Abstract
Pigs are the most likely source animals for cardiac xenotransplantation. However, an appropriate method for estimating the cardiac function of micropigs had not been established. Computed tomography (CT) analysis aimed at estimating cardiac function and assessing the coronary arteries has not been carried out in micropigs. This study determined the feasibility of evaluating cardiac function in a micropig model using multidetector row computed tomography (MDCT) and compared the cardiac function values with those of conventional pigs. The mean age of the conventional pigs and micropigs was approximately 80 days and approximately 360 days, respectively. The mean body weight in the conventional pigs and micropigs was 29.70 ± 0.73 and 34.10 ± 0.98 kg, respectively. Cardiac MDCT detected ejection fractions of 52.93 ± 3.10% and 59.00 ± 5.56% and cardiac outputs of 1.46 ± 0.64 l/min and 1.21 ± 0.24 l/min in conventional pigs and micropigs, respectively. There were no significant differences in cardiac function between conventional pigs and micropigs in the reconstructed CT images. There were also no differences in the coronary angiographic images obtained by MDCT. It is expected that the results of this study will help improve understanding of cardiac function in micropigs. The data presented in this study suggest that MDCT is a feasible method for evaluating cardiac function in micropigs.
Transplantation is often used to treat fulminant organ failure. However, severe shortages in the availability of suitable human donors have limited the volume of heart transplants [15]. This shortage of donors has stimulated interest in the possibility of using animal organs for transplantation into humans. Animal-to-human transplantation (or xenotransplantation) would offer an unlimited supply of organs and tissue for transplantation. Both non-human primates and non-primate mammals have been used as sources for heart transplants. Non-human primates such as chimpanzees and baboons are closely related to humans phylogenetically and share many immunological properties with humans [3,5,25]. However, non-human primates are unlikely to be a fruitful source of organs in the future for the following reasons: slow growth rate, limited offspring, difficulty breeding in captivity, and smaller size [27]. Pigs are now considered to be the most likely source animals for human xenotransplantation in the future. They have several advantages over non-human primates. The heart size of miniature swine including the micropig is compatible with the human heart, and cardiovascular function and hemodynamic parameters are also similar [2]. In addition, the reproduction-related features of pigs, such as early sexual maturity, short gestation time, and generation of large litters, allow for a potentially large pool of animal donors for xenotransplantation [37].
One essential question in xenotransplantation is whether the animal organ can be an effective physiological proxy for the human organ. In order to answer to this question, the functional and anatomical compatibility of a pig heart and its human counterpart has been investigated [35]. The cardiac output of porcine and human hearts of similar size was found to be comparable [19], and their action potentials were also similar [35]. Despite previous study, most physiological incompatibilities and species-specific differences between mammalian species remain unknown. Moreover, even individuals of one species or strain may exhibit slight genetically-derived metabolic differences [13]. These differences have the potential to create problems for support of the human cardiovascular system after xenotransplantation.
It is clinically important to measure cardiac function in individual micropigs used for heart transplantation for purposes of diagnosis and prognosis. The indices of a healthy heart are verified through assessment of ejection fraction (EF), end systolic volume (ESV), end diastolic volume (EDV), cardiac output (CO), and coronary artery angiography [32,36]. However, there is no reliable method for evaluating these parameters in micropigs. Therefore, we studied the feasibility of evaluating cardiac function in micropigs using multidetector row computed tomography (MDCT) and compared the parameters with those of conventional pigs.
In recent years, major technological improvements have been achieved in computed tomography (CT). The most significant development has been the introduction of MDCT, which has brought about substantial improvements in spatial and temporal resolution [12,17]. This study is the first to show MDCT to be a reliable method for assessing cardiac function in micropigs and for selecting suitable donor pigs for heart xenotransplantation.
