Abstract
The aim of the present study was to assess the clinical and histopathological findings in a canine model of ischemic stroke. Cerebral ischemic stroke was induced by middle cerebral artery occlusion in four healthy beagle dogs using silicone plugs. They showed neurological signs of forebrain dysfunction such as reduced responsiveness, head turning, circling, postural reaction deficits, perceptual deficits, and hemianopsia. These signs gradually regressed within 4 weeks without therapy. On magnetic resonance imaging, T2 hyperintensity and T1 hypointensity were found in the cerebral cortex and basal ganglia. These lesions were well-defined and sharply demarcated from adjacent brain parenchyma with a homogenous appearance. No abnormalities of the cerebrospinal fluid were observed. At necropsy, atrophic and necrotic lesions were observed in the cerebral cortex. The cerebral cortex, basal ganglia, and thalamus were partially unstained with triphenyl-tetrazolium chloride. Histopathologically, typical features of infarction were identified in cortical and thalamic lesions. This study demonstrates that our canine model resembles the conditions of real stroke patients.
Strokes have been reported rarely in dogs and cats [1,6,7,11,13,16,21]. They are likely underdiagnosed because of a lack of clinical suspicion, unavailability of magnetic resonance imaging (MRI) or computed tomography (CT), and the wide array of presenting clinical signs [9]. However, strokes are now being recognized with greater frequency in dogs due to the increased availability of MRI [13]. Until recently, most reports of strokes in dogs have been limited to postmortem investigations of dogs that died or were euthanized due to the severity of their brain infarct or the suspected underlying cause for infarction [6]. Thus, the true incidence and prognosis of strokes in dogs are unknown. There is no proven preventive or treatment for stroke in dogs [10].
Even though suspected cases of stroke in dogs can now be studied antemortem, necropsy must be performed for definitive diagnosis. It is also difficult to find effective diagnostic or therapeutic methods for stroke in clinical patients during short periods because of presentation related to time of onset, the limited number of cases, and restriction of continuous examination and monitoring after the improvement of neurological signs. In human medicine, to overcome these difficulties, many animal models of stroke have been created using various techniques [23]; among these, some canine models have been developed [4,8,12,14,15,18-20,24].
Four healthy mature beagle dogs (all males, 3-4 years old, weighing 10 to 15 kg), which had been reared in a farm for 6 months after importation from a commercial laboratory animal company (Harlan Interfauna, UK), were studied. Prior to arrival in our facility, all dogs were tested for canine distemper virus by reverse transcription polymerase chain reaction (RT-PCR), and for Toxoplasma and Neospora by IgG antibody titer test, and were only accepted after testing seronegative. They were screened for metabolic diseases by complete blood count and serum chemistry analysis, and for external and internal parasites related to neurological diseases (ticks and Dirofilaria immitis). After arrival, they were adapted and assessed daily for neurological or behavioral abnormalities and general health status for 2 weeks. Each dog was housed in a single cage and fed twice a day with commercial dry food at a well-ventilated facility. The surgical procedures and the experimental protocol were approved by the Institutional Animal Care and Use Committee (Konkuk University, Korea). The approved study endpoint was 4 months following middle cerebral artery occlusion (MCAO). All dogs were euthanized at this point. Criteria for early euthanization included: serious neurological or clinical compromise and inability of the animal to care for itself, inability to self-feed after the initial recovery period, and inactivity and lack of alertness for a continuous 24-h period.
Dogs were restricted for 12 h prior to the induction of anesthesia. They were premedicated with atropine (Je-Il Pharm, Korea) (0.02 mg/kg body weight, subcutaneously [SC]) and acepromazine (Samu Median, Korea) (0.2 mg/kg body weight, intramuscularly [IM]), and were then anesthetized 30 min later using propofol (Hana Pharm, Korea) (5 mg/kg body weight, intravenously [IV]), orally intubated, and mechanically ventilated. Anesthesia was maintained with isoflurane (Minrad, USA) at 2 to 3% inspired volume during surgery. The tidal volume and ventilatory frequency were adjusted to maintain a partial pressure of arterial oxygen (PaO2) of 150 ± 50 mm Hg and a partial pressure of arterial carbon dioxide (PaCO2) of 40 ± 5 mmHg. Blood gases, glucose, and hematocrit were measured before, during, and after MCAO. The fluid balance was maintained by intravenous administration of 0.9% sodium chloride (Dai Han Pharm, Korea). Rectal temperature was monitored continuously and maintained at 36-38℃ throughout the surgery.
