Abstract
Mesenchymal stem cells (MSCs) secrete a variety of neuroregulatory molecules, such as nerve growth factor, brain-derived neurotrophic factor, and glial cell-derived neurotrophic factor, which upregulate tyrosine hydroxylase (TH) gene expression in PC12 cells. Enhancing TH gene expression is a critical step for treatment of Parkinson's disease (PD). The objective of this study was to assess the effects of co-culturing PC12 cells with MSCs from feline bone marrow on TH protein expression. We divided the study into three groups: an MSC group, a PC12 cell group, and the combined MSC + PC12 cell group (the co-culture group). All cells were cultured in DMEM-HG medium supplemented with 10% fetal bovine serum for three days. Thereafter, the cells were examined using western blot analysis and immunocytochemistry. In western blots, the co-culture group demonstrated a stronger signal at 60 kDa than the PC12 cell group (p<0.001). TH was not expressed in the MSC group, either in western blot or immunocytochemistry. Thus, the MSCs of feline bone marrow can up-regulate TH expression in PC12 cells. This implies a new role for MSCs in the neurodegenerative disease process.
Parkinson's disease (PD) is characterized by a loss of dopamine in the striatum resulting from the progressive degeneration of dopaminergic neurons within the substantia nigra [20]. Tyrosine hydroxylase (TH) is the rate-limiting enzyme in the synthesis of catecholamine, and is widely used as a marker of dopaminergic cells [23]. Tang et al. [32] have shown that dopaminergic differentiation of striatum-derived precursor cells following transplantation requires TH induction. Furthermore, Lu et al. [16] observed significant functional recovery in rats model of PD receiving TH-engineered mesenchymal stem cells transplanted into the striatum. Moreover, Peschanski et al. [25] have demonstrated bilateral motor improvement in two patients with PD after grafting of fetal mesencephalic tissue. Therefore, enhancing TH gene expression or protein production is a critical step for treatment of PD.
The PC12 cell line is a neural crest-derived adrenal chromaffin cell line obtained from a rat pheochromocytoma. PC12 cells serve as a useful model system for studying the molecular mechanisms responsible for sympathetic neuronal differentiation induced by nerve growth factor (NGF) because they can synthesize and store the catecholamine neurotransmitters dopamine and norepinephrine [8]. Mesenchymal stem cells (MSCs) derived from bone marrow can differentiate into osteoblasts, chondrocytes, adipocytes [26], and neural cells [15,24]. In addition, MSCs express neuroregulatory molecules, such as NGF, brain-derived neurotrophic factors (BDNF), and glial cell-derived neurotrophic factors (GDNF) [5,6,14,17,18]. NGF can upregulate TH gene expression in PC12 cells [7,13]. Others have reported that induction of TH gene expression can be achieved by co-treatment fetal cerebral cortex with dopamine and BDNF [37,38]. Therefore, novel methods of inducing TH expression via treatment with growth factors, either directly or through cell co-cultures, has significant potential.
Co-cultures of two different cell types provide insight into mechanisms of cell-cell interactions. Direct-contact co-cultures can promote more mature differentiation of neural stem cells [30], which also involves the extracellular matrix or soluble factors secreted by supporting cells [1,30,31]. Moreover, direct contact cultures better mimic intracerebral grafting in terms of the cell-cell interactions.
The cat is the preferred model for studying human neurodegerative disorders [3,19,27,36]. It provides a number of important advantages for biomedical research, particularly stem cell transplantation in fetal and postnatal animals. The purpose of this study was to explore whether MSCs derived from feline bone marrow could enhance the expression of TH in PC12 cells by direct contact co-cultures.
The protocol used in this study was approved by the Gyeongsang National University Animal Use Committee. Feline bone marrow was harvested by bone marrow aspiration from a greater trochanter of the femur. Mononuclear cells were isolated from the collected samples by the Ficoll 400 (Sigma, USA) density gradient method. The cells were then rinsed twice with PBS, and grown in Dulbeco's Modified Eagle high glucose medium (DMEM, 4.5 g/l glucose; Gibco-BRL, USA) with 10% fetal bovine serum (FBS; Gibco-BRL, USA) and penicillin/streptomycin (50 IU/ml / 50 µg/ml; Sigma, USA). After 5 days, the resulting monolayer of cells, hereafter named bone marrow-derived MSCs, was trypsinized and aliquots were frozen and stored or cultivated further.
