This article has been corrected. See "Erratum: Characterization of HC58cDNA, a putative cysteine protease from the parasite Haemonchus contortus" in Volume 7 on page 405.
Abstract
Because of the complexity of the cathepsin B-like (CBL) family, an information on the biological and biochemical characteristics of individual CBL genes is lacking. In this study, we investigated the degradative effects of the recombinant HC58 protein isolated from Haemonchus contortus parasites on protein substrates over a broad pH range in vitro. This protein, which hydrolyzed the synthetic peptide substrates Z-FR-AMC and Z-RR-AMC, had characteristics of the cysteine protease class of proteins. In the acidic pH range, the isolated protein actively degraded hemoglobin (Hb), the heavy chain of goat immunoglobulin G, and azocasein. By contrast, it degraded fibrinogen in the alkaline pH range. These activities were strongly inhibited in the presence of the cysteine protease inhibitor E-64. While the protein digested Hb, it did not induce the agglutination of erythrocytes from its natural host. These results suggest that the HC58 protein may play a role in the nutrition of this parasite.
Haemonchus contortus is a highly pathogenic parasite affecting sheep, goats, and cattle. The adult parasite causes severe anemia, weight loss, and death, especially in young animals [12]. The adult nematode, located in the abomasum of ruminants, derives nutrients through bloodfeeding and the ingestion of tissue debris. After the proteolytic anticoagulants of Ancylostomum hookworms were first described [6], a considerable amount of interest arose in the role of proteinases in the maintenance of bloodfeeding parasitic helminthes [20]. The digestive proteases of schistosomes were extensively characterized [15], especially those involved in the digestion of hemoglobin (Hb) by the adult parasite. The main molecules responsible for Hb digestion in schistosomes are the cathepsin B-like cysteine proteases (CBLs) [16]. The intense proteinase activity of crude extracts from H. contortus is thought to be carried out by cathepsin B-like (CBL) enzymes [15]. The molecular cloning and sequencing of cysteine proteases with putative nutrient degrading properties from adult H. contortus was described in a series of reports [13,16]. Most authors have reported that intestinal CBLs constitute a large family of proteins in the parasitic nematode H. contortus [7,13,16,17]. CBL genes comprised the most abundant portion of the cDNAs analyzed in a small set of expressed sequence tags (ESTs) in the intestines of the adult female H. contortus [13,16]. The CBL proteins were localized to the microvilli of the intestines of adult H. contortus organisms, where active cysteine proteases are likely to hydrolyze ingested host proteins [8,13]. To date, however, the complexity of the CBL family has hindered our ability to characterize individual sequences or determine their biochemistry and function [16]. HC58 is an abundantly expressed CBL gene in H. contortus [5]. Its partial sequence, published as GenBank accession number AF305964, indicates that it might be a cathepsin B molecule. Its ubiquitous localization, however, differs from that of previously characterized H. contortus cathepsin B molecules. In this study, we investigated the degradation of several protein substrates by the recombinant HC58 protein over a broad pH range. The protein was further characterized on the basis of substrate specificity and inhibitor sensitivity.
Adult H. contortus worms were collected from goat abomasa, as previously described [5]. Total RNA was prepared from pooled parasite samples using the single-step protocol [3]. The 3'-and 5'-rapid amplification of complementary DNA ends (RACE) polymerase chain reaction (PCR) was performed using a 3' and 5'-Full RACE Core Set cDNA Kit (Takara Biotechnology, Japan) according to the manufacturer's instructions. Briefly, 5'-RACE cDNA was generated using a reverse transcription (RT) primer [5'-TGAATGCCGCTTG-3'] based on published mRNA sequence of accession number AF305964. The 5'-RACE PCR primers S1 [5'-TTT GCCAAGACTTTATCGAG-3'], A1 [5'-CATACAGGTTCC AAGATTT-3'], S2 [5'-TTTGCCAAGACTTTATCGAG-3'] and A2 [5'-AACAAAAGGACCAGTTCAAGC-3'] were used in concentrations of 20 pMol/µl each. The 3'-site adapter primer (provided in the kit) and the A3 primer (5'-ACGACCGTTCATTCAAGACA-3') were used at concentrations of 20pMol/µl each for the 3'-RACE PCR. The full-length HC58cDNA was amplified using a sense primer directed to the 5'-end of the coding region and an antisense primer directed to the 3'-polyA+ region of the target transcript. The product of this reaction was subcloned and sequenced, and the data were used to design primers to the 5' [5' AAGGATC CATGTCAGATAGGGCC-3'] and 3' [5'-CGAAGCTTTAG AAATCTCCAGCGA-3'] ends of the coding region. The primers contained XbaI and HindIII restriction enzyme recognition sequences that facilitated directional subcloning into the pET-28a expression vector.
