Abstract
Organotypic slice cultures have been developed as an alternative to acute brain slices because the neuronal viability and synaptic connectivity in these cultures can be preserved well for a prolonged period of time. This study evaluated a stationary organotypic slice culture developed for the hypothalamic paraventricular nucleus (PVN) of rat. The results showed that the slice cultures maintain the typical shape of the nucleus, the immunocytochemical signals for oxytocin, vasopressin, and corticotropin-releasing hormone, and the electrophysiological properties of PVN neurons for up to 3 weeks in vitro. The PVN neurons in the culture expressed the green fluorescent protein gene that had been delivered by the adenoviral vectors. The results indicate that the cultured slices preserve the properties of the PVN neurons, and can be used in longterm studies on these neurons in vitro.
Brain slices have been used as important tools for studying the physiological and morphological properties of the neuronal circuit as an in vitro model. In most studies, experiments are performed using brain slices immediately after their preparation. However, the acute brain slice preparations have not been used in experiments over more than a day because the neuronal viability does not last for more than 12 h [6]. For this reason, several laboratories have developed methods for the long-term culturing of brain slices [10,11,20]. Among them, the stationary organotypic slice culture method using porous membrane filters allows long-term survival as well as a high degree of cellular differentiation and organization resembling that of the native tissue [1,3,4,11,18,20].
The paraventricular nucleus (PVN) is the major center that regulates the endocrine and autonomic functions for maintaining homeostasis. Neurons in the nucleus are composed of magnocellular and parvocellular neurons. Magnocellular neurons project to the pituitary gland and release oxytocin and vasopressin to the posterior hypophysis. On the other hand, parvocellular neurons project to either the adenohypophysis or the autonomic centers in the brain stem and spinal cord [21]. The neurons projecting to the adenohypophysis can be further classified by their hypophysiotropic releasing factors, including corticotropin-releasing hormone (CRH), thyrotropin-releasing hormone (TRH), and vasopressin [2]. However, the properties of individual groups of these neurosecretory cells were not well-known because an easy method with which to identify a specific neuron group from the heterogenous pools of PVN neurons is not available. Recently, a promising approach was introduced by Dr. Ueta's group, which generated transgenic rats expressing a vasopressin-enhanced green fluorescent protein (EGFP) fusion gene [23]. These transgenic rats will be valuable tools for studying the properties of the neurosecretory cells releasing vasopressin in the PVN, but such transgenic animals are not yet available for the majority of the other neurosecretory cells in the PVN.
Using a stationary organotypic slice culture, Fields et al. [9] demonstrated the possibility that fluorescent proteins such as green fluorescent protein (GFP) could be expressed in selective neuron groups in the hypothalamus in vitro. This approach can allow us to study the electrophysiological properties of various neurosecretory PVN neurons if a specific group of neurons can be selectively marked with reporters such as GFP by gene transfer using viral vectors. In this study, we characterized the properties of PVN neurons in an organotypic slice culture of the hypothalamic coronal slice.
Sprague-Dawley rats (7-10 days old; Samtaco, Korea) were maintained under conditions of constant temperature and humidity, on a 12 h light/dark cycle (lights on at 8 : 00 A.M.), with ad libitum access to food and water. The animal experiments were carried out according to the protocol for the care and use of animals, which was approved by the Laboratory Animal Care Advisory Committee of Seoul National University, Korea.
