Abstract
This study was performed to evaluate the effect of beta-tricalcium phosphate and poly L-lactide-co-glycolide-co-epsilon-caprolactone (TCP/PLGC) membrane in the repair of partial bone defects in canine proximal humerus. Three adult mixed-breed dogs were used during the experimental period. The length of the defect was quarter of the full length of humerus, and width of the defect was quarter of middle diameter of the lateral aspect of humerus. The humeri of each dog were divided into treatment (TCP/PLGC) and control groups. The defect was covered with TCP/PLGC membrane in treatment group. To evaluate regeneration of the bone, computerized tomography (CT) and histopathologic examination were performed. The radiopaque lines were appeared at the original defect sites in TCP/PLGC group but below the original site in control at 4th week. Radiopacity and thickness of the defect sites, and radiopaque lines were more increased at 8th week than those of 4th week. Histopathologic findings revealed fibrous connective tissue migration into the defect and the migration inhibited the structure of new cortex to be placed in the original level in control whereas new cortex growth was found in the level of original line in TCP/PLGC group. However, the new cortical bone in the TCP/PLGC group was thinner and less organized than the adjacent intact cortex, and the amount of new cancellous bones were also scanty. The result suggested that TCP/PLGC membrane is a good guided bone regeneration material to restore the original morphology of humerus in partial defect.
A number of bone transplantation techniques and graft materials have been developed for the repair of comminuted fractures, bone defects, osteomyelitis, non-union, and arthrodesis [19]. Among these materials and surgical techniques, autogenous bone graft is the most popular method because of its histocompatibility, osteoconductivity, and low cost. Cancellous bone donor sites used in veterinary medicine include the proximal portions of the humerus and tibia and the wing of the ilium [10] where the iliac wing, ribs, and coccygeal vertebrae as the source of corticocancellous bone [7]. Proximal portion of humerus was superior to the tibia as a donor site for cancellous bone because it yielded larger quantities of cancellous bone and undergoes more rapid and complete healing than the tibia [21,23,25].
Donor-site morbidity and risk of postoperative fracture after harvesting of bone is of great concern for the veterinarians. Major complications in the donor site of the tibia were seroma formation, wound disruption and the fracture of tibial cortex [11]. It was reported that incidence of fracture in the donor site occurred 10% patients [3]. Imperfectly healed up donor site may not only cause fatigue fracture, but also render it unusable. Cortical defects in long bone are the sites of stress concentration, decreasing the energy absorbing capacity by 30~70% [22]. The defect of the donor sites after harvesting of bone should be completely regenerated as early as possible. However, complete repair of the bone in the defect was disturbed by the ingrowth of connective tissue into the defect [12]. To prevent the ingrowth of connective tissue, guided bone regeneration (GBR) method has been introduced [20]. GBR technique, excluding connective tissue and epithelium growth from the defect, has been successfully applied in the treatment of periimplant bone defects [6]. Severe mechanical and chemical properties are required for biodegradation of GBR membrane. Composite biodegradable membranes were reported to fulfill the conditions for biodegradation [8,9,13~17].
Rapid and complete healing of the partial bone defects is important for the normal movement and activity of the donor animals within a short period of time. Composite biodegradable materials may be of great help for the partial defects left after collection of cancellous bone. GBR has been applied for the treatment of bone defects around implants in the mandible or segmental bone defect model [6,12,16,17]. However, the effect of GBR on healing of partial bone defect has not been evaluated. This study was performed to evaluate the effect of beta-tricalcium phosphate and poly L-lactide-co-glycolide-co-epsilon-caprolactone (TCP/PLGC) membrane as a GBR material for the repair of partial bone defects in canine proximal humerus, and to explore the prospective of TCP/PLGC in donor-site remodeling.
Three mixed-breed clinically healthy male dogs were used weighing 2.5 to 8.5 kg and age ranged from 1 to 2 years. The dogs were allowed for free movement in the cage with adequate food and water ad libitum. The left humerus of each dog was used for treatment group (TCP/PLGC) where the partial defect was coverd by TCP/PLGC membrane, and TCP/PLGC membrane was not applied in control group. Animal was handled according to the Animal Research Committee of the Seoul National University.
