involved in cartilage proteoglycan catabolism. Matrix Biol. 2000. 19:333–344.


13. Caterson B, Griffin J, Mahmoodian F, Sorrell JM. Monoclonal antibodies against chondroitin sulphate isomers: their use as probes for investigating proteoglycan metabolism. Biochem Soc Trans. 1990. 18:820–823.


14. Caterson B, Hughes CE, Roughley P, Mort JS. Anabolic and catabolic markers of proteoglycan metabolism in osteoarthritis. Acta Orthop Scand Suppl. 1995. 266:121–124.


15. Caterson B, Mahmoodian F, Sorrell JM, Hardingham TE, Bayliss MT, Carney SL, Ratcliffe A, Muir H. Modulation of native chondroitin sulphate structure in tissue development and in disease. J Cell Sci. 1990. 97:411–417.


16. DeAngelis PL. Hyaluronan synthases: fascinating glycosyltransferases from vertebrates, bacterial pathogens, and algal viruses. Cell Mol Life Sci. 1999. 56:670–682.


17. Fuller CJ, Barr AR, Sharif M, Dieppe PA. Cross-sectional comparison of synovial fluid biochemical markers in equine osteoarthritis and the correlation of these markers with articular cartilage damage. Osteoarthritis Cartilage. 2001. 9:49–55.


18. George J, Stern R. Serum hyaluronan and hyaluronidase: very early markers of toxic liver injury. Clin Chim Acta. 2004. 348:189–197.


19. Goldberg RL, Rubin AS. Serum hyaluronate as a marker for disease severity in the Lactobacillus casei model of arthritis in the rat. J Rheumatol. 1989. 16:92–96.
20. Hazell PK, Dent C, Fairclough JA, Bayliss MT, Hardingham T. Changes in glycosaminoglycan epitope levels in knee joint fluid following injury. Arthritis Rheum. 1995. 38:953–959.


21. Hegemann N, Kohn B, Brunnberg L, Schmidt MF. Biomarkers of joint tissue metabolism in canine osteoarthritic and arthritic joint disorders. Osteoarthritis Cartilage. 2002. 10:714–721.


22. Heinegård D, Hascall VC. Aggregation of cartilage proteoglycans. 3. Characteristics of the proteins isolated from trypsin digests of aggregates. J Biol Chem. 1974. 249:4250–4256.
23. Hollander AP, Heathfield TF, Webber C, Iwata Y, Bourne R, Rorabeck C, Poole AR. Increased damage to type II collagen in osteoarthritic articular cartilage detected by a new immunoassay. J Clin Invest. 1994. 93:1722–1732.


24. Jacques C, Gosset M, Berenbaum F, Gabay C. The role of IL-1 and IL-1Ra in joint inflammation and cartilage degradation. Vitam Horm. 2006. 74:371–403.


25. Johnson JA, Austin C, Breur GJ. Incidence of canine appendicular musculoskeletal disorders in 16 veterinary teaching hospitals from 1980 through 1989. Vet Comp Orth Traum. 1994. 7:56–59.


26. Kaewsakorn T, Chotayaporn M, Suwankong N, Nganvongpanit K, Jitpean S, Cutesuwan P. Normal hematology and blood chemistry in small, medium and larged adult healthy dogs. Chiangmai Vet J. 2003. 1:35–49.
27. Kelly GS. The role of glucosamine sulfate and chondroitin sulfates in the treatment of degenerative joint disease. Altern Med Rev. 1998. 3:27–39.
28. Kiani C, Chen L, Wu YJ, Yee AJ, Yang BB. Structure and function of aggrecan. Cell Res. 2002. 12:19–32.


29. Kikuchi T, Yamada H, Shimmei M. Effect of high molecular weight hyaluronan on cartilage degeneration in a rabbit model of osteoarthritis. Osteoarthritis Cartilage. 1996. 4:99–110.


30. Knudson CB, Knudson W. Hyaluronan-binding proteins in development, tissue homeostasis, and disease. FASEB J. 1993. 7:1233–1241.


31. Knudson W. The role of CD44 as a cell surface hyaluronan receptor during tumor invasion of connective tissue. Front Biosci. 1998. 1:d604–d615.


32. Kongtawelert P, Ghosh P. An enzyme-linked immunosorbent-inhibition assay for quantiation of hyaluronan (hyaluronic acid) in biological fluids. Anal Biochem. 1989. 178:367–372.


33. Leighton EA. Genetics of canine hip dysplasia. J Am Vet Med Assoc. 1997. 210:1474–1479.
34. Leipold HR, Goldberg RL, Lust G. Canine serum keratan sulfate and hyaluronate concentrations. Relationship to age and osteoarthritis. Arthritis Rheum. 1989. 32:312–321.


35. Lohmander LS. Markers of cartilage metabolism in arthrosis. A review. Acta Orthop Scand. 1991. 62:623–632.
36. Lohmander LS, Dahlberg L, Eyre D, Lark M, Thonar EJ, Ryd L. Longitudinal and cross-sectional variability in markers of joint metabolism in patients with knee pain and articular cartilage abnormalities. Osteoarthritis Cartilage. 1998. 6:351–361.


37. Lohmander LS, Ionescu M, Jugessur H, Poole AR. Changes in joint cartilage aggrecan after knee injury and in osteoarthritis. Arthritis Rheum. 1999. 42:534–544.


38. Lust G. An overview of the pathogenesis of canine hip dysplasia. J Am Vet Med Assoc. 1997. 210:1443–1445.
39. Manicourt DH, Pita JC. Quantification and characterization of hyaluronic acid in different topographical areas of normal articular cartilage from dogs. Coll Relat Res. 1988. 8:39–47.