Recombinant expression was induced using isopropyl-β-D thiogalactopyranoside (Sigma, UK) at a final concentration of 1 mM for 4 h at 37℃. Following the induction, bacterial pellets were collected and recombinant proteins were isolated under denaturing conditions using a method described by the manufacturer (Qiagen, USA). Briefly, the bacterial pellet was dissolved in a combination of 8 M urea, 0.1 M Na2HPO4, 0.01 M Tris-HCl (pH 8), and 1 M 2-mercaptoethanol, which was sonicated and centrifuged. The supernatant was applied to nickel-nitrilotriacetic acid (Ni-NTA) agarose. The NTA column was washed with 20 volumes of 8 M urea, 0.1 M Na2HPO4, and 10 mM Tris-HCl (pH 6.3), and then by a 20-column volume wash with 8 M urea and 50 mM Tris (pH 8.0). The recombinant HC58 protein was refolded in 0.1 M urea, 50 mM Tris-HCl, 2 M oxidized glutathione (Sigma, USA), and 0.02 M reduced glutathione (pH 8.0; Sigma, USA). Protein renaturation was carried out on the Ni-NTA column using a linear gradient over a period of 2 h. After renaturation, the proteins were eluted with 0.3 M imidazole in the refolding buffer. The eluted proteins were first dialyzed in 0.1 M urea and 50 mM Tris-HCl (pH 8.0) to remove the glutathione, then underwent dialysis in 0.05 mM urea and 50 mM Tris-HCl (pH 8.0). The protein concentration was determined, as previously described [2].
Cathepsin B activity was assayed using Z-RR-AMC (Sigma, USA) as a substrate, and cathepsin L activity was assayed using Z-FR-AMC in the presence of 5 mM dithiothreitol (DTT) at 25℃ with a automated microtiter plate spectrofluorometer multiscan RC device (Lab systems, Denmark), in a final volume of 165 µl per well. Cleavage of 7-amino-4-methylcoumarin (AMC) was measured using excitation and emission wavelengths of 405 nm and 455 nm, respectively. An increase in optical density (OD) of 0.1 units corresponded to 39.3 nMol of AMC released per microgram of HC58 protein per min. The same procedure was used for inhibition sensitivity assays, except that before the peptide substrates were added, we incubated the HC58 protein with E64, which had been added to a final concentration of 50 µM. Inhibition was determined spectrometrically in 3 replicate OD readings. Inhibitor activity was expressed as the percentage inhibition (%I) equivalent to 100 × (AI--AI+)/AI-, where AI- and AI+ are the activity with and without the inhibitor present, respectively. In addition, control assays of the buffer alone or the bacterial extracts alone (i.e., without the HC58 protein) were made against these substrates to determine the spontaneous background activity.
Stock Hb solution (32 mg/ml; Sigma, USA) was prepared in 0.1 M phosphate buffer at pH 6 in the presence of 5 mM DTT. Each assay consisted of 80 µg/ml, 1 mg/ml, or 2 mg/ml of recombinant HC58 protein with 80 µg/ml of Hb in a final volume of 10 µl incubated for 24 h at 37℃. Control assays were either Hb, recombinant HC58 only, or bacterial extracts (i.e., without recombinant HC58 protein) prepared under similar experimental conditions. The inhibition assays were prepared in a similar way, except that before adding Hb, we incubated the recombinant HC58 protein (1 mg/ml) with 1 µl of the inhibitor E64 (which had been added to a final concentration of 43 µM) for 30 minutes at 37℃. All assays were analyzed with 12% sodium dodecylsulfate-polyacrylamide gel eletrophoresis (SDS-PAGE) under reducing conditions.
Protein hemagglutination activity was determined as described previously [10]. Briefly, human blood type AB, A, B, and O samples, and goat, rabbit, chicken, rat, buffalo, and dog blood samples were collected in heparinized tubes. Erythrocytes were pelleted (1,500 rpm for 10 min), washed 3 times in a sterilizing 0.9% NaCl solution, and resuspended in the same buffer at concentrations of 2% (v/v). 25 µl of HC58 protein at 3 concentrations of 80 µg/ml (2 µl/well), 400 µg/ml (10 µg/well), and 1.6 mg/ml (40 µg/well) were serially diluted by reducing the concentration into half (2-fold) in a 0.9% NaCl saline solution, then 25 µl of the 2% erythrocyte suspension was added to each diluted sample in the 96-conical well microtiter plate. Negative 25-µl control samples of sterilized 0.9% NaCl and the 2% erythrocyte suspension per well (without HC58 protein) were included in each test plate. After standing for 1 h at room temperature, the wells were examined for evidence of agglutination.
