Abstract
The barn owl (BO) and the collared scops owl (CSO) are common nocturnal raptors throughout Thailand. Blood samples from 23 adult BOs and 14 CSOs were collected and processed for complete blood cell counts and parasite morphological examinations. Two Haemoproteus-positive samples were processed for ultrastructural observation. Polymerase chain reaction (PCR) analysis for a partial cytochrome b gene (cytb) from Haemoproteus was performed in all samples. Haemoproteus presence detected by light microscopy was lower than that detected by PCR (30.4% and 34.8%, respectively, in BO; and 50.0% and 78.6%, respectively, in CSO). Comparative hematology revealed that Haemoproteus-positive BOs had higher mean cell hemoglobin concentration, total leukocyte, absolute heterophil, basophil, and monocyte counts than Haemoproteus-negative BOs, but no significant differences between Haemoproteus-negative and -positive CSOs. Monocyte ultrastructure analysis revealed a role in the elimination of gametocytes. Morphologically, the Haemoproteus in 3 BOs and 6 CSOs were identified as H. noctuae, while that in 1 CSO was identified as H. syrnii. Phylogenetic analysis indicated the Haemoproteus spp. in 8 BOs and 7 CSOs were not closely related to H. noctuae or H. syrnii, and the cytb of 2 CSOs was that of H. syrnii. These results should be useful for study of Haemoproteus.
The barn owl (BO, Tyto alba, family Tytonidae) and collared scops owl (CSO, Otus lettia, family Strigidae) are nocturnal raptors of the order Strigiformes and are common in all parts of Thailand [7]. Since 1992, both owl species have been protected under the Wildlife Protection and Preservation Law. BOs were the most common species of raptors submitted to the Kasetsart University Raptor Rehabilitation Unit (KURRU) from 2008 to 2011 [14], and the BO is listed as a near-threatened species in the Red Data of Thailand. Although the hematologic values in BO have been reported [1], little is known about the hemoparasites of these two owl species in Thailand.
Avian blood parasites cause infections of concern in wild birds. Haemoproteus spp., a vector-borne parasite, is transmitted by hippoboscid flies and biting midges [26]. Haemoproteus spp. often does not result in clinical disease; however, hemolytic anemia resulting in severe anemia in Harris hawks [19] and juvenile snowy owls has been reported [20]. These hemoparasites can cause disease in pigeons and quails, but low pathogenicity has been observed in other avian species [5]. Nested cytochrome b (cytb) polymerase chain reaction (PCR) analysis is widely used to detect hemosporidian blood parasites in the peripheral blood of birds [491028], and molecular studies have described a parasite diversity remarkably higher than previously expected [29]. The cytb sequences of only three species of Haemoproteus (H. noctuae, H. syrnii, and H. ilanpapernai [1226]) that infect owls in the order Strigiformes have been reported in the GenBank database (National Center for Biotechnology Information, USA) [41213]. Paperna et al. [21] reported H. noctuae morphometry in brown hawk owls (Ninox scutulata) and collared owlets (Glaucidium brodiei) from Malaysia and H. syrnii morphometry in N. scutulata and spotted wood owls (Strix seloputo) from Singapore. However, there were no reports describing Haemoproteus infections in BO and CSO from Thailand, even in the Asia survey [1121].
The purposes of this study were to determine the hematologic, morphometric, and phylogenetic relationships, as well as the ultrastructural characteristics, of Haemoproteus infecting the BO and CSO submitted to KURRU. The hematozoa infections based on blood smear examination and PCR screening were also compared.
The present study was approved by the Institutional Laboratory Animal Care and Use Committee of Kasetsart University of Thailand (protocol No. ACKU 01560).
Blood samples were collected from 23 adult BOs (10 males and 13 females) and 14 CSOs (6 males and 8 females) at KURRU from May 2013 to February 2016. The owls were clinically healthy, with a good body score, and were prepared for release from KURRU. For sample collection, each owl was physically restrained by using a net, and 1 mL of blood was collected from the jugular or ulnar vein into an ethylenediaminetetraacetic acid (EDTA)-containing tube, and the sample was immediately stored at 4℃.