The protocol used for the organotypic culture of hypothalamic slices in this study (Fig. 1) was modified from the protocols previously described by House et al. [15] and Stoppini et al. [20]. The aim of all modifications was to obtain slices with improved cell survival and normal physiological and morphological properties. Seven- to 10-day-old Sprague-Dawley pups were used. This age corresponds to the end of the period of hyporesponsiveness to stress of the hypothalamus-pituitary-adrenal axis [24]. The rats were decapitated and their brains were quickly removed from the skull. Under aseptic conditions, the brains were cooled in ice-cold Grey's balanced salt solution (GBSS; Sigma, USA) saturated with carbogen (95% O2/5% CO2 mixture) for 5 min, and the hypothalamic tissue was blocked. The tissue was submerged in chilled GBSS enriched with glucose (5 mg/ml), and 200 µm thick sections were cut from the anterior to posterior using a Vibratome (Vibratome, USA). Sections including the PVN were selected and trimmed dorsally at the level of the anterior commissure and lateral to the supraoptic nucleus. After preincubation of the slices in GBSS at 4℃ for 1 h, the slices were placed on Millicell-CM filter inserts (30 mm in diameter; Millipore, USA), which were transferred to a 6-well plate (Nunc, Denmark) containing 1 ml of the culture medium. The slices were incubated at 36℃ in a 5% CO2 atmosphere. The medium was typically changed every 48 h.
The standard medium (310 mOsm/l, pH 7.3) was composed of a mixture of 50% basal Eagle's medium (Gibco BRL, USA), 25% Hank's balanced salt solution (Gibco BRL, USA), 25% heat-inactivated horse serum (Gibco BRL, USA). In order to prevent the overgrowth of glial cells, 0.01mM cytosine arabinoside, uridine, and fluorodeoxyuridine (Sigma, USA) were added to the standard medium for the first 24 h. Penicillin (25 µg/ml), streptomycin (25 µg/ml), and neomycin (50 µg/ml) were added to the medium as antibiotics for the first 3 days. Serum-free medium (SFM) contained 100% basal Eagle's medium, 1.0 mM sodium pyruvate, and 3% bovine serum albumin (300 mOsm/L, pH 7.3), and was applied after 11 days in vitro (DIV). All media were changed every 2 days, except the the SFM, which was changed every day. All media were supplemented with 5 mg/ml glucose and 1 mM glutamine.
The slices were cultured in standard medium for 11 days and in SFM for 3 days, and were then used for the electrophysiological recordings, immunocytochemistry, or viral infection.
After 10 DIV, the slices adhering to the filters were immersed in 4% paraformaldehyde/0.1 M phosphate buffer (PB, pH 7.2) for 2 h at 4℃. The filters were used with a supporting plastic frame. After rinsing three times with 0.01 M phosphate-buffered saline (PBS, pH 7.4), the tissues on the membranes were stained with cresyl violet solution (0.5%) for 5 min. After rinsing in distilled water for 30 sec, the filters were excised from their plastic frame and then mounted on the silane-coated glass slide. The slice explants on the filter membrane on a slide glass were dehydrated in a graded series of ethanol (1 min each, 70%, 90%, 95%, and 100%), cleared in xylene (twice, 10 min), and mounted between the glass slide and coverslips in Canada balsam.
At the end of the experiments, the cultured slices maintained on filter membranes were immersed in 4% paraformaldehyde/0.2% picric acid in 0.1 M phosphate buffer for 2 h at 4℃. The filters were excised from the plastic frame, and the immunocytochemical procedure was performed in the culture dishes while the cultured slices remained on the surface of the support membrane. After rinsing three times with 0.01 M PBS, the cultured slices were blocked with 10% donkey serum/0.3% Triton X-100 (Sigma, USA) for 2 h in order to prevent nonspecific binding. The filters were then incubated with the rabbit anti-oxytocin antibody (1 : 1000; Chemicon, USA), rabbit anti-vasopressin antibody (1 : 1000; Chemicon, USA), or rabbit anti-CRH antibody (1 : 100; Chemicon, USA) diluted in PBS containing 0.1% BSA and 0.3% Triton X-100 for 48 h at 4℃. After incubation with the primary antibody, the cultures were further incubated with a fluorescence-conjugated secondary antibody, Alexa Flour 488 or 555 labeled donkey anti-rabbit IgG (1 : 200; Molecular Probes, USA) or a biotinylated secondary antibody (1 : 200; Molecular Probes, USA), for 2 h at room temperature. After washing four times with phosphate buffer, the slices were detached using a No. 0 or a No. 1 fine brush. When using the biotinylated secondary antibody, the cultures were rinsed three times with PBS and then incubated with biotinylated horseradish peroxidase (1 : 500) for 1 h (Vectastain Elite ABC kit; Vector, USA). The chromogen 3,3' diaminobenzidine tetrahydrochloride (DAB reaction kit; Vector, USA) was then applied to the filters with or without nickel sulphate. The slices were then placed on a silane-coated glass slide and mounted in antifade mounting media, 0.1% p-phenylenediamine in 50% glycerol-based phosphate buffer (20 mM, pH 8.5).