The ability of recombinant HC58 protein to degrade immunoglobulin (Ig)G was tested by incubating 3 concentrations of the protein (80 µg/ml, 1 mg/ml, and 2 mg/ml) with 1.0 µl of goat IgG (25 mg/ml) in 10 µl of 0.1 M phosphatebuffered saline (PBS) (pH 7.0) incubation buffer for 16 hours at 37℃. The control assays were either IgG (1.0 µl) plus buffer alone or bacterial extracts and IgG (1.0 µl) alone (without HC58 protein) prepared under similar conditions. The inhibition sensitivity assays were similar, except before adding IgG (1.0 µl), we incubated recombinant HC58 protein (30 µg) with 1.0 µl of the inhibitor (E64, which had been added to a final concentration of 43 µM) for 30 min at 37℃. The outcome of the overnight digestion was analyzed using 12% reducing SDS-PAGE.
The substrates azocasein (15 mg/ml), Hb, fibrinogen, and goat IgG were purchased from Sigma (USA). The effect of pH on recombinant HC58 protein activity against these substrates was determined with different test buffers of overlapping pH in the range of 3 to 11, as follows; 0.1M acetate (pH 3.0-3.5), 0.1M phosphate (pH 5-7), 0.1 M Tris (pH 7-9), and 0.1 M glycine (pH 9-11). All test buffers were supplemented with penicillin (500 U/ml) and streptomycin (5mg/ml). Recombinant HC58 protein activity was determined, as previously described [9]. Briefly, each assay consisted of 10 µl of HC58 protein (2 mg/ml), 100 µl of test buffer, and 10 µl of antibiotic mixture incubated with either 10 µl of azocasein, Hb, fibrinogen, IgG substrates, or water blanks to a final volume of 130 µl for 16 h at 37℃. The undigested protein was precipitated by adding 130 µl of 5% trichloroacetic acid. After incubation on ice for 30 min, the precipitated protein was pelleted by centrifugation at 12,000 rpm for 10 min and the supernatant fraction was harvested for analysis. An 80-µl sample of the supernatant fraction was dispensed into microtiter plate wells, and a 120-µl sample of the assay buffer (500 mM Tris HCl, pH 8.8) was added to the plates just before the absorbance was read at 405 nm. The activity was calculated by subtracting the OD reading for the negative water blanks. Each analysis was performed in triplicate.
Nucleotide sequencing, combined with a search of the GenBank database, revealed 5 clones generated by 5'-RACE-PCR that had single 490-bp products, suggesting a single transcriptional start site (data not shown). Four other clones, generated by 3'-RACE-PCR, had complete 3' ends containing a polyadenylation tail of 17 adenosine units at a position 30 bp downstream of the stop codon, TAA. Another 10 clones had inserts of the HC58cDNA gene, as verified by XbaI and HindIII digestion and sequencing. The full-length 3 and 5 ends plus the complete HC58cDNA gene sequence consisted of 526 bp, 577 bp, and 851 bp, respectively, and had more than 99% homology with the H. contortus partial EST of accession number AF305964. The complete sequence of H. contortus HC58cDNA and inferred amino acids data reported in this paper are available in the GenBank database under accession number AY948978. The expression product in Escherichia coli migrated, as expected, as a 27-kDa band and was associated exclusively with isopropyl thiogalactose (IPTG)-induced cells visualized under reducing conditions (Fig. 1). This band was lacking in the empty pET-28a expression vector and in E. coli extracts transformed into pET-28a before induction (Fig. 1).
Cathepsin B and L activities were tested using the Z-RR-AMC and Z-FR-AMC synthetic substrates, respectively. The cathepsin L activity at protein concentrations of 80 µg/ml, 350 µg/ml, 1 mg/ml, and 1.6 mg/ml were 15.3 ± 5.35, 29.3 ± 12.55, 40.3 ± 17.21 and 42.95 ± 15.71 nMol AMC/mg/min, respectively. While the cathepsin B activity at similar concentrations was 10.7 ± 4.41, 17.2 ± 6.7, 27.4 ± 19.09 and 32.9 ± 10.7 respectively (Fig. 2), there was absolutely no activity in the assays of buffer alone (without HC58). After incubation with specific protease inhibitor E64 (50 µM), the percentage inhibition of activity in the presence of the Z-FR-AMC substrates was 87.9 ± 6.4 (80 µg/ml), 95.3 ± 8.6 (350 µg/ml) and 98.7 ± 17.06% (1 mg/ml), respectively. The corresponding inhibition of activity on Z-RR-AMC substrate was 80.1 ± 7.35, 88.6 ± 13.56, 90.7 ± 16.21%, respectively. The percentage inhibition of cathepsin L substrate (Z-FR-AMC) was significantly higher (p < 0.05) than the cathepsin B substrate (Z-RR-AMC) at similar protein concentrations (Table 1).
Recombinant HC58 protein digested hemoglobin at ~64.5 kDa and ~27 kDa bands (Fig. 3, lane 1, 2 & 3). At high protein concentrations of 1-2 mg/ml there was almost complete digestion of hemoglobin (Fig. 3, lane 2 and 3), while at low protein concentrations of 80 µg/ml there was partial digestion of hemoglobin (Fig. 3, lane 1). The presence of protease inhibitor E64 (43 µM) abolished Hb digestion completely, as indicated by the presence of prominent Hb bands (Fig. 3, lane 6). By contrast, no Hb degradation activity was observed in the assays of bacterial extract alone (i.e., without HC58) (Fig. 3, lane 7).