Blood smears were immediately prepared, air-dried, and stained with Wright's stain (an in-house preparation using Wright eosin methylene blue and Giemsa's azur eosin methylene blue; Merck KGaA, Germany) for grading of blood parasite infection and morphologic evaluation of red blood cells (RBCs). Each smear was examined for 10 min at ×400 magnification to detect blood parasite infection. Infection intensity was estimated as a percentage by counting the number of parasites per 10,000 RBCs at high magnification (×1,000). Leukocyte differential counts were based on an average count of 200 cells by two veterinary hematologists. Thrombocytes per 100 white blood cells (WBCs; expressed as thrombocyte percentage) were also counted.
Complete blood cell counts were obtained by using previously described methods [23]. Briefly, packed cell volumes (PCV) were determined by using a microhematocrit centrifuge at 10,000 × g for 5 min. Total RBC and WBC counts were determined manually by using a counting chamber after the blood had been diluted 200 times with Natt and Herrick's solution, as described previously [523]. A corrected WBC count was calculated by applying the following formula: WBC × 100/(100 + thrombocyte percentage). The hemoglobin concentration was determined by the cyanmethemoglobin method, in which free RBC nuclei were removed by centrifugation before reading the absorbance. The mean cell volume (MCV) and mean cell hemoglobin concentration (MCHC) were calculated from the PCV, hemoglobin concentration, and RBC count. Reticulocyte counts (aggregate and punctate) were determined by staining with new methylene blue and using a wet preparation. Total protein and fibrinogen concentrations were determined by using a refractometer (Atago, Japan) and the heat precipitation method for fibrinogen.
A BX53 light microscope (Olympus, Japan) equipped with a DP73 digital camera and cellSens Standard imaging software (ver. 01.07; Olympus) was used to examine the stained smears. Morphometry (width, length, area, number of pigment granules, and nuclear displacement ratio [NDR] [2]) of 16 to 20 macrogametocytes was undertaken in each owl determined to be positive for Haemoproteus by light microscopy. The NDR was calculated according to the following formula: NDR = 2x/(x + y), where x and y were the width between the nucleus and cell membrane with and without the gametocyte [2]. Morphometry (width, length, area, perimeter, and diameter) of both Haemoproteus-infected and non-Haemoproteus-infected RBCs was performed in 20 to 131 randomly selected RBCs.
The hematological results are presented as mean ± SE values. The Mann-Whitney U test was used to determine the statistical significance of differences between Haemoproteus-negative and -positive owls. Extreme morphometric values were assessed by using scatter plots before exclusion from the data. The data were examined for the presence of a normal distribution by using the Shapiro-Wilk W test and the Kolmogorov-Smirnov test. Descriptive statistics (mean and SD) were used to describe the data. The length and width hematozoa data that had a normal distribution were compared with previously reported data (means and SD) [21] by using a two-sample t-test. The effect of macrogametocytes on RBC parameters was assessed by using one-way analysis of svariance (ANOVA). All statistical analyses were performed by using NCSS 2007 (NCSS, USA).
The EDTA-treated blood samples from 1 BO (KU71) and 1 CSO (KU222) were processed for transmission electron microscopy by using standard methods, as previously described [24]. Briefly, buffy coats were fixed with 2.5% glutaraldehyde, post-fixed in 1% osmium tetroxide, dehydrated in an acetone series, and embedded in Spurr's epoxy resin. Ultrathin sections stained with uranyl acetate and lead citrate were observed by using an HT7700 transmission electron microscope (Hitachi, Japan).
All EDTA blood samples were processed for genomic DNA extraction by using a Blood Genomic DNA Extraction mini-kit (Favorprep, Taiwan). Genomic DNA from each blood sample was analyzed by using nested PCR to amplify a fragment of the cytb gene from Haemoproteus spp., as described previously [610]. Briefly, specific primers designated as HaemNF and HaemNR2 were used for primary PCR amplification by using recombinant Taq DNA polymerase (Invitrogen, USA). The amplified primary PCR product was used as the template for nested PCR. The amplicons were stained with GelStar (Lonza Japan, Japan), and subjected to 1.5% agarose gel electrophoresis. The amplicons (426 base pairs [bp]) were agarose-gel purified by using a Gel/PCR Purification mini-kit (Favorprep) and sequenced by First BASE Laboratories (Malaysia). The nucleotide sequences were analyzed by using BLAST and BioEdit (ver. 7.1.9) [8].