The whole-cell currents from the PVN neurons in the slice explants were recorded as reported previously, with the visualization of individual neurons [8,13]. In brief, the pipettes (2-5 MΩ) were filled with a solution containing 135 mM K-gluconate, 5 mM KCl, 0.5 mM CaCl2, 5 mM EGTA, and 5 mM MgATP (pH 7.2 with KOH). The membranes of the Millicell-CM filter inserts were carefully isolated with the slice explants, placed in the recording chamber (0.7 ml), and fixed with a grid made from nylon stocking threads supported by a U-shaped silver wire weight while being perfused (2 ml/min) with oxygenated (95%O2-5%CO2) artificial cerebrospinal fluid at 30-33℃. The individual neurons were identified using an upright microscope with differential interference contrast (BX50WI; Olympus, Japan) for whole-cell patch recording. Electrical signals were recorded by an Axoclamp 2B amplifier (probe gain, × 0.01 MU with HS-2 probe; Axon Instruments, USA). Current or voltage signals were filtered at 1 kHz and digitized at 10 kHz with an analog-digital converter (Digidata 1200; Axon Instruments, USA) and the pClamp program (Version 8; Axon Instruments, USA). Membrane potential was presented after correction of liquid junction potential (~14.4 mV).
An EGFP expression adenovirus, AdEGFP, was constructed according to the manual of the AdEasy vector system (Q-BIOgene, USA). The EGFP gene was excised from pEGFP-N1 with KpnI and NotI, and was ligated into the same sites of the adenovirus construction shuttle vector, the pShuttle-CMV vector (Q-BIOgene, USA). In order to create the recombinant adenovirus vector, the shuttle vector carrying this EGFP marker was linearized with PmeI and cotransformed with the pAdEasy-1 vector (Q-BIOgene, USA) into BJ5183 bacteria cells. After screening and confirming of the positive recombinant vector, this vector was linearized with PacI and then transfected to the human embryonic kidney (HEK) 293 cells using the calcium phosphate precipitation method. The transfected HEK 293 cells were overlaid with 0.5% agarose and 10% fetal calf serum in Dulbecco's modified Eagle's medium (Gibco BRL, USA). After the recombinant plaque appeared, the recombinant virus was plaque-purified twice. The AdEGFP-infected HEK 293 cells were easily selected from these purified recombinant viruses because the cells emitted a green color under a fluorescence microscope. The AdEGFP virus titer of the stock solution was 1011 plaque-forming units ml-1.
After 11 DIV, the virus stock was mixed with an equal volume of the culture medium, pre-warmed to 36℃, and then applied to the cultured slices. After 4 h, the slices were washed with the culture media and incubated for 3 days before the EGFP expression was examined in the cultured slices.
The coronal hypothalamic slices placed on the filter insert membrane were evaluated after 24 h of incubation in vitro. The majority of slices were attached to the membrane without additional treatments such as plasma clotting. As shown in Fig. 2, the outline of the slice explant became dim at 4 DIV, the surface area increased progressively, and the slice explant become more translucent with time. The typical thickness after fixation was < 80 µm in standard medium. The dead cells and debris appeared as dark spots at the bottom of the membrane. The overall shape and organization of the cultured hypothalamic slices were well-preserved for up to 3 weeks after explantation. The cultured slices were thin enough for immunocytochemistry to be performed on the filter membrane as whole mounts without resectioning. As shown in Fig. 3A, cresyl violet staining of the cultured slices at 11 DIV showed the third ventricle and dense staining of neurons in the PVN located bilaterally. Fig. 3C also shows the PVN