The embolus was made as described previously [15,19], with some modifications. In brief, a silk suture (4-0) (B.Braun Medical Industries, Malaysia) was passed into the tip of a 20-gauge venous catheter (Becton Dickinson Korea, Korea), lopped at the hub, and passed back out of the tip. Using a 3-ml syringe, silicone rubber (Dow Corning, USA) with catalyst was injected into the hub of the suture-containing catheter and cured for 24 h. After curing, the catheter was dissected from the silicone-attached suture. The embolus was made by cutting the cured silicone to a length of 7 mm. It was inserted into the tip of an 18-gauge venous catheter; the suture was passed out of the hub and then coiled in a 25-ml syringe. The syringe was connected to the embolus-containing catheter, and was then filled with 20 ml of physiological saline. The plunger was then placed in the syringe.
Cerebral ischemia was induced by MCAO as described previously [15,19], with some modifications. Animals were positioned in right lateral recumbency. Hair from the neck area that was to be surgically exposed was shaved, and the skin was thoroughly prepared with povidone-iodine and alcohol scrub. A strict, sterile surgical technique was utilized in all cases. A cervical incision was made to expose carotid arteries. By using blunt dissection and palpating the carotid pulse, the carotid sheath was exteriorized at the level of bifurcation under the sternomastoideus muscle. The common carotid artery was separated from the vagosympathetic trunk. The internal and external carotid arteries were identified. The common carotid artery was temporarily elevated using umbilical tape. A 16-gauge venous catheter was directly inserted into the left internal carotid artery through the carotid bulb. The 18-gauge catheter/25-ml syringe loaded with an embolus was inserted through the 16-gauge catheter. The embolus was flushed into the internal carotid artery and up to the origin of the middle cerebral artery (MCA) by applying moderate force to the syringe plunger. The saline was injected at a total volume of 20 ml, at a rate of 2 ml per second. Delivery of the embolus was confirmed by arterial back-flow in the syringe. The traction on the common carotid artery was removed, and the catheters were also removed. The neck incision was then sutured, exposing the remaining suture of the neck. All dogs were permanently occluded until they were euthanized.
After surgery, the dogs were woken up, extubated, and then returned to the cages in the animal recovery room. Butorphanol (Myungmoon Pharm, Korea) (0.4 mg/kg body weight, IM) and ampicillin (Unibiotech, Korea) (20 mg/kg body weight, IV) were administered to the dogs for 1 week to control pain and bacterial infection. A floor heating lamp was placed in front of each cage, and the radiant heat was directed to one side of the cage (not directly at the animal). Animals were observed continuously until they had fully recovered, for about 4 h in total. The next day, they were transported to the holding area and periodically observed until euthanasia. The incision was cleaned daily with chlorohexidine flush solution and bandaged for 1 week. Diuretic therapy with mannitol (Daehan Pharm, Korea) (1 g/kg body weight, constant rate of IV infusion for 30 min) was also continued for the next 1-3 days post-stroke as necessary.
Neurobehavioral scoring was performed using a standardized categorical rating scale as described previously [2,18]; scoring was performed for motor function (no deficit = 1, hemiparetic but able to walk = 2, stands only with assistance = 3, hemiplegic and unable to stand = 4, comatose or dead, not testable = 4), consciousness (normal = 1, mildly reduced = 2, severely reduced = 3, comatose or dead = 4), head turning (absent = 0, posturing and turns toward side of infarct = 1, unable to lift head, comatose, or dead = 1), circling (absent = 0, present = 1, does not walk or dead = 1), and hemianopsia (absent = 0, present = 1, unable to test because of reduced consciousness or death = 1). According to this scoring, a completely normal dog would have a total score of 2, and a dog with the most severe deficits (comatose or dead) would have a total score of 11. Each dog was assessed prior to anesthesia, daily during the first week, and then weekly until euthanasia.
Scans were performed 2 days after occlusion. All dogs were fasted the night before the procedures as a precaution for anesthesia. They were pre-medicated and anesthetized using the same procedure, described in animal preparation and monitoring. Anesthesia was adjusted to maintain immobility during the scan. The scanning was performed in a 3.0 Tesla research scanner instrument (Impedance Imaging Research Center, Kyung-Hee University, Korea). The MR coil was placed around the head, and the dog was placed in the magnet for MRI to identify infracted regions. T1- and T2-weighted images (WI) were obtained in sagittal, transversal, and dorsal planes.
The ischemic lesion area was calculated from T2-WI using imaging software (MRIcro, Version 1.40; Chris Rorden, USA). For each slice, the higher intensity lesions in T2-WI when the signal intensity was 1.25 times higher than that of the counterpart in the contra-lateral brain lesion were marked as the ischemic lesion areas. The lesion volume was presented as a volume percentage of the lesion compared with the contralateral hemisphere.