For multiple alignment and phylogenic tree generation, we included 8 BO and 11 CSO sequences and 18 mitochondrial cytb gene sequences of avian Haemoproteus spp. obtained from GenBank. One lineage of Leucocytozoon spp. SPOW2 (EU627825) was used as an out-group. All sequences were aligned and grouped. To estimate evolutionary distances, phylogenetic analysis of 346 bp of the cytb gene was performed by using the neighbor-joining method in the MEGA5 program [25] and the Kimura two-parameter model [15]. To assess tree topology, 1,000 cycles of bootstrap resampling were implemented.
For comparison of amino acid sequences, residues 1259–1373 of the cytb protein were aligned by using Clustal W. Amino acid positions were compared with the complete cytb protein sequence of H. columbae (NC 012448).
Haemoproteus gametocytes were found in blood smears of 7 BOs (30.4%; panels A and B in Fig. 1) and 7 CSOs (50%; panels C–F in Fig. 1). In general, less than 0.1% infected RBCs were found, except for CSO KU222, which had a 2% RBC infection rate (panel E in Fig. 1). Hematology revealed significantly lower MCHC, total leukocyte, absolute heterophil, basophil, and monocyte counts in Haemoproteus-negative than in Haemoproteus-positive BOs (Table 1). In contrast, for CSOs, there were no significant differences detected between the Haemoproteus-negative and -positive owls (Table 2). BOs had higher MCV than those of CSOs (Tables 1 and 2).
The Haemoproteus gametocytes displayed halteridial (almost completely encircling the nucleus of infected RBCs; panels A and C in Fig. 1) or banana (panels B and F in Fig. 1) shapes. Some mature gametocytes (CSO KU181) completely encircled the nuclei of the infected RBCs (circumnuclear; panel D in Fig. 1) but did not laterally displace any nuclei. Extra-erythrocyte rounded gametocytes (panel E in Fig. 1) were frequently observed in all owls except CSO KU127, which showed only banana-shaped gametocytes (panel F in Fig. 1). The cytoplasm of the gametocytes contained grouped or scattered yellow-brown-to dark-pigmented granules (Fig. 1), with different average numbers of granules per parasite (Tables 3 and 4). The Haemoproteus isolates in 3 BOs and 6 CSOs were identified as H. noctuae (Table 3) according to the morphology of the mature gametocytes, which were circumnuclear or halteridial shaped, represented over 90% of the total number mature gametocytes, and had NDR greater than 0.7 average. However, circumnuclear gametocytes were not detected in CSO KU127; although the NDR was greater than 0.7, this isolate was identified as H. syrnii (Table 4).
Ultrastructurally, gametocytes were enclosed in a parasitophorous vacuole bounded by a continuous membrane formed from the plasmalemma of the host cell (panels A and B in Fig. 2). The cavity of the parasitophorous vacuole was not clearly pronounced. Growing gametocytes did not touch the nuclei or cell membrane of the infected RBCs (panels A and B in Fig. 2). The gametocyte cytoplasm contained a nucleus (panels B and C in Fig. 2) and numerous organelles, such as ribosomes (panels B and C in Fig. 2), mitochondria, homogeneous dense or osmiophilic bodies (panels A and B in Fig. 2) and clearly identifiable pigment granules (Fig. 2). Extra-erythrocytic macro- and microgametocytes were delineated (panel C in Fig. 2), and evidence of monocyte-engulfing gametocytes was observed in CSO KU222 (panel D in Fig. 2).
Results of morphometric analysis of the gametocytes in each group of owls were slightly different (Tables 3 and 4). Only the average length of the halteridial-shaped Haemoproteus in 3 BOs was significantly greater than that in 5 CSOs, but they were significantly shorter than that reported for H. noctuae [21] (Table 3). The circumnuclear-shaped gametocytes in 3 BOs also exhibited a significantly shorter length than that reported for H. noctuae [21] (Table 3). The macrogametocytes of Haemoproteus in CSO KU127 were longer but slightly narrower than that in the reference data (Table 4), and molecular study revealed that they were closely related to H. syrnii obtained from spotted wood owls [21] (Figs. 3 and 4).
In BO individuals, H. noctuae halteridial gametocyte-infected RBCs showed significantly greater length, width, and area than those of non-infected RBCs, whereas the nuclei length in infected RBCs was significantly shorter than that in non-infected RBCs (Table 5). The nuclei of circumnuclear gametocyte-infected RBCs were smaller in length and area, but the length and area of the infected RBCs were larger than those of non-infected RBCs (Table 5). The infected nuclei were more condensed than non-infected nuclei (Fig. 1).
In CSOs, H. noctuae-infected RBCs were significantly greater in length and area, but smaller in nuclei length and area of the nucleus than those of non-infected RBCs (Table 5). Only the area of H. syrnii-infected RBCs was significantly lesser than that in non-infected RBCs (Table 5). None of the Haemoproteus gametocytes displaced the host nuclei (NDR > 0.7). The non-infected RBCs in BO had a greater area than those in CSO (Table 5), which correlated with the larger MCV in BO (Tables 1 and 2).