neurons in the slice explants with clear contouring and processes at 17 DIV. In an attempt to record the electrophysiological properties of the PVN neurons in the slice explants, we were able to identify the type of PVN neurons and basic electrophyiological properties of the neurons tested (Fig. 3D). The neuron, which was identified as a type II PVN neuron [22], showed a resting membrane potential and input resistance of -62.4 mV and 482MΩ, respectively. The amplitude and the duration at half amplitude of the first action potential were 57 mV and 0.81 ms, respectively. The resting membrane potential was similar to those of type II PVN neurons in the acute slices (-55~-58 mV) [8,13]. In general, the neurons in the organotypic slice culture were more fragile than the neurons in the acute slice in the artificial cerebrospinal fluid, and more care was required in creating a tight seal and/or whole cell configuration in the patch clamp experiments.
The topographical organization of the hypothalamic PVN on the cultured slices was examined by performing immunocytochemistry against oxytocin, vasopressin, and CRH, which are the major cell groups in the magnocellular and parvocellular regions of the PVN. The immunoreactivity for oxytocin and vasopressin in the neurons of the PVN indicates that the neurons of the magnocellular region retained their anatomical properties in cultured slices (Fig. 4A & B). CRH neurons were identified in both the parvocellular and magnocellular regions, as shown in Figs. 4C and D.
The expression of the transgene using viral vectors in the cultured hypothalamic slices was examined by the addition of adenovirus containing the EGFP gene in the cultural medium. The addition of the adenoviral stock solution (1 µl) to the culture medium at 14 DIV expressed EGFP in the PVN neurons in the slice explants after 48 h. As shown in Fig. 5, PVN neurons expressing EGFP were found throughout the cultured hypothalamic slices including the PVN without an apparent specific cellular or regional pattern, except at the edges of the cultured slices. The EGFP was expressed on the cell body, axon, and dendrites, which allowed the whole structure of the neurons in the slice explants to be observed (Fig. 5).
These results showed that the PVN in the slice explants can be maintained for up to 3 weeks. In this organotypic slice culture, the architecture of the PVN and the expression of oxytocin, vasopressin, and CRH in the PVN were similar to those in the acute slices, which indicated that the PVN neurons in the organotypic slice culture maintain the typical functional integrity of the PVN. In addition, the administration of the GFP-adenovirus into the culture medium directly resulted in the expression of GFP in the cultured slices.
In this study, the stationary organotypic slice culture method established all the stationary organotypic slice culture features that have been reported previously [1,17,19]. One of the important advantages of this method is that the slice explant is thin, but preserves the functional integrity of the neurons in the specific nuclei such as the PVN. Almost all of the individual neurons in the hypothalamic slices can be visualized by immunocytochemistry without further sectioning. It also allows for one to study intrinsic physiological functions of the PVN neurons, including the gene expression and release of hypophysiotropic hormones [1,3,18].
Another advantage of stationary organotypic slice culture is its use in the study of gene transfer because most of the constraints and limitations inherent in direct in vivo gene transfer in a living brain can be avoided [16]. In addition, gene delivery using an organotypic slice culture is economical in terms of the use of animals because a large number of cultures can be prepared from the brain of a single animal [5,14]. In addition, the infected organotypic slice cultures can be refined to design more complex experiments such as 1) the labeling of specific cell groups with various fluorescence proteins with promoters for specific hypophysiotropic hormones such as vasopressin, CRH and TRH, 2) the coinoculation of two candidate genes (with or without cell-specific promoters) either into a single or into two different areas of one or more co-cultured slices.