MSCs were from passage 4. The study was divided into three experimental groups: a MSC group, a PC12 cell group, and the combined MSC + PC12 cell group. The cells were seeded at 1.5 × 105 cells of each cell type (3 × 105 total in the combined group) onto 60 mm dishes and grown in DMEM supplemented with 10% FBS and penicillin/streptomycin (50 IU/ml / 50 µg/ml). Cells were incubated at 37℃ in a humidified atmosphere of 5% CO2 for 3 days.
Ten microliters of CD9 (Serotec, USA), CD18, CD44, and CD45-like (VMRD, USA) cat-specific antibodies were added to the bottom of tubes, followed by 100 µl of 6 × 106 cells/ml single cell suspensions of cultured MSCs in the 4th passage. The mixture was incubated for 30 min at 4℃ in the dark and washed. Five microliters fluorescent-tagged secondary antibodies were then added to the resuspended cells for 30 min at 4℃ in the dark. Positive cells were then detected on flow cytometers.
To identify MSCs from feline derived bone marrow, the primary chicken anti-vimentin antibody (1 : 5,000, polyclonal; Chemicon, USA) was employed; the secondary antibody was a FITC-conjugated antibody against chicken IgG (1 : 320; Sigma, USA). To identify TH-positive differentiation of feline MSCs in co-cultures experiment, MSCs were prelabeled using Dil-Ac-LDL (1 : 20; Biomedical Technologies, USA) and then co-cultured with PC12 for three days. Anti-TH (1 : 500, polyclonal; Chemicon, USA) was the primary antibody used to identify TH in all groups; a FITC-labeled antibody against rabbit IgG (1 : 80; Sigma, USA) was used as the secondary antibody. The cells were cultured in a four-well chamber slide and fixed with 4% paraformaldehyde for 10 min. Following fixation, the cells were treated with 0.3% Triton X-100 containing 10% normal goat serum for 30 min. Cells were then incubated with the primary antibody at room temperature for 2 h, followed by incubation with the FITC-conjugated secondary antibody at 37℃ for 30 min. To prevent non-specific staining, secondary antibodies were used at the same concentrations as the primary antibodies. The slides were examined with an microscope (Olympus, Japan) equipped with fluorescent illumination.
Western blot analysis was performed to determine whether the presence of MSCs affected TH protein expression in PC12 cells. The cells were lysed using a SDS-sample buffer. After ultracentrifugation (12,000 rpm/4℃/10 min), the supernatant was collected. Fifteen µg of protein was separated by SDS-PAGE and transferred to a PVDF membrane (Bio-Rad, USA). Immunoreactions were performed with antibodies against TH (1 : 500, polyclonal; Chemicon, USA) and GAPDH (1 : 300, monoclonal; Chemicon, USA). The membrane was washed and incubated with horseradish peroxidase conjugated anti-rabbit IgG (1 : 1,000; Santa Cruz Biotechnology, USA) or anti-mouse IgG (1 : 5,000; Chemicon, USA). Antigen protein was visualized using chemiluminescence with an ECL-detecting reagent (Amersham Pharmacia Biotech, USA) according to the protocol of the manufacturer.
Experiments were repeated three times. The band from the western blot was scanned and analyzed by densitometry using the Sigma Gel System (SPSS, USA). Density values are expressed as the mean ± SD. Statistical analysis was performed using one-way ANOVA analysis followed by a posthoc LSD test. The acceptance level for statistical significance was p<0.05.
The feline bone marrow-derived mononuclear fraction was isolated and then cultured. Attached cells were observed at 10-14 days after the initial plating. These cells comprised a morphologically heterogeneous population of cells that included spindle-shaped cells, large flat cells, and small round cells (Fig. 1A).
The feline MSC-surface antigen profile was ascertained by staining with feline-specific monoclonal antibodies followed by flow cytometry (Fig. 2). Feline MSCs were strongly positive for CD9 and CD44, but negative for CD18 and CD45. They demonstrated typical characteristics of mesenchymal cells, including the capacity to transform into osteocytes and adipocytes.
In the MSCs alone, the cells showed an elongated fibroblast shape (Fig. 3A). In the PC12 cells alone, the cells showed modest growth and tended to grow in small clusters (Fig. 3B). In the co-cultured group, both cells appeared robust. Although the PC12 cells did not change morphology significantly, the MSCs possessed long, thin, complex processes (Fig. 3C).
Representative immunoblot bands for TH proteins are shown in Fig. 4. TH protein demonstrated a stronger signal at 60 kDa in the co-culture group than the PC12 cell group (p<0.001). In contrast, TH wasn't expressed in the MSC alone group.
MSCs (red) did not show immunostaining of TH protein (green) (Figs. 5A-C), indicating that the MSCs had not differentiated into TH positive cells under our experimental conditions.