All experimental procedures were approved by the Ethics Committee of Chonnam National University Hospital. Studies were performed using mixed-breed, conditioned Yucatan micropigs and Landrace breed conventional pigs, all of which were provided by the animal breeding house of Chonnam National University Research Institute of Medical Sciences. The pigs were housed individually indoors in cages, fed dry pig food, and provided with water. The mean age was approximately 80 days for conventional pigs and approximately 360 days for micropigs. The mean body weight for the conventional pigs and micropigs was 29.70 ± 0.73 kg and 34.10 ± 0.98 kg, respectively.
After premedication with azaperone (0.5 mg/kg, intramuscular) and xylazine (8 mg/kg, intramuscular), normal saline with midazolam (0.2 mg/kg) was infused through a 20-G venous access line placed in an ear vein. CT examinations were performed using a two-phase, contrast-enhanced, ECG-gated, MDCT scanner (SOMATOM Sensation Cardiac 64; Siemens, Germany) set at a 0.75-mm section thickness, with a gantry rotation time of 330 msec and a kernel value of B25f. The tube current was 800 mAs at 120 kVP. The pitch, which is defined as the ratio of the table feed in a single rotation over the detector coverage in the transverse direction, was determined to be 0.2. Serial CT scanning in the axial plane, together with an ECG-triggered examination, was performed from the level of the left ventricular apex after a bolus injection of 60 ml of non-ionic contrast media (Ultravist 370; Schering, Germany) followed by a 60 ml saline bolus injection through the ear vein. Both were injected at a flow rate of 4 ml/sec. Axial images were reconstructed at multiple phases that covered the cardiac cycle in 10% increments of the RR interval between 5% and 95%. Multiphase reconstruction was performed with commercially available software (Argus; Siemens, Germany) by using short axis slices from the base of the heart to the apex. The end-diastole and end-systole were defined as the maximal and minimal left ventricular volume, respectively. The EDV, ESV, LVEF, stroke volume (SV), CO, and myocardial mass were compared between the two groups. A 7 Fr. arterial sheath was placed in the left carotid artery after achieving local anesthesia with 2% lidocaine, and a cutdown was made. After infusing 10,000 units of heparin, a 7 Fr. coronary artery guiding catheter was placed within the ostia of the left and right coronary arteries under fluoroscopic guidance using a mobile C-arm (Phillips BV-25 Gold; Phillips, Netherlands). Coronary angiography demonstrated left and right coronary arteries with branches. The coronary angiograms for the left and right coronary arteries and their branches were compared with the images of the CT angiogram. During the experiment, oxygen and normal saline were supplied continuously, and anesthesia was maintained with an additional infusion of midazolam. Continuous ECG monitoring was performed in order to confirm that the pigs had a normal ST segment at baseline.
Statistical analysis was carried out with Statistical Package for Social Sciences software (SPSS 12.0 for Windows; SPSS, USA). Continuous variables with normal distributions were compared using an unpaired Student's t-test and are expressed as mean ± SD. Where appropriate, categorical variables were compared using a Wilcoxon test. A p-value < 0.05 was considered significant.
The end-systolic and end-diastolic phase images were displayed (Figs. 1 and 2), and EDV, ESV, EF, SV, CO, and myocardial mass were calculated automatically (Tables 1 and 2). The EDV and ESV were 48.80 ± 23.30 ml and 22.97 ± 11.30 ml in conventional pigs and 36.70 ± 9.36 ml and 13.30 ± 7.43 ml in micropigs under a pre-medicated condition, respectively. There were no significant differences in MDCT-measured parameters between conventional pigs and micropigs, including SV (25.83 ± 12.11 ml vs. 23.40 ± 2.26 ml), CO (1.46 ± 0.64 l/min vs. 1.21 ± 0.24 l/min), and myocardial mass (41.83 ± 19.47 g vs. 37.03 ± 6.89 g) (Table 1). The values measured in conventional pigs had broader variation relative to those of micropigs. This is likely due to the fact that the conventional pigs used in the present experiment were mixed-breed and were not fully grown. There was no significant difference in the measured EF, which was 52.93 ± 3.10% in conventional pigs and 59.00 ± 5.56% in micropigs. Furthermore, the EF measured by MDCT was not significantly different than the EF value detected by echocardiography (65.47 ± 5.17% vs. 58.40 ± 8.18%) (Table 2). The small differences between the MDCT and echocardiography values are likely due to differences in individual characteristics, body weights, ages, conditions of evaluation, and modalities. The coronary circulation, as revealed by CT angiogram, was similar between conventional pigs and micropigs (Fig. 3).
Cardiac mortality is closely related to cardiac volumes and global left ventricular function, which is expressed as the LVEF [36]. An accurate assessment of these parameters is essential for determining prognosis in micropigs used for xenotransplantation, as well as in individual patients. Measures of cardiac function should be carried out using modalities that provide quick, noninvasive images with superior temporal and spatial resolution. To date, cardiac functional assessment has been performed using various noninvasive modalities, such as echocardiography [7,29], nuclear medicine [6], single-detector row helical CT [26], MDCT [12,17], electron beam CT [23], and magnetic resonance imaging (MRI) [28].