TCP/PLGC is the composite of poly L-lactide-co-glycolideco-epsilon-caprolactone (PLGC) and beta-tricalcium phosphate (TCP) which are used as matrix and filler, respectively. Both PLGC and TCP are biodegradable through hydrolysis, and the TCP also has good osteoconductivity [17]. This prosthetic membrane has thermoplasticity suitable for shaping according to bone defects, hardness to resist against external forces and pH autoregulation property to prevent inflammation by an acidic condition caused from decomposing of the PLGC.
All dogs were premedicated with acepromazine malate (0.01 mg/kg, IV, Samwoo, Korea), meloxicam (0.1 mg/kg, IM, Boehringer Ingelheim, Korea) and atrophine sulfate (0.05 mg/kg, SC, Jeil, Korea). Cephazolin sodium (22 mg/kg, IV, Chong Kun Dang, Korea) was also administered for prophylaxis. Anesthesia was induced with thiopental sodium (15 mg/kg, IV, Joongwei, Korea) and maintained with 2% isoflurane (Ilsung, Korea) with oxygen supply at a flow rate of 100 ml/kg/min. Lactated Ringer's solution (10 ml/kg/h, IV, Daehan Pharm, Korea) was administered during the surgical procedure.
The proximal humerus was exposed by craniolateral skin incision. Partial defects were created with sterile carbon steel blade, curette and a mallet in the lateral aspect of the humerus. Defects were produced by removal of cortical bone and bone defect sites were trimmed with curette. The length of the defects was a quarter of the full length of humerus, and width of the defects was quarter of middle diameter of humerus. Defects were covered with TCP/PLGC membrane in the treatment group and without any membrane in the control. Fascia and skin were closed routinely without any external support to the defects. Postoperative cares were continued with cephadroxil monohydrate (22 mg/kg, PO, Kukje, Korea) and carprofen (2.2 mg/kg, PO, Pfizer, USA) twice a day for 7 days. All dogs were restricted in the cage for 8 weeks.
Computerized tomography (General Electronics Medical System, Japan) was performed to evaluate regeneration of the defect at 1st, 2nd, 4th, and 8th week after surgery. Initially scout lateral and caudocranial views were scanned. Thereafter transverse planes were acquired with 1mm of thickness with 120 kVp and 60 mAs axial scanning setting.
All dogs were euthanized and humeri were collected for histopathologic examination at the 8th week after surgery. The specimens were fixed in 10% buffered formalin and decalcified in decalcifying solution , nitric acid and ethanol. Hematoxylin and eosin staining was performed for light microscopic examination after routine tissue processing and paraffin embedding.
Until 2 weeks after surgery, no change was noted in both groups on computerized tomography (CT) scan. The density of bone defects did not increase and there were clear margins to adjacent normal cortical and cancellous bones at this early stage. Mild irregular radiopaque lines were appeared over the bone defect area in both groups (Fig. 1). Radiopaque lines started appearing in the original site in TCP/PLGC group while in control below the defects. Same pattern of radiopacity at defect sites was marked at 8th week but the intensities were more than that of 4th week (Fig. 2). The thickness and radiopacity of the lines in control group were increased more than those in TCP/PLGC group.
In the control group, fibrous connective tissue migrated into the defect and contacted the new cortex (Fig. 3). The degree of cancellous bone formation is less prominent than that of adjacent intact area. New cortical bone was almost same in thickness compared to adjacent cortex, and microstructures of cortical bone seemed fully matured. However, in the TCP/PLGC group, there was no connective tissue migration into bone defect area (Fig. 4). The new cortical bone was thinner and less organized than the adjacent intact cortex and that of control group. The amount of new cancellous bone was also less.
Humeral fracture is the least common in small animals. Anatomically, humerus is good site for cancellous bone harvesting because there is abundant cancellous bone [5,21,23,24]. However, evidence of fracture of the humerus through the hole used to obtain the graft was recorded [5]. There was no consequence of fractures in the humeri during our experimental periods. Clark et al. [4] reported that increasing the width of the hole caused a significant reduction of strength, while increasing the length did not. In the present study, the length of the defect created was a quarter of the full length of humerus, and the width was a quarter of middle diameter of the humerus. It was considered that the defect was cylinder like, and seemed to be ideal size for collecting cancellous-cortical graft. Radiography was used to observe new bone formation in the defect in the previous reports [2,12] but in the present study CT was employed to avoid superimposition of bone defect and visualize new bone formation clearly with circumferential cortex remained. In TCP/PLGC group, radiopaque lines were placed in the original site, but in control, the lines were below the original outer cortex. The radiopaque line on the level of original defects indicated the new bone formation in the right sites.