CSF was directly obtained from each dog after MR scanning. It was collected by puncture with a 20-gauge sterile, disposable spinal needle (Hakko, Japan) of the cerebellomedullary cistern in lateral recumbency. The inclusion criterion for the study was no iatrogenic blood contamination during CSF collection. A total of 2-3 ml of CSF was collected into a plain, sterile tube without anticoagulant. It was used for routine diagnostic evaluation; the erythrocyte and nucleated cell count was determined using a standard hematocytometer chamber, cytocentrifuge cytology, and estimation of the total protein concentration using a urine dipstick (Young-Dong, Korea). These analyses were performed within 30 min after collection.
Four months after surgery, all dogs were euthanized with sodium pentobarbital (Hanrim Pharm, Korea) (80 mg/kg body weight, IV). The brains were carefully removed and dissected into coronal 2-mm sections. The fresh brain slices were immersed in a 2% solution of 2,3,5-triphenyl-tetrazolium chloride (TTC) in normal saline at 37℃ for 30 min.
Brain slices were placed in 10% paraformaldehyde in phosphate buffer. After at least 72 h of immersion fixation, the slices dehydrated and embedded in paraffin. Transverse sections (5 µm) were cut, stained with hematoxylin and eosin, and examined by light microscopy for histopathologic alterations associated with ischemic stroke.
In all dogs, anesthesia and physiological parameters were well maintained throughout the surgery. Infection in or around the incision site and pain and discomfort were successfully treated with butorphanol. There were no significant differences in the physiological parameters before, during, and after MCAO. The mean and standard deviation of each parameter are summarized in Table 1.
All embolized dogs were slow to awaken. No dogs met the criteria for early euthanization. One dog (identification number (ID) 1) showed neurological signs of forebrain dysfunction, such as reduced responsiveness, head turning, and circling. The other three dogs commonly showed other signs, such as walking into walls, postural reaction deficits, perceptual deficits (menance response and facial sensation), and hemianopsia, as well as the abnormalities observed in ID 1. These signs gradually improved within 4 weeks without therapy. However, hemianopsia was not resolved prior to euthanasia. The neurobehavioral scores of each dog are summarized in Table 2.
MRI scanning was performed in all dogs. Increased signal intensity on T2-weighted images and decreased signal intensity on T1-weighted images were commonly found in basal ganglia (all dogs), the left ventral cortex (ID 1), the left ventrolateral cortex (ID 3 and 4), and almost the entire left cortex of the cerebrum (ID 2). These lesions were well-defined and sharply demarcated from adjacent brain parenchyma with a homogenous appearance, and were confined to gray matter. However, occasional white matter involvement was found in 3 dogs (ID 2-4) due to severe gray matter changes. Swelling and a midline shift of the brain caused by mass effects were identified in the same dogs (Fig. 1). No other lesions were found in the thalamus, brain stem, or cerebellum.
On the estimation of the percent lesion volume, ID 2 had the largest volume (67.8%). The other three dogs had similar lesion volumes at 16.9% (ID 1), 23.8% (ID 3), and 20% (ID 4).
In all of the samples obtained, no erythrocytes were found and nucleated cells numbered less than 5 cells/µl. On cytological examination, mononuclear cells were uncommonly observed without pleocytosis. CSF protein concentrations were found to be less than 30 mg/dl by estimation with a urine dipstick.
Atrophic and necrotic lesions were observed on the ventral surface of the left cerebral cortex (ID 1) and the lateral surface of the left cerebral cortex (ID 2-4) (Fig. 2). In these three dogs, lesions that were not stained with TTC were commonly found in the basal ganglia, lateral cortex, and thalamus (Fig. 3).
Microscopic examinations were performed in all dogs. Hallmarks of infarction such as neuronal cell body shrinkage, pyknosis of nuclei, eosinophilia of the cytoplasm, and neuronal loss were observed in thalamic lesions of experimental dogs (Fig. 4A). In cortical lesions, loss of tissue cohesion, infiltration by leukocytes, neuronal loss, marked angioblastic proliferation, and congestion of small parenchymal blood vessels were identified (Fig. 4B).
We have successfully implemented a canine model of permanent embolic stroke. Models of cerebral ischemia can be created in various animals. There are advantages and disadvantages of utilizing animals of different sizes to study cerebral ischemic stroke [24]. Small animals (e.g., mice, rats, gerbils) are often more cost-effective, and allow for relatively simpler genetic modification and management. However, they have lissencephalic brains, and thus may be quite different in terms of anatomy and functional aspects compared to the human brain. On the other hand, large animals (e.g., cats, dogs, pigs, sheep, and monkeys) have gyrencephalic brains, which are structurally and functionally similar to the human brain [23]. Even though primates would be the best model for human ischemic stroke among large animals, both ethical and economic issues limit the widespread use of primates [18]. Thus, we selected the dog as an experimental animal for the study of ischemic stroke because it is readily and economically available, easy to care for, and have predictable intercurrent diseases.