Over the past few years, MDCT has become more popular for noninvasive cardiac imaging [1,30]. Initially, MDCT was used to detect coronary artery stenosis and to assess cardiac morphology [14,31]. Because data acquisition in MDCT is continuous, retrospective ECG-gating allows image reconstruction in any phase of the cardiac cycle. Therefore, an end-systolic and end-diastolic image can be produced to assess the ventricular volume and function. The LV volume measurement from the ECG-gated MDCT image shows a good correlation with cardiac MRI, which is accepted as the reference method for a precise quantitative LV functional analysis [24,38].
In this study, there were no significant differences in left ventricular end systolic volume (LV-ESV) or end diastolic volume (LV-EDV) between conventional pigs and micropigs. This result correlates well with a previous study that compared the cardiac function between the two strains using echocardiography and radiography [22]. In previous evaluations of cardiac function, MDCT with a temporal resolution of 125-250 ms was shown to be comparable to echocardiography [9,34], single photon emission CT [38], and MRI. Over the last few years, rapid technical developments in scanner hardware have led to improvements in spatial and temporal resolution, as well as led to significantly faster cardiac scans. Consequently, MDCT has become an attractive option for evaluating coronary artery obstruction and assessing ventricular function. According to a previous study that estimated the EF in humans using MDCT, MRI, and echocardiography, the EF by echocardiography, MDCT, and MRI was 54.6 ± 16.7%, 50.7 ± 16.0%, and 51.8 ± 15.9%, respectively [38]. This suggests that there are no significant differences in cardiac function between humans and micropigs.
A few studies comparing MDCT LV functional measurements with ventriculography, MRI, and transthoracic echocardiography (TTE) have reported a good correlation among these modalities [10,11,18]. Moreover, in studies focused on visual analysis and quantification of regional LV function [8,16,33], wall-motion anomalies were accurately identified with regard to TTE and MR. The reproducibility of global function parameters in MDCT appears to be comparable to that seen in other modalities. The reported inter-observer variability has ranged from 2% to 11% for LV-EDV and from 6% to 9% for LV-ESV. The corresponding values for MR are 2% to 6% [4]. MDCT allows imaging of the coronary arteries with a high temporal and spatial resolution. In recent years, there has been continuous improvement in the capability of both technologies for the visualization of the coronary lumen and the detection of coronary artery stenosis. The sensitivity of MDCT for the detection of significant stenosis ranges from 72% to 95%, with specificity ranging from 84% to 97% [1,20,21]. In conclusion, MDCT is a reliable modality for evaluating cardiac function in healthy micropigs.
Figures and Tables
Fig. 1
Left ventriculogram by computerized tomography (A: end-systolic phase, B: end-diastolic phase) in a conventional pig.
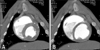
Fig. 2
Left ventriculogram by computerized tomography (A: end-systolic phase, B: end-diastolic phase) in a micropig.
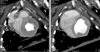
Fig. 3
Coronary circulation by computerized tomography in a conventional pig (A) and a micropig (B). Arrow; right circumflex artery (RCA). Arrowhead; left anterior descending artery (LAD). Arrow with dotted line; left circumflex artery (LCX).
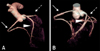
Table 1
Cardiac parameters in conventional pigs and micropigs as measured by multidetector row computed tomography
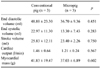
Table 2
Comparison of ejection fraction (EF) detected by multidetector row computed tomography (MDCT) and echocardiography in conventional pigs and micropigs
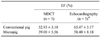
*Cited from Lee et al. [22].
Acknowledgments
The authors wish to thank Drs. Yong Sook Kim, Jin Sook Kwon, Jae Young Cho, Ki Hong Lee, Woo Seok Lee, and Dae Ho Jeong for their technical support in acquiring the cardiac MDCT images and data analysis. This work was supported by a grant (code # 20070401034006) from the BioGreen 21 Program, Rural Development Administration, Korea. The authors acknowledge a graduate fellowship provided by the Ministry of Education, Science and Technology through the Brain Korea 21 project, Korea.
References
1. Achenbach S, Giesler T, Ropers D, Ulzheimer S, Derlien H, Schulte C, Wenkel E, Moshage W, Bautz W, Daniel WG, Kalender WA, Baum U. Detection of coronary artery stenoses by contrast-enhanced, retrospectively electrocardiographically-gated, multislice spiral computed tomography. Circulation. 2001. 103:2535–2538.