In histopathological findings, fibrous connective tissue invaded into the defect and contacted with new cortical bone in the control group. There was no invasion of fibrous connective tissue into the defects in TCP/PLGC group. These findings suggested that TCP/PLGC membrane protected the invasion of fibrous connective tissue into the defect and seemed to be an emerging technique for bone defect repair in dogs. Similarly the use of GBR techniques has become a standard technique for bone regeneration in human dentistry [18]. The method has also been tried for successful recovery for segmental defect in long bone [12]. TCP/PLGC composite could provide a good room for healing the defects after harvest of cortex and cancellous bones.
In control group at 8 weeks after operation, new cortical bone grew upto almost the same thickness of the adjacent cortex, and microstructures of cortical bone appeared mature. Johnson [11] reported that none of the cortical defects in the medial proximal tibia was bridged by new cortical bone at 12 weeks. The difference of healing time between the present and previous studies was probably due to the different location of defect sites. Restoration of cancellous bone defect was more rapid and complete in the humerus than in the tibia [21]. The present study suggested that fibrous connective tissue which invaded the defect did not interfere with regeneration of cortical bone. Primary bone healing occurs in partial defect due to stable condition of the bone. However, secondary healing in segmental bone defect model was expected and the segmental defect would not heal without any filling materials. Extraosseus vascular supply is less abundant in medial proximal tibia because it has little amount of soft tissues than humerus. It is known that abundant blood supply from the connective tissue might play a role in accelerating defect healing. Regeneration rate of new bone in segmental bone defect model was higher in TCP/PLGC membrane treated animal than that of control [12].
The new cortical bone in TCP/PLGC group was thinner and less organized than the adjacent intact cortex and that in control group. The amount of new cancellous bone was also less than adjacent intact cancellous bone. These results indicate that the membrane delays healing of the defect. Extraosseous blood supply impeded by the membrane and only bone marrow around the defect participated in the healing. This is probably the reason why the difference of bone healing existed between TCP/PLGC and control groups. Therefore, TCP/PLGC membrane may be needed for the restoration of the defect to the original morphology. The membrane was observed until 8th week in CT image. Similarly the TCP/PLGC membrane was identified in the x-ray image at 12 weeks after implantation [12].
The principle of structural engineering indicated that the restoration of bone defect to the original morphology could be better for stress endurance than that of distorted morphology [1]. The present study suggested that TCP/PLGC membrane interferes healing of the defect, and this delay of the healing is helpful to restore the original morphology.
Figures and Tables
Fig. 1
Computerized tomography in the transverse planes of the middle of the defects at 4th week after surgery. Radiopaque lines appeared on bone defect area in both groups, control group (L1-3) and TCP/PLGC group (R1-3).
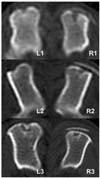
Fig. 2
Computerized tomography at the transverse planes of the middle of the defects at 8th week after surgery. Radiopaque lines were found in the same site of the 4th week scan, but their thickness and radiopacity were increased. L1-3 (Control group) and R1-3 (TCP/PLGC group).
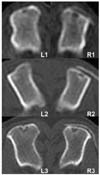
Fig. 3
Histopathologic findings of the bone defect in the control group at 8th week. Active periosteal reaction was observed, but the degree of cancellous bone formation is less prominent than that of adjacent intact area. (arrow: new regenerated bone; arrow-head: intact bone). H&E stain, ×40.
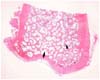
Fig. 4
Histopathologic findings of the bone defect in the TCP/PLGC group at 8th week. Active periosteal reaction was observed. The new cortex was recovered up to the original level. Regenerated cancellous bone seemed to be less amount compared with adjacent intact cancellous bone. (arrow: new regenerated bone; arrow-head: intact bone). H&E stain. ×40.
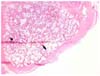
References
1. Arbabi F. Structural Analysis and Behavior. 1991. NewYork: McGraw Hill;244–245.
2. Artzi Z, Rohrer MD, Nemcovsky CE, Prasad HS, Tal H. Qualitative and quantitative expression of bovine bone mineral in experimental bone defects. Part 1: Description of a dog model and histological observations. J Periodontol. 2003. 74:1143–1152.