Experimental cerebral ischemia can be global, hemispheric, multifocal, or focal. The focal or regional cerebral ischemic model was chosen because, in clinical practice, those patients affected by an ischemic insult of this nature potentially have the greatest capacity for recovery [4]. Until now, two major techniques have been used for the production of experimental models of focal cerebral ischemia: 1) extravascular arterial occlusion by clips, ligatures, or elecrocautery; and 2) intravascular occlusion of one major cerebral blood vessel, such as the MCA, by the intra-arterial injection of various embolic materials [12]. Because secondary problems such as transoperative hypoxia, meningitis, intra-temporal hematoma, subarachnoid hemorrhage, intracranial infection, and hydrocephalus resulted from mechanical manipulation and violation of the dura, an extravascular arterial occlusion model requiring intracranial surgery is likely to be suboptimal [18]. MCAO models do not require craniotomy, and have been used extensively because of their purported relevance to human thromboembolic stroke [23]. Even though the major causes of canine infarction are not exactly known, thromboembolic stroke may be typical in dogs [5]. Thus, we created a canine cerebral infarction model by MCAO and used a silicone plug as an embolus.
In previous studies [15,19], an embolus was inserted into the internal carotid artery through an incision. We injected a silicone plug through a catheterized vessel instead of an incision, and thus, the process of insertion and the control of hemorrhaging could be easily performed without suturing. We also injected a larger volume (8 to 13 ml) of saline than in previous studies [15,19]. Using these modified procedures, we successfully lodged an embolus into the MCA in all dogs. Even though the number of dogs examined in our study was small, our model showed continuous reproducibility. In previous studies, an embolus was inserted into the MCA in 70% [15] and 78% [19] of examined dogs, respectively. Because those studies used several dogs (55 [15] and 19 dogs [19]), results obtained from greater numbers of experimental dogs will be needed to verify the reproducibility of this model.
In this study, significant differences of physiological parameters were not found before, during, or after the surgery. Some other studies using rats [25] and macaques [3] also showed no differences following occlusion. Thus, these variables may not be closely related to canine ischemic stroke. However, other parameters, such as mean arterial blood pressure, cerebral blood flow, hemoglobin concentration, and blood gases, should be studied in the future because they have not been well-studied and their relationship with stroke in canine patients or the experimental model is not yet known.
MCAO can be maintained transiently or permanently according to the purpose of study. Even though reperfusion was not allowed during the 4 months of the study, all dogs survived and improved over time. Because the vertebral arteries of dogs assume a greater importance in terms of the total blood supply to the brain [5], it may be possible to protect the brain against the effects of cerebral arterial occlusion during such long periods. Thus, longer periods of occlusion may be needed to create sufficient ischemic lesions and allow for continuous reproducibility in transient and permanent occlusion canine models than in other animals. Because the end of an inserted suture is exposed through the incision site in our model, reperfusion can be easily performed at any time without thrombolytic therapy; this is necessary in a thromboembolic model using autologous clots. The optimal time of reperfusion in a transient canine model should be investigated through further study.
In canine stroke, clinical signs are typically characterized as peracute or acute at onset, focal, and nonprogressive [22]. With forebrain lesions, the clinical signs may vary from simple disorientation to death. A unilateral lesion will induce ipsilateral circling and head turning, hemi-inattention syndrome, contralateral central blindness, contralateral ataxia, and proprioception deficits [17]. Most dogs with ischemic stroke tend to recover within several weeks with only supportive care [5].
In this study, mental alteration, ipsilateral circling, and head turning were commonly observed in all dogs. All signs were acute after awakening from anesthesia, and improved within 4 weeks. Seizures were not observed in any of the dogs. In veterinary medicine, seizures have been reported, but are considered an uncommon manifestation of focal ischemic stroke in dogs [9]. Three dogs (ID 2-4) with large lesions in the cerebral cortex showed contralateral proprioceptive deficits and permanent hemianopsia, as well as the commonly observed signs mentioned above. To improve these signs, more time (1 to 3 weeks) was needed compared to the other dog (ID 1). Hemianopsia and motor dysfunction may not have been observed in ID 1 because it had a relatively small lesion on the occipital lobe related to vision and the cerebrum. Even though ID 3 had the second largest lesion, the reduced level of consciousness that was commonly shown in other dogs was not observed. Based on these findings, the prognosis of the canine model of ischemic stroke may be closely related to the initial severity of the neurological deficits and the presence of secondary pathological effects (extracellular edema and increased intracranial pressure).