We detected fewer Haemoproteus infections by using light microscopy than by PCR analysis (30.4% vs. 34.8% in BO and 50.0% vs. 78.6% in CSO). Samples from 1 BO and 4 CSOs that were PCR-positive but microscopy-negative were re-examined microscopically by two hematologists screening two entire blood smears from each individual owl. They were all confirmed to be Haemoproteus-negative.
The phylogenic tree of 19 partial cytb gene sequences from 8 BOs and 11 CSOs (Fig. 3) revealed that all partial Haemoproteus cytb genes in this study were grouped in a monophyletic group with a 94% bootstrap value. The Haemoproteus isolates from 8 BOs (KU71, -86, -112, -120, -132, -200, -202, and -203) and 4 CSOs (KU284, -163, -248, and -250) were similar to H. noctuae hCIRCUM01 (KP794612) isolated from Asio otus in Russia (96.2%). The Haemoproteus isolates from 5 CSOs (KU181, -222, -226, -259, and -318) were very similar to H. noctuae hCIRCUM01, with 98% similarity. H. noctuae (KP794612) and H. syrnii (KP794611) formed a small clade, suggesting that they were closely related. Similarly, 2 H. syrnii (KF279523 and EF607209) were observed to be closely related.
The Haemoproteus isolate from KU234 CSO was similar (96.5%) to H. syrnii (EF607290; Fig. 3), whereas the Haemoproteus in KU127 CSO was identical to H. syrnii in tawny owls, Strix aluco, from Russia (KP794611), and was closely related (99.4%) to H. syrnii isolated from Eurasian scops owls, Otus scops, in Bulgaria (KP451480).
Haemoproteus isolates in our study formed one large cluster and were different from those infecting other avian species (isolated from Passeriformes, Suliformes, and Columbiformes birds), which showed that the genetic distance ranged from 3.8% to 13.6%. The Haemoproteus majoris in passerine birds (AF254972 and AF254977) were distant from the studied Haemoproteus, with 93.9% to 96.2% genetic similarity (Fig. 3). However, the types of Haemoproteus described in our study were all more closely related to each other than to H. majoris.
Comparison of amino acid sequences of the cytb protein revealed specific consensus residues for the studied Haemoproteus groups (Fig. 4). Alanine was conserved at residue 1288 of Haemoproteus in KU234 CSO. Methionine was located at residue 1288 of the Haemoproteus isolates from the other 5 CSOs (KU181, -222, -226, -318, and -259). Two isoleucines and 1 threonine were conserved at residues 1264, 1281, and 1282 of the Haemoproteus infecting 8 BOs (KU71, -86, -112, -120, -132, -200, -202, and -203) and 4 CSOs (KU 163, -248, -250, and -284).
To our knowledge, this is the first study to record Haemoproteus in BO and CSO in Thailand based on optical microscopy and molecular studies. The higher MCHC in Haemoproteus-positive BOs may due to the hemolysis of infected RBCs, but this result was not observed in CSOs; that difference might be due to the low number (only 3 owls) of Haemoproteus-negative owls compared to 11 Haemoproteus-positive CSOs. The higher total leukocyte, absolute heterophil, basophil, and monocyte counts in Haemoproteus-positive BOs may be due to active Haemoproteus elimination functions in this species of owl. Haemoproteus is generally considered nonpathogenic in raptors; however, there have been reports of fatalities in owls [22]. The increased leukocyte counts in BO supports the greater pathogenicity of Haemoproteus. The erythrocyte parameters (PCV, MCV, and MCHC) in Haemoproteus-negative BO in the present study were less than those reported from BO (n = 21) in the USA [1], which might be due to different climates and/or different water intake of the owls.
Both halteridial and circumnuclear gametocyte-infected RBCs were larger than non-infected RBCs due to loading of the parasite in the cytoplasm of the RBCs. The reduction in length of nuclei of infected RBCs in both BOs and CSOs may be a pathologic effect in which infected nuclei are more condensed than non-infected nuclei. The lengths of Haemoproteus halteridial gametocytes from CSOs were smaller than those from BOs, although the phylogenetic analysis revealed that they were the same Haemoproteus species. This might be due to the larger size of RBCs in BOs than in CSOs. The shorter width but longer length of H. syrnii gametocytes in KU127 CSO compared to that in spotted wood owls [21] might also be due to a RBC size difference in spotted wood owls, but we have no data on spotted wood owl RBCs to support this hypothesis. The high-resolution images obtained from computerized image analysis yielded better parameter values than those from conventional microscopy. Therefore, the morphometric values of Haemoproteus gametocytes might also be slightly different when obtained by different instruments or from different avian RBCs; regardless, morphological characteristics remain a gold standard for use in species identification of Haemoproteus [26]. We speculated that the identification of parasites via morphometry was difficult in Haemoproteus species.