2. Appel JZ 3rd, Buhler L, Cooper DK. The pig as a source of cardiac xenografts. J Card Surg. 2001. 16:345–356.


3. Balner H, van Leeuwen A, van Vreeswijk W, Dersjant H, van Rood JJ. Leukocytes antigens of chimpanzees and their relation to human HL-A antigens. Transplant Proc. 1970. 2:454–462.
4. Barkhausen J, Ruehm SG, Goyen M, Buck T, Laub G, Debatin JF. MR evaluation of ventricular function: true fast imaging with steady-state precession versus fast low-angle shot cine MR imaging: feasibility study. Radiology. 2001. 219:264–269.


5. Barnes AD, Hawker RJ. Leukocyte antigens in baboons: a preliminary to tissue typing for organ grafting. Transplant Proc. 1972. 4:37–42.
6. Bavelaar-Croon CDL, Kayser HWM, van der Wall EE, de Roos A, Dibbets-Schneider P, Pauwels EKJ, Germano G, Atsma DE. Left ventricular function: correlation of quantitative gated SPECT and MR imaging over a wide range of values. Radiology. 2000. 217:572–575.


7. Buck T, Hunold P, Wentz KU, Tkalec W, Nesser HJ, Erbel R. Tomographic three-dimensional echocardiographic determination of chamber size and systolic function in patients with left ventricular aneurysm: comparison to magnetic resonance imaging, cineventriculography, and two-dimensional echocardiography. Circulation. 1997. 96:4286–4297.


8. Dirksen MS, Bax JJ, de Roos A, Jukema JW, van der Geest RJ, Geleijns J, van der Wall EE, Lamb HJ. Images in cardiovascular medicine. Dynamic multislice computed tomography of left ventricular function. Circulation. 2004. 109:e25–e26.
9. Dirksen MS, Bax JJ, de Roos A, Jukema JW, van der Geest RJ, Geleijns K, Boersma E, van der Wall EE, Lamb HJ. Usefulness of dynamic Multislice computed tomography of left ventricular function in unstable angina pectoris and comparison with echocardiography. Am J Cardiol. 2002. 90:1157–1160.


10. Fisher MR, von Schulthess GK, Higgins CB. Multiphasic cardiac magnetic resonance imaging: normal regional left ventricular wall thickening. AJR Am J Roentgenol. 1985. 145:27–30.


11. Germano G, Erel J, Lewin H, Kavanagh PB, Berman DS. Automatic quantitation of regional myocardial wall motion and thickening from gated technetium-99m sestamibi myocardial perfusion single-photon emission computed tomography. J Am Coll Cardiol. 1997. 30:1360–1367.


12. Grude M, Juergens KU, Wichter T, Paul M, Fallenberg EM, Muller JG, Heindel W, Breithardt G, Fischbach R. Evaluation of global left ventricular myocardial function with electrocardiogram-gated multidetector computed tomography: comparison with magnetic resonance imaging. Invest Radiol. 2003. 38:653–661.


13. Hammer C, Thein E. Determining significant physiologic incompatibilities. Graft. 2001. 4:108–110.


14. Hoffmann U, Moselewski F, Cury RC, Ferencik M, Jang IK, Diaz LJ, Abbara S, Brady TJ, Achenbach S. Predictive value of 16-slice multidetector spiral computed tomography to detect significant obstructive coronary artery disease in patients at high risk for coronary artery disease: patient-versus segment-based analysis. Circulation. 2004. 110:2638–2643.


15. Hosenpud JD, Bennett LE, Keck BM, Fiol B, Boucek MM, Novick RJ. The registry of the International Society for Heart and Lung Transplantation: fifteenth official report-1998. J heart Lung Transplant. 1998. 17:656–668.


16. Juergens KU, Fischbach R. Left ventricular function studied with MDCT. Eur Radiol. 2006. 16:342–357.


17. Juergens KU, Grude M, Maintz D, Fallenberg EM, Wichter T, Heindel W, Fischbach R. Multi-detector row CT of left ventricular function with dedicated analysis software versus MR imaging: initial experience. Radiology. 2004. 230:403–410.


18. Juergens KU, Maintz D, Grude M, Boese JM, Heimes B, Fallenberg EM, Heindel W, Fischbach R. Multi-detector row computed tomography of the heart: does a multi-segment reconstruction algorithm improve left ventricular volume measurements? Eur Radiol. 2005. 15:111–117.


19. Kirkman R. Hardy M, editor. Of swine and man: organ physiology in different species. Xenograft. 1989. vol. 25. Amsterdam: Elsevier;124–136.
20. Knez A, Becker CR, Leber A, Ohnesorge B, Becker A, White C, Haberl R, Reiser MF, Steinbeck G. Usefulness of multislice spiral computed tomography angiography for determination of coronary artery stenoses. Am J Cardiol. 2001. 88:1191–1194.


21. Kopp AF, Schroeder S, Kuettner A, Baumbach A, Georg C, Kuzo R, Heuschmid M, Ohnesorge B, Karsch KR, Claussen CD. Non-invasive coronary angiography with high resolution multidetector-row computed tomography. Results in 102 patients. Eur Heart J. 2002. 23:1714–1725.