Here, we show that MSCs derived from feline bone marrow can enhance TH protein expression in PC12 cells. There are several possibilities for what mediates this induction. First, PC12 cells can synthesize and release dopamine [8], and MSCs can secrete NGF and BDNF [5]. NGF can up-regulate TH mRNA expression in PC12 cells [13], and TH gene expression can be induced in human and murine fetal cerebral cortex by co-treatment with dopamine and BDNF [37,38]. Therefore, the NGF and BDNF produced by MSCs and the dopamine from PC12 cells could stimulate TH induction [13,37,38].
Second, PC12 cells originated from adrenal chromaffin cell-derived tumors [8]. Adrenal medullary chromaffin cells produce FGF-2, TGF-β, GDNF, etc [11,35]. Adult rat bone marrow stromal cells express genes which are associated with dopaminergic neurons [10], and MSCs can differentiate into dopaminergic neurons both in vitro and in vivo [12,33,34]. Here, MSCs did not express TH in western blots or immunostaining, perhaps due to immaturity of the cells at the time of the immunocytochemical study.
Third, studies in both chromaffin and PC12 cells reveal that TH gene expression is dependent on cell density [2,8,28,29]. The increase in TH mRNA at a high cell density is correlated with higher levels of TH immunoreactive protein, increased enzymatic activity, and elevated dopamine content [4,8]. However, TH expression did not vary with increasing PC12 cell density (data not shown), possibly due to the short-term culture conditions. Chromaffin cell differentiation was also not affected by increased cell density [30].
Fourth, direct cell-cell contact may help enhance TH expression. Direct contact between bone marrow stromal cells and the host brain tissue is important for differentiation [1]. Direct chromaffin cell contact may stimulate differentiation of neuronal progenitor cells [30]. The direct interaction of MSCs with neurons promoted long-last survival [31].
In summary, co-culture of MSCs with PC12 cells increases TH expression in the cultures. In vivo, MSCs from bone marrow markedly increase the proliferation of hippocampal-derived neural stem cells via secretion of cytokines [22]. Furthermore, MSCs transplantation into rats following traumatic brain injury improved functional recovery [15]. However, only a small number of transplanted cells expressed neuronal antigens in that study, making functional improvement in recipients unlikely. Therefore, the secretion of neurotrophic factors or direct cell-cell contact by MSCs may be more important than the resulting neural differentiation in transplant experiments. Our results imply a new role for MSCs in treating neurodegenerative diseases such as PD.
Figures and Tables
Fig. 1
Identification of isolated bone marrow-derived MSCs. (A) Phase contrast image of heterogeneous MSCs after passage 1. (B) Phase contrast image of fibroblast-like, homogeneous MSCs after passage 4. (C) Immunostaining of vimentin antigen, a marker expressed by BMCs. Scale bar = 200 µm, A and B; 50 µm, C.
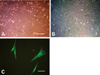
Fig. 2
Flow cytometric determination of cell-surface antigen profiles for feline MSC. Feline bone marrow MSCs were stained with feline specific antibodies to a variety of cell-surface antigens. The percentage of cells positive for each antibody is listed in the corresponding histogram. An isotype control is used to identify background fluorescence. MSC from 3 separate cats were tested with similar results. Representative results from 1 cat are shown.
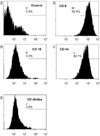
Fig. 3
Phase contrast image of PC12 cells and MSCs. (A) MSCs cultured alone for 3 days. The cells exhibited an elongated spindle shape resembling fibroblasts. (B) PC12 cells cultured alone for 3 days. The cells tended to grow in small clusters. (C) PC12 cells were co-cultured with MSCs for 3 days. The PC12 cells were not significantly changed, but the MSCs possessed a longer, more complex processes (white arrowheads). Scale bar = 200 µm.
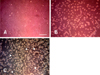
Fig. 4
Representative Western blot analysis of TH proteins. (A) Immunoblots of TH and GAPDH protein expression. M, molecular marker, Lane 1, MSC group; Lane 2, PC12 group; Lane 3, MSC + PC12 group. (B) Ratio of TH to GAPDH density values in A. TH expression was significantly increased in the MSC + PC12 group. **p<0.001 vs. PC12 group.
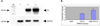
Acknowledgments
This work was supported by funds from KOSEF (Grant #M10525010001-05N2501-00110) and Post BK21 for G.Z. Jin and S.J. Cho. We deeply thank Dr. Tae-Hoon Lee (Department of Stomatology, Chonnam National University, Korea) for the kind donation of PC12 cells.
References
1. Abouelfetouh A, Kondoh T, Ehara K, Kohmura E. Morphological differentiation of bone marrow stromal cells into neuron-like cells after co-culture with hippocampal slice. Brain Res. 2004. 1029:114–119.