MRI is the most sensitive imaging modality for diagnosing ischemic stroke [5]. CT is also used in the diagnosis of stroke, but is inferior to MRI in detecting ischemic infarction because of beam-hardening artifacts, inferior contrast display, and inability to provide detailed multiplanar views [10]. Because dogs have larger brains than other common animal models, they are more amenable to study with imaging modalities [18]. The MRI findings in ischemic stroke result from the accumulation of water due to cytotoxic and vasogenic edema [10]. Thus, the typical MRI features of ischemic stroke include T2 hyperintensity and T1 hypointensity, and these were well-observed in this study. Even though the thalamus is vulnerable to MCAO and unstained lesions were clearly observed on TTC staining, no abnormalities were found on MRI. MRI was only performed at 2 days after the occlusion, and more time may be needed to find thalamus lesions than the basal ganglia and cerebral cortex. The detection of ischemic tissue in the peracute and early subacute stage is difficult; thus, other techniques (e.g., diffusion-weighted imaging, perfusion-weighted imaging, magnetic resonance angiography, magnetic resonance spectroscopy, and fluid-attenuated inversion recovery imaging) can be applied [6,7,10]. Because the features of these techniques are not well known in dogs, the canine model can be useful for imaging as well as therapeutic study in veterinary medicine.
CSF analysis is variable in dogs with ischemic stroke. In most cases, the CSF is either normal or reflects a mild mononuclear or neutrophilic pleocytosis [16]. No CSF abnormalities were observed in this study.
MCA has tree-like branches that bring blood to the entire lateral cortex of each hemisphere. The central branches of the MCA are the medial and lateral striata arteries. The striata supply the basal ganglia, internal capsule, and thalamus with blood [5]. The areas of the brain that are vulnerable to ischemic stroke include the hippocampus, cerebral cortex, cerebellum, thalamus, and basal ganglia [16]. In this study, ischemic lesions could be identified in the cerebral cortex, thalamus, and basal ganglia using MRI, necropsy, and TTC staining. These lesions were confirmed by histopathologic examinations.
In conclusion, we have developed a canine model of ischemic stroke that has a resemblance to real stroke patients. However, additional studies are needed to verify the reproducibility and clinical time courses of this model. Until recently, most therapeutic (neuroprotective and neuroregenerative), diagnostic, and preventive studies for ischemic stroke have been performed using small animals. If verified canine models are used in these fields, more effective, promising, and reliable results can be obtained and applied to human and veterinary medicine.
Figures and Tables
Fig. 1
Transverse (A and B) and dorsal (C and D) T1-weighted and T2-weighted MR images of the brain in an experimentally embolized dog (ID 2). Hypointense (A and C) and hyperintense (B and D) lesions were found in lateral cortex (arrows) and caudate nucleus (arrow heads). In T2-weighted image, the well defined lesion was sharply demarcated from adjacent brain parenchyma with a homogenous appearance. Swelling, midline shift, and suppressed left lateral ventricle and thalamus by mass effect were identified in all images.
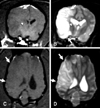
Fig. 2
The ventral (A) and lateral (B) surface of the brain (ID 2) 4 months after MCAO by a silicone embolus exhibits remarkable atrophy and necrosis (arrows) in the affected lateral cortex.
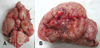
Fig. 3
Coronal section of the brain (ID 2) after TTC staining demonstrate unstained lesion on thalamus (arrow) and atrophic changes (arrow heads) on the left lateral cortex.
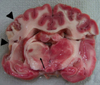
Fig. 4
Microscopic features of the brain in an experimentally embolized dog (ID 2). (A) Thalamic lesion. Necrotic neurons (arrows), nuclear pyknosis, eosinophilia of the cytoplasm, and karyolysis were prominent. H&E stain, ×400. (B) Cortex lesion. Loss of tissue cohesion, infiltration by leukocytes (especially polymorphonuclear leukocytes), congestion of small parenchymal blood vessels (arrow heads), and angioblastic proliferation were observed. H&E stain, ×100.
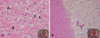
Acknowledgments
This work was supported by the SRC/ERC Program (R11-2002-103) and a Grant (M103KV010026-07K2201-02610) from the Brain Research Center of the 21st Century Frontier Research Program funded by the Ministry of Science and Technology (MOST), Korea.
References
1. Berg JM, Joseph RJ. Cerebellar infarcts in two dogs diagnosed with magnetic resonance imaging. J Am Anim Hosp Assoc. 2003. 39:203–207.