22. Lee MY, Lee SH, Lee SG, Park SH, Lee CY, Kim KH, Hwang SH, Lim SY, Ahn YK, Han HJ. Comparative analysis of heart functions in micropigs and conventional pigs using echocardiography and radiography. J Vet Sci. 2007. 8:7–14.
23. Lipton MJ, Higgins CB, Farmer D, Boyd DP. Cardiac imaging with a high-speed Cine-CT Scanner: preliminary results. Radiology. 1984. 152:579–582.
24. Mahnken AH, Spuentrup E, Niethammer M, Buecker A, Boese J, Wildberger JE, Flohr T, Sinha AM, Krombach GA, Gunther RW. Quantitative and qualitative assessment of left ventricular volume with ECG-gated multislice spiral CT: value of different image reconstruction algorithms in comparison to MRI. Acta Radiol. 2003. 44:604–611.


25. Metzgar RS, Seigler HF. Tissue antigens of man and chimpanzees; their role in xenografting. Transplant Proc. 1970. 2:463–467.
26. Mochizuki T, Murase K, Higashino H, Koyama Y, Doi M, Miyagawa M, Nakata S, Shimizu K, Ikezoe J. Two- and three-dimensional CT ventriculography: a new application of helical CT. AJR Am J Roentgenol. 2000. 174:203–208.
27. Nairne P, Allen I, Brazier M, Forrester D, Heap B, Kennedy I. Animal-to-human transplants: the ethics of xenotransplantation. 1996. London: Nuffield Council on Bioethics;1–123.
28. Pattynama PM, Lamb HJ, van der Velde EA, van der Wall EE, de Roos A. Left ventricular measurements with cine and spin-echo MR imaging: a study of reproducibility with variance component analysis. Radiology. 1993. 187:261–268.


29. Qin JX, Jones M, Shiota T, Greenberg NL, Tsujino H, Firstenberg MS, Gupta PC, Zetts AD, Xu Y, Ping Sun J, Cardon LA, Odabashian JA, Flamm SD, White RD, Panza JA, Thomas JD. Validation of real-time three-dimensional echocardiography for quantifying left ventricular volumes in the presence of a left ventricular aneurysm: in vitro and in vivo studies. J Am Coll Cardiol. 2000. 36:900–907.


30. Raff GL, Gallagher MJ, O'Neill WW, Goldstein JA. Diagnostic accuracy of noninvasive coronary angiography using 64-slice spiral computed tomography. J Am Coll Cardiol. 2005. 46:552–557.


31. Ropers D, Baum U, Pohle K, Anders K, Ulzheimer S, Ohnesorge B, Schlundt C, Bautz W, Daniel WG, Achenbach S. Detection of coronary artery stenoses with thin-slice multi-detector row spiral computed tomography and multiplanar reconstruction. Circulation. 2003. 107:664–666.


32. Schoepf UJ, Savino G, Lake DR, Ravenel JG, Costello P. The age of CT pulmonary angiography. J Thorac Imaging. 2005. 20:273–279.
33. Schuijf JD, Bax JJ, Jukema JW, Lamb HJ, Vliegen HW, Salm LP, de Roos A, van der Wall EE. Noninvasive angiography and assessment of left ventricular function using multislice computed tomography in patients with type 2 diabetes. Diabetes Care. 2004. 27:2905–2910.
34. Schuijf JD, Bax JJ, Salm LP, Jukema JW, Lamb HJ, van der Wall EE, de Roos A. Noninvasive coronary imaging and assessment of left ventricular function using 16-slice computed tomography. Am J Cardiol. 2005. 95:571–574.


35. Stankovicova T, Szilard M, De Scheerder I, Sipido KR. M cells and transmural heterogeneity of action potential configuration in myocytes from the left ventricular wall of the pig heart. Cardiovasc Res. 2000. 45:952–960.


36. White HD, Norris RM, Brown MA, Brandt PW, Whitlock RM, Wild CJ. Left ventricular end-systolic volume as the major determinant of survival after recovery from myocardial infarction. Circulation. 1987. 76:44–51.


37. Wilmut I, Schnieke AE, McWhir J, Kind AJ, Campbell KH. Viable offspring derived from fetal and adult mammalian cells. Nature. 1997. 385:810–813.


38. Yamamuro M, Tadamura E, Kubo S, Toyoda H, Nishina T, Ohba M, Hosokawa R, Kimura T, Tamaki N, Komeda M, Kita T, Konishi J. Cardiac functional analysis with multi-detector row CT and segmental reconstruction algorithm: comparison with echocardiography, SPECT, and MR imaging. Radiology. 2005. 234:381–390.