2. Acheson AL, Thoenen H. Cell contact-mediated regulation of tyrosine hydroxylase synthesis in cultured bovine adrenal chromaffin cells. J Cell Biol. 1983. 97:925–928.


3. Arts MP, Cools AR. 6-hydroxydopamine lesion in the A8 cell group of cats produces a short-lasting decreased accuracy in goal-directed forepaw-movements. Behav Brain Res. 1999. 103:13–21.


4. Badoyannis HC, Sharma SC, Sabban EL. The differential effects of cell density and NGF on the expression of tyrosine hydroxylase and dopamine beta-hydroxylase in PC12 cells. Brain Res Mol Brain Res. 1991. 11:79–87.


5. Crigler L, Robey RC, Asawachaicharn A, Gaupp D, Phinney DG. Human mesenchymal stem cell subpopulations express a variety of neuro-regulatory molecules and promote neuronal cell survival and neuritogenesis. Exp Neurol. 2006. 198:54–64.


6. Garcia R, Aguiar J, Alberti E, de la Cuetara K, Pavon N. Bone marrow stromal cells produce nerve growth factor and glial cell line-derived neurotrophic factors. Biochem Biophys Res Commun. 2004. 316:753–754.


7. Gizang-Ginsberg E, Ziff EB. Nerve growth factor regulates tyrosine hydroxylase gene transcription through a nucleoprotein complex that contains c-Fos. Genes Dev. 1990. 4:477–491.


8. Greene LA, Tischler AS. Establishment of a noradrenergic clonal line of rat adrenal pheochromocytoma cells which respond to nerve growth factor. Proc Natl Acad Sci USA. 1976. 73:2424–2428.


9. Guarita-Souza LC, Carvalho KA, Rebelatto C, Senegaglia A, Hansen P, Furuta M, Miyague N, Francisco JC, Olandoski M, Faria-Neto JR, Oliveira SA, Brofman PR. Cell transplantation: differential effects of myoblasts and mesenchymal stem cells. Int J Cardiol. 2006. 111:423–429.


10. Kramer BC, Woodbury D, Black IB. Adult rat bone marrow stromal cells express genes associated with dopamine neurons. Biochem Biophys Res Commun. 2006. 343:1045–1052.


11. Krieglstein K, Henheik P, Farkas L, Jaszai J, Galter D, Krohn K, Unsicker K. Glial cell line-derived neurotrophic factor requires transforming growth factor-beta for exerting its full neurotrophic potential on peripheral and CNS neurons. J Neurosci. 1998. 18:9822–9834.


12. Li Y, Chen J, Wang L, Zhang L, Lu M, Chopp M. Intracerebral transplantation of bone marrow stromal cells in a 1-methyl-4-phenyl-1,2,3,6-tetrahydropyridine mouse model of Parkinson's disease. Neurosci Lett. 2001. 316:67–70.


13. Li XM, Qi J, Juorio AV, Boulton AA. Reciprocal regulation of the content of aromatic L-amino acid decarboxylase and tyrosine hydroxylase mRNA by NGF in PC12 cells. J Neurosci Res. 1997. 47:449–454.


14. Liu LH, Sun Z, Sun QY, Huang YJ, Man QH, Guo M, Zhao CH, Ai HS. Study on biological characteristics of cultured rhesus mesenchymal stem cells. Zhongguo Shi Yan Xue Ye Xue Za Zhi. 2005. 13:417–421.
15. Lu J, Moochhala S, Moore XL, Ng KC, Tan MH, Lee LK, He B, Wong MC, Ling EA. Adult bone marrow cells differentiate into neural phenotypes and improve functional recovery in rats following traumatic brain injury. Neurosci Lett. 2006. 398:12–17.


16. Lu L, Zhao C, Liu Y, Sun X, Duan C, Ji M, Zhao H, Xu Q, Yang H. Therapeutic benefit of TH-engineered mesenchymal stem cells for Parkinson's disease. Brain Res Brain Res Protoc. 2005. 15:46–51.