40. Masuhara K, Nakai T, Yamaguchi K, Yamasaki S, Sasaguri Y. Significant increases in serum and plasma concentrations of matrix metalloproteinases 3 and 9 in patients with rapidly destructive osteoarthritis of the hip. Arthritis Rheum. 2002. 46:2625–2631.


41. May SA. Holton JEF, Collinson R, editors. Degenerative joint disease (osteoartritis), osteoartrosis, secondary joint disease. Manual of Small Animal Arthrology. 1994. Bournemounth, Cheltenham: British Small Animal Veterinary Association;62–74.
42. McLaughlin RM, Roush JK. Diagnosing osteoarthritis. Vet Med. 2002. 97:120–133.
43. Nganvongpanit K, Ong-Chai S. Biological marker for canine osteoarthritis diagnosis. Chiangmai Vet J. 2004. 2:39–49.
44. Okumura M, Tagami M, Fujinaga T. Measurement of serum and synovial fluid keratan sulphate and antibody to collagen type II in equine osteoarthritis. Zentralbl Veterinarmed A. 1998. 45:513–516.


45. Owens JM, Biery DN. Radiographic Interpretation for the Small Animal Clinician. 1999. 2nd ed. Baltimore: Williams & Wilkins.
46. Paimela L, Heiskanen A, Kurki P, Helve T, Leirisalo-Repo M. Serum hyaluronate level as a predictor of radiologic progression in early rheumatoid arthritis. Arthritis Rheum. 1991. 34:815–821.


47. Peansukmanee S, Trinarong C, Kongtawelert P, Ongchai S. Chondroitin sulfate epitopes in sera of normal and osteoarthritic horses. Chiangmai Vet J. 2003. 1:3–10.
48. Petersson IF, Boegård T, Svensson B, Heinegård D, Saxne T. Changes in cartilage and bone metabolism identified by serum markers in early osteoarthritis of the knee joint. Br J Rheumatol. 1998. 37:46–50.


49. Pothacharoen P, Siriaunkgul S, Ong-Chai S, Supabandhu J, Kumja P, Wanaphirak C, Sugahara K, Hardingham T, Kongtawelert P. Raised serum chondroitin sulfate epitope level in ovarian epithelial cancer. J Biochem. 2006. 140:517–524.


50. Pothacharoen P, Teekachunhatean S, Louthrenoo W, Yingsung W, Ong-Chai S, Hardingham T, Kongtawelert P. Raised chondroitin sulfate epitopes and hyaluronan in serum from rheumatoid arthritis and osteoarthritis patients. Osteoarthritis Cartilage. 2006. 14:299–301.


51. Ratcliffe A, Beauvais PJ, Saed-Nejad F. Differential levels of synovial fluid aggrecan aggregate components in experimental osteoarthritis and joint disuse. J Orthop Res. 1994. 12:464–473.


52. Ratcliffe A, Billingham MEJ, Saed-Nejad F, Muir H, Hardingham TE. Increased release of matrix components from articular cartilage in experimental canine osteoarthritis. J Orthop Res. 1992. 10:350–358.


53. Rettenmaier JL, Keller GG, Lattimer JC, Corley EA, Ellersieck MR. Prevalence of canine hip dysplasia in a veterinary teaching hospital population. Vet Radiol Ultrasound. 2002. 43:313–318.


54. Roush JK, McLaughlin RM, Radlinsky MAG. Understanding the pathophysiology of osteoarthritis. Vet Med. 2002. 97:108–119.
55. Sakugawa H, Nakayoshi T, Kobashigawa K, Yamashiro T, Maeshiro T, Miyagi S, Shiroma J, Toyama A, Nakayoshi T, Kinjo F, Saito A. Clinical usefulness of biochemical markers of liver fibrosis in patients with nonalcoholic fatty liver disease. World J Gastroenterol. 2005. 11:255–259.


56. Simánek V, Kren V, Ulrichová J, Gallo J. The efficacy of glucosamine and chondroitin sulfate in the treatment of osteoarthritis: Are these saccharides drugs or nutraceuticals? Biomed Pap Med Fac Univ Palacky Olomouc Czech Repub. 2005. 149:51–56.


57. Smedsrød B, Kjellén L, Pertoft H. Endocytosis and degradation of chondroitin sulphate by liver endothelial cells. Biochem J. 1985. 229:63–71.


58. Smedsrød B, Pertoft H, Gustafson S, Laurent TC. Scavenger functions of the liver endothelial cell. Biochem J. 1990. 266:313–327.


59. Smith GK, Mayhew PD, Kapatkin AS, Mckelvie PJ, Shofer FS, Gregor TP. Evaluation of risk factors for degenerative joint disease associated with hip dysplasia in German shepherd dogs, Golden retrievers, Labrador retrievers, and Rottweilers. J Am Vet Med Assoc. 2001. 219:1719–1724.


60. Tangkijvanich P, Kongtawelert P, Pothacharoen P, Mahachai V, Suwangool P, Poovorawan Y. Serum hyaluronan: a marker of liver fibrosis in patients with chronic liver disease. Asian Pac J Allergy Immunol. 2003. 21:115–120.
61. Uesaka S, Nakayama Y, Shirai Y, Yoshihara K. Serum and synovial fluid levels of chondroitin sulfate in patients with osteoarthritis of the knee joint. J Nippon Med Sch. 2001. 68:165–170.