The recombinant protein was found to be blood-group specific. It exhibited higher hemagglutination titers in the presence of erythrocytes to human blood types AB, A, B, and O, as well as to dog, rabbit, and mouse blood and lower activity in the presence of chicken, goat, and buffalo blood at concentrations of 400 µg/ml (10 µg/well) and 1.6 mg/ml (40 µg/well). Hemagglutination of chicken and goat erythrocytes did not occur at lower protein concentrations (80 µg/ml or 2 µg/well) (Table 2). The protein induced hemagglutination in chicken erythrocytes at high concentrations (10 µg and 40 µg/well), but still did not induce agglutination in goat erythrocytes (Table 2, lane 5). In contrast, agglutination occurred in the negative control wells containing buffer and goat erythrocytes alone (without HC58 protein). This suggests that hemagglutination of goat erythrocyte samples was prevented by recombinant HC58 protein.
The protein tested degraded the heavy chain of goat IgG at all 3 concentrations under acidic conditions (pH 4) (Fig 4, lanes 4, 5, and 6) after 16 hours of incubation. By contrast, no degradation was evident at pH 7 and pH 9 (data not shown). The light chain was unaffected under similar conditions. The cysteine proteinase inhibitor E64 (50 µM) completely abolished IgG degradation by the protein (Fig 4, lane 3). Notably, bacterial extracts had no effect on the light or heavy chains of goat IgG (Fig. 4, lane 2).
The proteolysis of fibrinogen, Hb, azocasein, and goat IgG over a broad pH range is shown (Fig. 5). The pH profiles were determined spectrometrically at OD 405 nm on 3 separate occasions using freshly purified recombinant HC58 protein, and the same peaks of activity were detected. Notably, all of the substrates exhibited single-peak responses to pH. Under acidic conditions, fibrinogen, azocasein, and IgG had a sharp peak in activity at pH 4 to 5, compared with Hb, which had peak responses at a neutral pH. All of the substrates examined exhibited lower activity under alkaline conditions.
The study increases the amount of information available about the effect of cysteine proteases on peptides isolated from the adult H. contortus parasite. We found that the complete HC58cDNA gene shares many features characteristic of cathepsin B in the Clan CA of the papain family (Family C1), which suggests that it is a CBL protease. The synthetic peptide substrate specificity assays indicated that the principal proteolytic machinery for recombinant HC58 protein was that of the cysteine protease type. The protein cleaved both cathepsin substrates, with more hydrolysis occurring in Z-FR-AMC (a cathepsin L substrate) compared with Z-RR-AMC (a cathepsin B substrate) at similar protein concentrations used in the study. The majority of H. contortus gut proteases belong to the CBL family [14]. In contrast, most CBL enzymes cleave synthetic L substrates [15]. Multiple amino acid sequence alignments revealed several residue substitutions that could alter the specificity from a CBL to cathepsin L-like substrate [15]. A possible explanation for the slightly higher cathepsin L-like activity, despite its structural resemblance to cathepsin B sequences, could be the G-I-E-S-A amino acid sequence in HC58 (data not shown). The alanine (A) residue in the pocket confers cathepsin L activity instead of cathepsin B activity. The cathepsin B in vertebrates has an acidic group at the base of the S2 pocket position, which interacts productively with the guanadino group of arginine. In contrast, mammalian cathepsin L proteases have an alanine or other molecules at this position that cannot accommodate arginine binding [15]. Recombinant HC58 protein probably represents a CBL with broad substrate specificity covering the spectrum of cathepsin B to L, which may be a feature of nematode proteases.
The isolated protein digested a variety of protein substrates that are thought to be encountered by the parasite in the host stomach mucosa. Although these data in of themselves do not prove a specific role, they demonstrate that the protein has the capacity to hydrolyze a variety of peptide substrates, including Hb, which forms a major component of the parasite's blood meal. The digestion of Hb reported here is in accord with observations of nutrient digestion by cathepsin B and L protease subfamily in many parasites [14,20]. This, coupled with evidence of the hemoglobinase motif in the cDNA sequence (data not shown) previously described in the blood feeding parasites [1], suggests a possible role for this protein during blood meal feeding.