The stationary organotypic slice culture also has some disadvantages. In the present study, both CRH and vasopressin neurons appear more numerous in the magnocellular division than the parvocellular division. Our results are similar to those of the report by Bartanusz et al. [3], in which CRH neurons were more numerous in the magnocellular division of the PVN, but different from the previous reports [1,18] in that only parvocellular but not magnocellular AVP neurons survive well in a given culture condition. This discrepancy could arise from variation of culture conditions such as osmolarity. It has been reported that vasopressin neurons survive better in low osmolarity (255 mOsm/l) and oxytocin neurons survive better in moderate osmolarity (300 mOsm/l), whereas neuroendocrine cells such as TRH and GHRH survive better at 320 mOsm/l [17]. Further study is needed to understand the factors affecting the survival and distribution of various neuroendocrine neurons in the PVN under this experimental conditions, including osmolarity (310 mOsm/l).
Overall, the organotypic slice cultures of the PVN established in this study preserve the known properties of the PVN neurons, which highlight their potential use as an in vitro model for the long-term study of the morphology and physiological mechanisms in the PVN of the hypothalamus.
Figures and Tables
Fig. 1
A schematic diagram of a stationary organotypic slice culture. After decapitating young rats at postnatal 7-10 days, the isolated brain was dissected to 200 µm using a Vibratome and placed on a filter insert under sterile conditions. Filter inserts were placed in 6-well plates containing the culture medium, and the cultures were grown in a CO2 incubator.
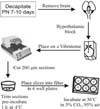
Fig. 2
Low magnification (× 40) views of a rat hypothalamus slice-explant at 0 (A), 4 (B), 10 (C), and 14 DIV (D). Note that the slice became more translucent on later days, indicating that slice thickness decreases with the length of incubation. DIV, day in vitro.
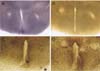
Fig. 3
The paraventricular nucleus and the electrophysiological recording from PVN neurons in the slice culture. (A) and (B), Cresyl violet staining of the cultured hypothalamic slices containing the PVN after 11 DIV (× 40). The area of the nucleus marked by a square in (A) is presented at higher magnification in (B) (× 100). (C), PVN neurons in a slice culture at 17 DIV. Scale bar = 10 µm. (D), Voltage responses to a depolarizing current pulse (60 pA, 250 ms) with a hyperpolarizing prepulse (-90 pA, 250 ms) from the neuron marked by the arrow in (C).
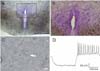
Fig. 4
Immunocytochemistry of oxytocin, vasopressin, and CRH in the PVN of the cultured slices. The PVN neurons were stained with antibodies against oxytocin (A), vasopressin (B), and CRH (C and D). D shows the CRH-immunoreactive cells in the area marked in C. Note that oxytocin and vasopressin neurons are distributed in the magnocellular regions, but the CRH neurons are distributed in both the magnocellular and parvocellular regions. 3V; third ventricle. bar = 100 µm.
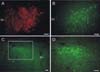
Fig. 5
Expression of EGFP in the neurons in the cultured slices. The EGFP gene was delivered by the adenoviral vectors. Low magnification (× 40) views at the bright-field (A) and under the fluorescent filter for EGFP (B). High magnification (× 400) views of the neurons in the left (C) and right PVN (D) showing the detailed anatomy of the neurons, including the cell bodies, processes, and possible contacts between neurons. The 2 horizontal lines are parts of the stabilizing net in the chamber.
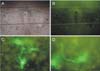
Acknowledgments
The authors thank Dr. Seung Yub Shin, Mr. Tae Hee Han, and Mr. Jian Hua Yang for their technical assistance. This work was supported by a grant (R01-2002-000-00128-0) from the Basic Research Program of the Korea Science and Engineering Foundation, and was performed as a portion of the Master's degree of the late Dr. Eun Seong Cho.
References
1. Arima H, House SB, Gainer H, Aguilera G. Neuronal activity is required for the circadian rhythm of vasopressin gene transcription in the suprachiasmatic nucleus in vitro. Endocrinology. 2002. 143:4165–4171.