2. Corbett RJ, Purdy PD, Laptook AR, Chaney C, Garcia D. Noninvasive measurement of brain temperature after stroke. AJNR Am J Neuroradiol. 1999. 20:1851–1857.
3. D'Arceuil HE, Duggan M, He J, Pryor J, de Crespigny A. Middle cerebral artery occlusion in Macaca fascicularis: acute and chronic stroke evolution. J Med Primatol. 2006. 35:78–86.
4. Diaz FG, Mastri AR, Ausman JI, Chou SN. Acute cerebral revascularization: Part I. Cerebral ischemia experimental animal model. Surg Neurol. 1979. 12:353–362.
5. Garosi LS, McConnell JF. Ischaemic stroke in dogs and humans: a comparative review. J Small Anim Pract. 2005. 46:521–529.


6. Garosi LS, McConnell JF, Platt SR, Barone G, Baron JC, de Lahunta A, Schatzberg SJ. Results of diagnostic investigations and long-term outcome of 33 dogs with brain infarction (2000-2004). J Vet Intern Med. 2005. 19:725–731.


7. Garosi LS, McConnell JF, Platt SR, Barone G, Baron JC, de Lahunta A, Schatzberg SJ. Clinical and topographic magnetic resonance characteristics of suspected brain infarction in 40 dogs. J Vet Intern Med. 2006. 20:311–321.