The greater number of Haemoproteus-positive owls detected by PCR methodology supported the suggestion of a higher sensitivity of molecular techniques compared with that from conventional microscopic examination in low parasitemia cases [28]. However, some same authors [27] and the other authors [18] have reported similar prevalences of hemosporidian parasites obtained from microscopy and PCR analysis. Nonetheless, blood smear examination is relatively inexpensive and provides valuable information. Thus, such examination is still suitable for field studies of parasites [5], comparative analysis [27], and molecular barcoding [29]. Demonstration of gametocytes in thin blood films remains a gold standard method for diagnosis [22] and species classification [326]. PCR-based information should be supported by observation of the blood stage of the parasite [28]. Because the majority of natural Haemoproteus infections are low and are difficult to identify at the species level using only morphological features, the development of new molecular markers is essential for future research [29].
H. syrnii showed the highest infection prevalence (22%) in tawny owl from Germany [17], although in our study H. noctuae was the most prevalent in both BO and CSO. These differences may be due to differences in the geographical distribution of hematozoans. The high prevalence of Haemoproteus-infection in BO and CSO in Thailand supports previous reports indicating that this parasite is the most common hemosporidian found in raptors throughout the world [1722].
Although the ultrastructure of Haemoproteus gametocytes has been relatively well described [26], there are no reports of same for BO and CSO, especially for monocytes phagocytizing gametocytes. Numerous clearly defined micronemes similar to those documented by Valkiūnas [26] were not detected in the Haemoproteus from BO and CSO. The electron density and structure of the osmiophilic bodies were similar to micronemes [26], but we found fewer osmiophilic bodies; although they were easier to identify by using transmission electron microscopy.
Phylogeny branch evidence grouped the Haemoproteus sequences in our study into Parahaemoproteus. The nucleotide sequences were located in the Culicoides spp. transmitted avian hemoprotozoan cluster [4]. Amino acid data were used to confirm the differences between our Haemoproteus isolates and H. noctuae (KP794612). All residues were located from the 1264 to 1288 positions of the cytb protein, and our Haemoproteus sequences displayed unique residues.
The tree topology results showed unique clustering of Haemoproteus from 8 BOs and 4 CSOs. The Haemoproteus isolates exhibited both halteridial-shaped (KU86, 112, 163, 200, 202, 203, 248, 250, and 284) and circumnuclear-shaped (KU71, 120, and 132) mature gametocytes, which were similar to those of H. noctuae but displayed clear differences in molecular characteristics. Therefore, these Haemoproteus may comprise a new species or subspecies of Haemoproteus in strigid owls. Conversely, some species of Haemoproteus, such as H. pallidus, H. minutes, and H. vacuolatus exhibited different morphologies but showed cytb gene genetic divergence of less than 2% [16]. Based on molecular characteristics, Haemoproteus in KU127 CSO (KJ561457) was suggested to be H. syrnii, although they differed in length and width from H. syrnii in spotted wood owls [21]; although it should be H. syrnii based on a morphological characteristic (lacking circumnuclear gametocytes) and phylogenetic analysis (closely related to H. syrnii, KJ541480).
The results of the partial cytb gene analysis revealed that the Haemoproteus spp. of the 8 BOs and 7 CSOs analyzed in this study and the previously reported H. noctuae and H. syrnii formed one large cluster (except KU234 and KU127). Therefore, the Haemoproteus spp. analyzed in this study were closely related to H. noctuae and H. syrnii; moreover, H. noctuae was closely related to H. syrnii. Although the Haemoproteus isolate from KU234 CSO was similar (96.5%) to H. syrnii (EF607290), it was not included in a large cluster, suggesting that this strain was slightly distant from the other large cluster of our study and might be from a different lineage with 3.5% divergence. This result revealed that phylogenetic analysis using short sequences of partial cytb genes has limitations and might not be able to distinguish H. noctuae from H. syrnii correctly. These data provide basic information that will be valuable in future studies of Haemoproteus in BO and CSO.