2. Armstrong WE. Paxinos G, editor. Hypothalamic supraoptic and paraventricular nuclei. The Rat Nervous System. 2004. 3rd. San Diego: Elsevier Academic Press;369–388.


3. Bartanusz V, Muller D, Gaillard RC, Streit P, Vutskits L, Kiss JZ. Local gamma-aminobutyric acid and glutamate circuit control of hypophyseotrophic corticotropin-releasing factor neuron activity in the paraventricular nucleus of the hypothalamus. Eur J Neurosci. 2004. 19:777–782.


4. Belenky M, Wagner S, Yarom Y, Matzner H, Cohen S, Castel M. The suprachiasmatic nucleus in stationary organotypic culture. Neuroscience. 1996. 70:127–143.


5. Benediktsson AM, Schachtele SJ, Green SH, Dailey ME. Ballistic labeling and dynamic imaging of astrocytes in organotypic hippocampal slice cultures. J Neurosci Methods. 2005. 141:41–53.


6. Bergold PJ, Casaccia-Bonnefil P. Preparation of organotypic hippocampal slice cultures using the membrane filter method. Methods Mol Biol. 1997. 72:15–22.


7. Bertini LT, Kursner C, Gaillard RC, Corder R, Kiss JZ. A tissue culture model of the hypophysiotrophic CRH producing neuronal system. Neuroendocrinology. 1993. 57:716–728.


8. Chong W, Li LH, Lee K, Lee MH, Park JB, Ryu PD. Subtypes of α1- and α2-adrenoceptors mediating noradrenergic modulation of spontaneous inhibitory postsynaptic currents in the hypothalamic paraventricular nucleus. J Neuroendocrinol. 2004. 16:450–457.


9. Fields RL, House SB, Gainer H. Regulatory domains in the intergenic region of the oxytocin and vasopressin genes that control their hypothalamus-specific expression in vitro. J Neurosci. 2003. 23:7801–7809.


10. Gähwiler BH. Organotypic monolayer cultures of nervous tissue. J Neurosci Methods. 1981. 4:329–342.


11. Gähwiler BH, Capogna M, Debanne D, McKinney RA, Thompson SM. Organotypic slice cultures: a technique has come of age. Trends Neurosci. 1997. 20:471–477.


12. Graulich J, Hoffmann U, Maier RF, Ruscher K, Pomper JK, Ko HK, Gabriel S, Obladen M, Heinemann U. Acute neuronal injury after hypoxia is influenced by the reoxygenation mode in juvenile hippocampal slice cultures. Brain Res Dev Brain Res. 2002. 137:35–42.


13. Han SK, Chong W, Li LH, Lee IS, Murase K, Ryu PD. Noradrenaline excites and inhibits GABAergic transmission in parvocellular neurons of rat hypothalamic paraventricular nucleus. J Neurophysiol. 2002. 87:2287–2296.


14. Hilton KJ, Bateson AN, King AE. A model of organotypic rat spinal slice culture and bolistic transfection to elucidate factors that drive the preprotachykinin-A promoter. Brain Res Brain Res Rev. 2004. 46:191–203.


15. House SB, Thomas A, Kusano K, Gainer H. Stationary organotypic cultures of oxytocin and vasopressin magnocellular neurones from rat and mouse hypothalamus. J Neuroendocrinol. 1998. 10:849–861.


16. Keir SD, House SB, Li J, Xiao X, Gainer H. Gene transfer into hypothalamic organotypic cultures using an adeno-associated virus vector. Exp Neurol. 1999. 160:313–316.


17. Kusano K, House SB, Gainer H. Effects of osmotic pressure and brain-derived neurotrophic factor on the survival of postnatal hypothalamic oxytocinergic and vasopressinergic neurons in dissociated cell culture. J Neuroendocrinol. 1999. 11:145–152.


18. Kuwahara S, Arima H, Banno R, Sato I, Kondo N, Oiso Y. Regulation of vasopressin gene expression by cAMP and glucocorticoids in parvocellular neurons of the paraventricular nucleus in rat hypothalamic organotypic cultures. J Neurosci. 2003. 23:10231–10237.


19. Leutgeb JK, Frey JU, Behnisch T. LTP in cultured hippocampal-entorhinal cortex slices from young adult (P25-30) rat. J Neurosci Methods. 2003. 130:19–32.


20. Stoppini L, Buchs PA, Muller D. A simple method for organotypic cultures of nervous tissue. J Neurosci Methods. 1991. 37:173–182.


21. Swanson LW, Sawchenko PE. Hypothalamic integration: organization of the paraventricular and supraoptic nuclei. Annu Rev Neurosci. 1983. 6:269–324.


22. Tasker JG, Dudek FE. Electrophysiological properties of neurones in the region of the paraventricular nucleus in slices of rat hypothalamus. J Physiol. 1991. 434:271–293.


23. Ueta Y, Fujihara H, Serino R, Dayanithi G, Ozawa H, Matsuda K, Kawata M, Yamada J, Ueno S, Fukuda A, Murphy D. Transgenic expression of enhanced green fluorescent protein enables direct visualization for physiological studies of vasopressin neurons and isolated nerve terminals of the rat. Endocrinology. 2005. 146:406–413.