4. Clark CR, Morgan C, Sonstegard DA, Matthews LS. The effect of biopsy-hole shape and size on bone strength. J Bone Joint Surg. 1977. 59A:213–217.


5. Ferguson JF. Fracture of the humerus after cancellous bone graft harvesting in a dog. J Small Anim Pract. 1996. 37:232–234.
6. Hockers T, Abensur D, Valentini P, Legrand R, Hammerle CHF. The combined use of bioresorbable membranes and xenografts around implants. A study in beagle dogs. Clin Oral Implants Res. 1999. 10:487–498.


7. Hulsse DA. Pathophysiology of autologous cancellous bone grafts. Compend Contin Educ Pract Vet. 1980. 11:136–142.
8. Ignatius AA, Ohnmacht M, Claes LE, Kreidler J, Palm F. A composite polymer/tricalcium phosphate membrane for guided bone regeneration in maxillofacial surgery. J Biomed Mater Res. 2001. 58:564–569.


9. Jansen JA, de Ruijter JE, Jansen PTM, Paquay YGCJ. Histological evaluation of biodegradable polylactive/hydroxyapatite membrane. Biomaterials. 1995. 16:819–827.


10. Johnson KA. Cancellous bone graft collection from the tibia in dogs. Vet Surg. 1986. 15:334–338.


11. Johnson KA. Histologic features of the healing of bone graft donor sites in dogs. Am J Vet Res. 1988. 49:885–888.
12. Koyama Y, Kikuchi M, Yamada T, Kanaya T, Hiroko N, Tsumoto M, Takakuda K, Miyairi H, Tanaka J. Guided bone regeneration with novel bioabsorbable membranes. JSME Int J (Series C). 2003. 46:1409–1416.


13. Kikuchi M, Suetsugu Y, Tanaka J, Akao M. Preparation and mechanical properties of calcium phosphate/copoly-L-lactide composites. J Mater Sci Mater Med. 1997. 8:361–364.
14. Kikuchi M, Tanaka J, Koyama Y, Takakuda K. Cell culture test of TCP/CPLA composite. J Biomed Mater Res. 1999. 48:108–110.


15. Kikuchi M, Tanaka J. Chemical Interaction in beta-tricalcium phosphate/copolymerized poly-L-lactide composites. J Ceram Soc Japan. 2000. 108:642–645.


16. Kikuchi M, Koyama Y, Takakuda K, Miyairi H, Shirahama N, Tanaka J. In vitro change in mechanical strength of beta-tricalcium phosphate/copolymerized poly-L-lactide composites and their application for guided bone regeneration. J Biomed Mater Res. 2002. 62:265–272.


17. Kikuchi M, Koyama Y, Yamada T, Imamura Y, Okada T, Shirahama N, Akita K, Takakuda K, Tanaka J. Development of guided bone regeneration membrane composed of beta-tricalcium phosphate and poly (L-lactide-co-glycolide-co-epsilon-caprolactone) composites. Biomaterials. 2004. 25:5979–5986.


18. McGinnis M, Larsen P, Miloro M, Beck FM. Comparison of resorbable and nonresorbable guided bone regeneration materials: A preliminary study. Int J Oral Maxillofac Implants. 1998. 13:30–35.
19. Millis LD, Martinez AS. Slatter DH, editor. Bone Grafts. Textbook of Small Animal Surgery. 2003. 3rd ed. Philadelphia: Saunders;1875–1891.
20. Nyman S. Bone regeneration using the principle of guided tissue regeneration. J Clin Periodontol. 1991. 18:494–498.


21. Penwick RC, Mosier DA. Healing of canine autogenous cancellous bone graft donor sites. Vet Surg. 1991. 20:229–234.


22. Reilly DT, Burstein AH. Mechanical properties of cortical bone. J Bone Joint Surg. 1974. 56:1001–1022.
23. Palmisano MP, Schrader SC. Premature closure of the proximal physis of the humerus in a dog as a result of harvesting a cancellous bone graft. J Am Vet Med Assoc. 1999. 215:1460–1462.