8. Guo J, Liao JJ, Preston JK, Batjer HH. A canine model of acute hindbrain ischemia and reperfusion. Neurosurgery. 1995. 36:986–992.


9. Hillock SM, Dewey CW, Stefanacci JD, Fondacaro JV. Vascular encephalopathies in dogs: incidence, risk factors, pathophysiology, and clinical signs. Compend Contin Educ Pract Vet. 2006. 28:196–207.
10. Hillock SM, Dewey CW, Stefanacci JD, Fondacaro JV. Vascular encephalopathies in dogs: diagnosis, treatment, and prognosis. Compend Contin Educ Pract Vet. 2006. 28:208–217.
11. Joseph RJ, Greenlee PG, Carrillo JM, Kay WJ. Canine cerebrovascular disease: clinical and pathological findings in 17 cases. J Am Anim Hosp Assoc. 1988. 24:569–576.
12. Kuwabara S, Uno J, Ishikawa S. A new model of brainstem ischemia in dogs. Stroke. 1988. 19:365–371.


13. McConnell JF, Garosi L, Platt SR. Magnetic resonance imaging findings of presumed cerebellar cerebrovascular accident in twelve dogs. Vet Radiol Ultrasound. 2005. 46:1–10.


14. Molinari GF. Experimental cerebral infarction. I. Selective segmental occlusion of intracranial arteries in the dog. Stroke. 1970. 1:224–231.


15. Okada Y, Shima T, Yokoyama N, Uozumi T. Comparison of middle cerebral artery trunk occlusion by silicone cylinder embolization and by trapping. J Neurosurg. 1983. 58:492–499.


16. Panarello GL, Dewey CW, Barone G, Stefanacci JD. Magnetic resonance imaging of two suspected cases of global brain ischemia. J Vet Emerg Crit Car. 2004. 14:269–277.


17. Platt SR, Garosi L. Canine cerebrovascular disease: Do dogs have strokes? J Am Anim Hosp Assoc. 2003. 39:337–342.


18. Purdy PD, Devous MD, Batjer HH, White CL, Meyer Y, Samson DS. Microfibrillar collagen model of canine cerebral infarction. Stroke. 1989. 20:1361–1367.


19. Purdy PD, Devous MD, White CL, Batjer HH, Samson DS, Brewer K, Hodges K. Reversible middle cerebral artery embolization in dogs without intracranial surgery. Stroke. 1989. 20:1368–1376.


20. Qureshi AI, Boulos AS, Hanel RA, Suri MFK, Yahia AM, Alberico RA, Hopkins LN. Randomized comparison of intra-arterial and intravenous thrombolysis in a canine model of acute basilar artery thrombosis. Neuroradiology. 2004. 46:988–995.


21. Swayne DE, Tyler DE, Batker J. Cerebral infarction with associated venous thrombosis in a dog. Vet Pathol. 1988. 25:317–320.