The results of this study highlight the hematology, morphometry, ultrastructure, and molecular characterization of Haemoproteus in BO and CSO from Thailand and also contribute to identifying the value of microscopy and molecular diagnosis in the study of avian blood parasites. The present study also provides morphological data on the molecular detection of 8 and 11 sequences of Haemoproteus from BO and CSO, respectively, which may be used as the basis for further study of Haemoproteus in these two owl species.
Figures and Tables
Fig. 1
Light micrographs of Haemoproteus noctuae gametocytes from 2 barn owls (A: KU120 and B: KU132) and 2 collared scops owls (C and D: KU181 and E: KU222) and Haemoproteus syrnii gametocytes from KU127 collared scops owl (F). (A and C) Halteridial-shaped microgametocytes. (B) Banana-shaped microgametocyte. (D) Circumnuclear macrogametocyte. (E) Extra-erythrocyte round (arrow) macrogametocyte. (F) Banana-shaped macrogametocyte. Wright's stain. Scale bars = 10 µm (A–F).
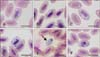
Fig. 2
Transmission electron micrographs of Haemoproteus noctuae gametocytes in KU71 barn owl (A and B) and KU222 collared scops owl (C and D). (A) Circumnuclear gametocyte in red blood cell (RBC) showing parasitophorous vacuole membrane, homogeneous dense or osmiophilic bodies, and pigment granules (arrows). (B) Halteridial gametocyte in RBC showing nucleus and one pigment granule (arrow). Parasitophorous vacuole membrane of growing gametocytes did not touch the nuclei or cell membrane of infected RBC. (C) Free macro- (lower left) and micro- (upper right) gametocytes showing the nucleus and pigment granules (arrows) in microgametocyte. (D) Monocyte containing a phagocytized gametocyte with pigment granules (arrows). The monocyte contained many mitochondria near the phagocytized parasite. Uranyl acetate and lead citrate stains used for all specimens. Scale bars = 1 µm (A, C, and D), 0.5 µm (B). *Nucleus. †Monocyte. ‡Mitochondria.
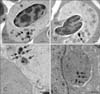
Fig. 3
Phylogenetic tree based on partial cytochrome b gene (346 bp) sequences of Haemoproteus from 8 barn owls (●) and 11 collared scops owls (○) from Thailand. The sequences in bold were obtained in the present study. Numbers on the branches indicate the percent of replicates that reproduced the topology for each clade.
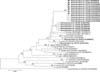
Fig. 4
Comparison of partial amino acid sequences of the cytochrome b (cytb) region inferred from previous reports and our nucleotide sequences obtained from Haemoproteus-infected owls. These amino acids were located on 1259–1373 residues of cytb protein compared with the complete genome of cytb gene of Haemoproteus columbae (NC 012448). Haemoproteus spp. in our study showed specific amino acids (isoleucine at the 1264 and 1281 positions, threonine at the 1282 position, alanine at the 1288 position [only in collared scops owl KU234], and methionine at the 1288 position).
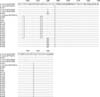
Table 3
Comparative morphometry of macrogametocytes of Haemoproteus noctuae in barn and collared scops owls compared with that reported for H. noctuae in brown hawk owl from Singapore and collared owlet from Malaysia [21]
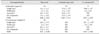
Table 4
Morphometry of Haemoproteus syrnii macrogametocytes in KU127 collared scops owl compared with H. syrnii in spotted wood owl
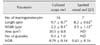
Table 5
Effect of Haemoproteus gametocytes on RBCs and nuclei in barn and collared scops owls
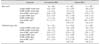
Data are presented as mean ± SD. NDR = 2x/(x + y), where x and y were the width between the nucleus and cell membrane with and without the gametocyte. RBCs, red blood cells; NDR, nuclear displacement ratio. *H. noctuae, halteridial gametocytes. †H. noctuae, circumnuclear gametocytes. ‡H. noctuae. §H. syrnii. abDifferent letters in the same row indicate significantly differences between groups at p < 0.05.
Acknowledgments
The authors acknowledge the Faculty of Veterinary Medicine of Kasetsart University in Thailand and the Kasetsart University Research and Development Institute for funding (grant No. 32.60).
References
1. Ammersbach M, Beaufrère H, Gionet Rollick A, Tully T. Laboratory blood analysis in Strigiformes---Part I: hematologic reference intervals and agreement between manual blood cell counting techniques. Vet Clin Pathol. 2015; 44:94–108.