The acidic pH optima and single-peak profiles observed for the proteolysis of Hb (pH 5), goat IgG (pH 5), and azocasein (pH 4) by recombinant HC58 concur with the general pH profiles of maximum CBL enzyme activity under the acidic conditions of the host stomach [9]. Peak fibrinogen degradation occurred at pH 7 and concurred with the reported pH optima for fibrinogen extracts of H. contortus [11]. The observation of fibrinogen degradation at a neutral pH supports the possibility of an anticoagulant role for this parasite, which may be necessary for the survival of this obligatory bloodfeeding parasite.
The acidic hemoglobinase (pH 5) activity of the HC58 protein concurs with the hemoglobinase activity of Schistosoma mansoni, Necator americanus, and A. caninum [11], and confirms general observations of the role of cysteine proteases during blood meal feeding. The isolated protein was active against host immunoglobulins, showing specificity for the heavy chain of IgG instead of the light chain. Local antibodies were found to be important effector mechanisms against infections by T. circumcinta, a closely related abomasal parasite in sheep [18]. Cathepsin B cysteine proteases have been implicated as factors that help infectious organisms evade host immune defenses [16,19], and the degradation of IgG by HC58 protein reported here suggests a possible role for this protein in helping pathogens evade immune activity.
Figures and Tables
Fig. 1
Prokaryotic expression of the recombinant HC58 protein. Gels were stained with Coomassie blue and 15-µl samples were loaded per lane. lane M: standard protein molecular weight marker; lane 1: pET-28a empty expression vector, without HC58 cDNA insert (negative control); lane 2: E. coli (BL21) extracts transformed into pET-28a, before induction with IPTG; lane 3 and 4: extracts of E. coli (BL21) transformed with pET-28a HC58cDNA, after 3 and 4 hours of induction with IPTG, respectively; lane 5: supernatants of HC58 inclusion bodies extracted with 8 M urea; lane 6: the purified HC58 protein inclusion body of approximately 27 kDa.
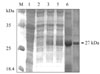
Fig. 2
Cathepsin B/L substrate specificity of the recombinant HC58 protein. Cathepsin B activity assays were performed using the Z-RR-AMC substrate and cathepsin L activity was assayed using Z-FR-AMC suspended in 0.1 M PBS pH 6.0, supplemented with 5 mM DTT. The activity was determined from triplicate assays done on 3 separate occasions with freshly prepared batches of recombinant HC58 protein. The cathepsin L substrate activity was significantly higher than the cathepsin B substrate activity at similar protein concentrations (p < 0.05).
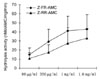
Fig. 3
Digestion of hemoglobin with recombinant HC58 protein. Lane P: protein molecular marker; lane 1: HC58 (80 µg/ml) incubated with Hb (80 µg/ml); lane 2: HC58 protein (1 mg/ml) incubated with Hb (80 µg/ml); lane 3: HC58 protein (2 mg/ml) incubated with Hb (80 µg/ml); lane 4: Hb(80 µg/ml) incubated in the absence of the recombinant HC58 protein; lane 5: HC58 protein (1 mg/ml) incubated in the absence of Hb substrate and inhibitor E64; lane 6: electrophoretic profile of HC58 (1 mg/ml) and Hb (80 µg) incubated in the presence of inhibitor E64 (43 µM), presence of inhibitor E64 completely abolished Hb digestion, as indicated by prominent Hb bands in lane 6; lane 7: Hb(80 µg/ml), incubated with extracts of the host bacteria carrying the empty pET-28a vector (without HC58 cDNA insert). Note complete and partial digestion of Hb lanes 1, 2, and 3.
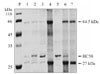
Fig. 4
Degradation of goat IgG by recombinant HC58 protein at pH 4. The outcome of overnight digestion (16 h) at pH 4 with varying protein concentrations analyzed on 12% SDS-PAGE under reducing conditions. lane 1: standard protein molecular marker; lane 2: bacterial extracts plus IgG (1.0 µl) without HC58; lane 3: 2 mg/ml HC58 protein plus IgG (1.0 µl) with E64 inhibitor; lane 4: IgG (1.0 µl) and 80 µg/ml HC58; lane 5: IgG (1.0 µl) and l mg/ml HC58; lane 6: IgG (1.0 µl) and 2 mg/ml of HC58; lane 7: IgG (1.0 µl) and buffer only control. Note the degradation of the IgG heavy chain in lanes 4, 5, and 6.
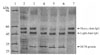
Fig. 5
The effect of pH on the degradation of protein substrates Hb, azocasein, fibrinogen and goat IgG by recombinant HC58 protein of adult H. contortus. The buffers 0.1 M acetate, 0.1 M phosphate, 0.1 M Tris and 0.1 M glycine with overlapping pH, in the ranges pH 3 to 11, supplemented with antibiotics were used.
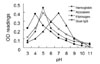
Table 1
Effects of various concentrations of the recombinant HC58 protein on cathepsin B and cathepsin L activity