17. Mahmood A, Lu D, Chopp M. Intravenous administration of marrow stromal cells (MSCs) increases the expression of growth factors in rat brain after traumatic brain injury. J Neurotrauma. 2004. 21:33–39.


18. Majumdar MK, Thiede MA, Mosca JD, Moorman M, Gerson SL. Phenotypic and functional comparison of cultures of marrow-derived mesenchymal stem cells (MSCs) and stromal cells. J Cell Physiol. 1998. 176:57–66.


21. Martin DR, Cox NR, Hathcock TL, Niemeyer GP, Baker HJ. Isolation and characterization of multipotential mesenchymal stem cells from feline bone marrow. Exp Hematol. 2002. 30:879–886.


22. Munoz JR, Stoutenger BR, Robinson AP, Spees JL, Prockop DJ. Human stem/progenitor cells from bone marrow promote neurogenesis of endogenous neural stem cells in the hippocampus of mice. Proc Natl Acad Sci USA. 2005. 102:18171–18176.


23. O'Byrne MB, Bolam JP, Hanley JJ, Tipton KF. Tyrosine-hydroxylase immunoreactive cells in the rat striatum following treatment with MPP+. Adv Exp Med Biol. 2000. 483:369–374.
24. Park KS, Lee YS, Kang KS. In vitro neuronal and osteogenic differentiation of mesenchymal stem cells from human umbilical cord blood. J Vet Sci. 2006. 7:343–348.


25. Peschanski M, Defer G, N'Guyen JP, Ricolfi F, Monfort JC, Remy P, Geny C, Samson Y, Hantraye P, Jeny R, et al. Bilateral motor improvement and alteration of L-dopa effect in two patients with Parkinson's disease following intrastriatal transplantation of foetal ventral mesencephalon. Brain. 1994. 117:487–499.


26. Pittenger MF, Mackay AM, Beck SC, Jaiswal RK, Douglas R, Mosca JD, Moorman MA, Simonetti DW, Craig S, Marshak DR. Multilineage potential of adult human mesenchymal stem cells. Science. 1999. 284:143–147.


27. Rohn JL, Gwynn SR, Lauring AS, Linenberger ML, Overbaugh J. Viral genetic variation, AIDS, and the multistep nature of carcinogenesis: the feline leukemia virus model. Leukemia. 1996. 10:1867–1869.
28. Saadat S, Stehle AD, Lamouroux A, Mallet J, Thoenen H. Influence of cell-cell contact on levels of tyrosine hydroxylase in cultured bovine adrenal chromaffin cells. J Biol Chem. 1987. 262:13007–13014.


29. Saadat S, Thoenen H. Regulation of tyrosine hydroxylase mRNA levels in rat pheochromocytoma PC12 cells by cell-cell contact. Exp Cell Res. 1988. 176:187–193.


30. Schumm MA, Castellanos DA, Frydel BR, Sagen J. Enhanced viability and neuronal differentiation of neural progenitors by chromaffin cell co-culture. Brain Res Dev Brain Res. 2002. 137:115–125.


31. Scuteri A, Cassetti A, Tredici G. Adult mesenchymal stem cells rescue dorsal root ganglia neurons from dying. Brain Res. 2006. 1116:75–81.


32. Tang Z, Yu Y, Guo H, Zhou J. Induction of tyrosine hydroxylase expression in rat fetal striatal precursor cells following transplantation. Neurosci Lett. 2002. 324:13–16.


33. Tao H, Rao R, Ma DD. Cytokine-induced stable neuronal differentiation of human bone marrow mesenchymal stem cells in a serum/feeder cell-free condition. Dev Growth Differ. 2005. 47:423–433.


34. Tatard VM, D'Ippolito G, Diabira S, Valeyev A, Hackman J, McCarthy M, Bouckenooghe T, Menei P, Montero-Menei CN, Schiller PC. Neurotrophin-directed differentiation of human adult marrow stromal cells to dopaminergic-like neurons. Bone. 2007. 40:360–373.


35. Unsicker K, Krieglstein K. Growth factors in chromaffin cells. Prog Neurobiol. 1996. 48:307–324.


36. Wade TV, Rothblat DS, Schneider JS. Changes in striatal dopamine D3 receptor regulation during expression of and recovery from MPTP-induced parkinsonism. Brain Res. 2001. 905:111–119.