2. Bennett GF, Campbell AG. Avian Haemoproteidae. I. Description of Haemoproteus fallisi n. sp. and a review of the haemoproteids of the family Turdidae. Can J Zool. 1972; 50:1269–1275.


3. Bennett GF, Peirce MA. Morphological form in the avian Haemoproteidae and an annotated checklist of the genus Haemoproteus Kruse, 1890. J Natural History. 1988; 22:1683–1696.


4. Bukauskaitė D, Žiegytė R, Palinauskas V, Iezhova TA, Dimitrov D, Ilgūnas M, Bernotienė R, Markovets MY, Valkiūnas G. Biting midges (Culicoides, Diptera) transmit Haemoproteus parasites of owls: evidence from sporogony and molecular phylogeny. Parasit Vectors. 2015; 8:303.


5. Campbell T, Ellis C. Hematology of birds. In : Campbell TW, Ellis C, editors. Avian and Exotic Animal Hematology and Cytology. 3rd ed. Oxford: Wiley-Blackwell;2007. p. 3–50.
6. Cosgrove CL, Knowles SC, Day KP, Sheldon BC. No evidence for avian malaria infection during the nestling phase in a passerine bird. J Parasitol. 2006; 92:1302–1304.


7. del Hoyo J, Elliott A, Sargatal J. Handbook of the Birds of the World: Barn-Owls to Hummingbirds. 5. Barcelona: Lynx Edicions;1999. p. 34–158.
8. Hall TA. BioEdit: a user-friendly biological sequence alignment editor and analysis program for Windows 95/98/NT. Nucl Acids Symp Ser. 1999; 41:95–98.
9. Hellgren O, Krizanauskiene A, Valkĭunas G, Bensch S. Diversity and phylogeny of mitochondrial cytochrome b lineages from six morphospecies of avian Haemoproteus (Haemosporida: Haemoproteidae). J Parasitol. 2007; 93:889–896.