Table 2
Hemagglutination of erythrocytes from different sources by recombinant HC58 protein

NB: The symbols AB, A, B, O indicate human blood group types. The numbers with exponents indicate HC58 hemagglutination titers and are shown as the reciprocal of the highest dilution capable of inducing a detectable level of agglutination of erythrocytes. One hemagglutination unit (HU) is the minimum HC58 concentration required for complete agglutination.
References
1. Baig S, Damian RT, Peterson DS. A novel cathepsin B active site motif is shared by helminth blood feeders. Exp Parasitol. 2002. 101:83–89.


2. Bradford MM. A rapid and sensitive method for the quantitation of microgram quantities of protein utilizing the principle of protein-dye binding. Anal Biochem. 1976. 72:248–254.


3. Chomczynski P, Sacchi N. Single-step method of RNA isolation by acid guanidinium thiocyanate-phenol-chloroform extraction. Anal Biochem. 1987. 162:156–159.


4. Geldhof P, Claerebout E, Knox DP, Jagneessens J, Vercruysse J. Proteinases released in vitro by the parasitic stages of the bovine abomasal nematode Ostertagia ostertagi. Parasitology. 2000. 121:639–647.
5. Hartman D, Donald DR, Nikolaou S, Savin KW, Hasse D, Presidente PJA, Newton SE. Analysis of developmentally regulated genes of the parasite Haemonchus contortus. Int J Parasitol. 2001. 31:1236–1245.


6. Hotez PJ, Cerami A. Secretion of a proteolytic anticoagulant by Ancylostoma hookworms. J Exp Med. 1983. 157:1594–1603.
7. Jasmer DP, Roth J, Myler PJ. Cathepsin B-like cysteine proteases and Caenorhabditis elegans homologues dominate gene products expressed in adult Haemonchus contortus intestine. Mol Biochem Parasitol. 2001. 116:159–169.


8. Jasmer DP, Mitreva MD, McCarter JP. mRNA sequences for Haemonchus contortus intestinal cathepsin B-like cysteine proteases display an extreme in abundance and diversity compared with other adult mammalian parasitic nematodes. Mol Biochem Parasitol. 2004. 137:297–305.


9. Knox DP, Redmond DL, Jones DG. Characterization of proteinases in extracts of adult Haemonchus contortus, the ovine abomasal nematode. Parasitology. 1993. 106:395–404.


10. Lourenco EV, Pereira SR, Faca VM, Coelho-Castelo AAM, Mineo JR, Roque-Barreira MC, Greene LJ, Panunto-Castelo A. Toxoplasma gondii micronemal protein MIC1 is a lactose-binding lectin. Glycobiology. 2001. 11:541–547.


11. Newton SE, Meeusen EN. Progress and new technologies for developing vaccines against gastrointestinal nematode parasites of sheep. Parasite Immunol. 2003. 25:283–296.


12. Newlands GFJ, Skuce PJ, Knox DP, Smith WD. Cloning and expression of cystatin, a potent cysteine protease inhibitor from the gut of Haemonchus contortus. Parasitology. 2001. 122:371–378.
13. Pratt D, Armes LG, Hageman R, Reynolds V, Boisvenue RJ, Cox GN. Cloning and sequence comparisons of four distinct cysteine proteases expressed by Haemonchus contortus adult worms. Mol Biochem Parasitol. 1992. 51:209–218.


14. Sajid M, Mckerrow JH, Hansell E, Mathieu MA, Lucas KD, Hsieh I, Greenbaum D, Bogyo M, Salter JP, Lim KC, Franklin C, Kim JH, Caffrey CR. Functional expression and characterization of Schistosoma mansoni cathepsin B and its trans-activation by an endogenous asparaginyl endopeptidase. Mol Biochem Parasitol. 2003. 131:65–75.


15. Sajid M, Mckerrow JH. Cysteine proteases of parasitic organisms. Mol Biochem Parasitol. 2002. 120:1–21.


16. Shompole S, Jasmer DP. Cathepsin B-like cysteine proteases confer intestinal cysteine protease activity in Haemonchus contortus. J Biol Chem. 2001. 276:2928–2934.


17. Skuce PJ, Redmond DL, Liddell S, Stewart EM, Newlands GFJ, Smith WD, Knox DP. Molecular cloning and characterization of gut-derived cysteine proteinases associated with a host protective extract from Haemonchus contortus. Parasitology. 1999. 119:405–412.


18. Stear MJ, Bishop SC, Doligalska M, Duncan JL, Holmes PH, Irvine J, McCririe L, McKeller QA, Sinski E, Murray M. Regulation of egg production, worm burden, worm length and worm fecundity by host responses in sheep infected with Ostertagia circumcincta. Parasite Immunol. 1995. 17:643–652.