10. Hellgren O, Waldenström J, Bensch S. A new PCR assay for simultaneous studies of Leucocytozoon, Plasmodium, and Haemoproteus from avian blood. J Parasitol. 2004; 90:797–802.


11. Ishtiaq F, Gering E, Rappole JH, Rahmani AR, Jhala YV, Dove CJ, Milensky C, Olson SL, Peirce MA, Fleischer RC. Prevalence and diversity of avian hematozoan parasites in Asia: a regional survey. J Wildl Dis. 2007; 43:382–398.


12. Karadjian G, Martinsen E, Duval L, Chavatte JM, Landau I. Haemoproteus ilanpapernai n. sp. (Apicomplexa, Haemoproteidae) in Strix seloputo from Singapore: morphological description and reassignment of molecular data. Parasite. 2014; 21:17.


13. Karadjian G, Puech MP, Duval L, Chavatte JM, Snounou G, Landau I. Haemoproteus syrnii in Strix aluco from France: morphology, stages of sporogony in a hippoboscid fly, molecular characterization and discussion on the identification of Haemoproteus species. Parasite. 2013; 20:32.


14. Kidsin K, Sanyathitiseree P, Pothieng D, Wajjwalku W, Kasorndorkbua C. A retrospective study of morbidity and mortality of raptors in Kasetsart University Raptor Rehabilitation Unit, 2008–2011. Korean J Ornithol. 2012; 19:87–92.
15. Kimura M. A simple method for estimating evolutionary rates of base substitutions through comparative studies of nucleotide sequences. J Mol Evol. 1980; 16:111–120.


16. Krizanauskiene A, Pérez-Tris J, Palinauskas V, Hellgren O, Bensch S, Valkiūnas G. Molecular phylogenetic and morphological analysis of haemosporidian parasites (Haemosporida) in a naturally infected European songbird, the blackcap Sylvia atricapilla, with description of Haemoproteus pallidulus sp. nov. Parasitology. 2010; 137:217–227.


17. Krone O, Priemer J, Streich J, Sömmer P, Langgemach T, Lessow O. Haemosporida of birds of prey and owls from Germany. Acta Protozool. 2001; 40:281–289.
18. Krone O, Waldenström J, Valkiūnas G, Lessow O, Müller K, Iezhova TA, Fickel J, Bensch S. Haemosporidian blood parasites in European birds of prey and owls. J Parasitol. 2008; 94:709–715.


19. Lacasse C. Falconiformes (falcons, hawks, eagles, kites, harriers, bazzards, ospreys, caracaras, secretary birds, old world and new world vultures). In : Miller RE, Fowler ME, editors. Fowler's Zoo and Wild Animal Medicine. St. Louis: Elsevier-Saunders;2012. p. 127–142.
20. Mutlow A, Forbes N. Haemoproteus in raptor: pathogenicity, treatment and control. In : Proceedings of the 21st Annual Conference and Expo of the Association of Avian Veterinarians; 30 August-1 September 2000; Portland, USA. p. 157–163.
21. Paperna I, Keong MSC, May CYA. Haemosporozoan parasites found in birds in Peninsular Malaysia, Singapore, Sarawak and Java. Raffles Bull Zool. 2008; 56:211–243.
22. Remple JD. Intracellular hematozoa of raptors: a review and update. J Avian Med Surg. 2004; 18:75–88.


23. Salakij C, Kasorndorkbua C, Salakij J, Suwannasaeng P, Jakthong P. Quantitative and qualitative morphologic, cytochemical and ultrastructural characteristics of blood cells in the Crested Serpent eagle and Shikra. Jpn J Vet Res. 2015; 63:95–105.
24. Salakij J, Lertwatcharasarakul P, Kasorndorkbua C, Salakij C. Plasmodium circumflexum in a Shikra (Accipiter badius): phylogeny and ultra-structure of the haematozoa. Jpn J Vet Res. 2012; 60:105–109.
25. Tamura K, Peterson D, Peterson N, Stecher G, Nei M, Kumar S. MEGA5: Molecular Evolutionary Genetics Analysis using maximum likelihood, evolutionary distance, and maximum parsimony methods. Mol Biol Evol. 2011; 28:2731–2739.


26. Valkiūnas G. Avian Malaria Parasites and Other Haemosporidia. Boca Raton: CRC Press;2005. p. 1–268.
27. Valkiūnas G, Iezhova TA, Krizanauskiene A, Palinauskas V, Sehgal RN, Bensch S. A comparative analysis of microscopy and PCR-based detection methods for blood parasites. J Parasitol. 2008; 94:1395–1401.


28. Valkiūnas G, Iezhova TA, Loiseau C, Sehgal RN. Nested cytochrome b polymerase chain reaction diagnostics detect sporozoites of hemosporidian parasites in peripheral blood of naturally infected birds. J Parasitol. 2009; 95:1512–1515.


29. Valkiūnas G, Palinauskas V, Ilgūnas M, Bukauskaitė D, Dimitrov D, Bernotienė R, Zehtindjiev P, Ilieva M, Iezhova TA. Molecular characterization of five widespread avian haemosporidian parasites (Haemosporida), with perspectives on the PCR-based detection of haemosporidians in wildlife. Parasitol Res. 2014; 113:2251–2263.

