Abstract
Paratuberculosis (PTB) is caused by Mycobacterium avium subsp. paratuberculosis (MAP) and is one of the most widespread and economically important diseases in cattle. After birth, calves are raised with natural breast feeding without separation from their mothers in most Korean native cattle (Hanwoo breed) farms. Vertical transmission of PTB has been reported, but the exact PTB infection route has not been revealed in Hanwoo farms. Calves of MAP seropositive dams were tested for MAP presence and MAP antibodies in feces and tissues. MAP was detected in calf tissues by using polymerase chain reaction. Expressions of genes reported to be prognostic biomarkers of MAP infection changed in both calves and cows (p < 0.05). Expression of two genes (HGF and SERPINE1) were significantly decreased in MAP-infected cattle and their offspring (p < 0.01). The results suggest that biomarker gene expression profiles can be useful in detecting early stage MAP infection. Based on the results, complete eradication of MAP may be possible if accurate diagnostic methods to detect infected calves are added to the current PTB eradication strategy, which, because infected individuals are likely to develop into fecal MAP shedders at any time, includes isolation of new born calves and feeding sterilized colostrum.
Johne's disease, also called paratuberculosis (PTB), is caused by Mycobacterium avium subsp. paratuberculosis (MAP) and is one of the most widespread and economically important diseases in cattle [23]. Because MAP is a slow-growing bacteria with a very long incubation period, the clinical signs of PTB may not be observed for more than two years after initial infection [32]. Therefore, MAP-infected animals usually have a prolonged subclinical stage of infection. However, such animals can be a source of infection because they can contaminate the surrounding environment by shedding MAP bacteria through their feces during their subclinical stage of infection. Animals can be easily infected by ingestion of MAP-contaminated environmental components such as feed, water, and bedding [33]. Therefore, the primary PTB control strategy is reduction of the number of cattle shedding MAP through their feces [20]. Although this strategy has reduced the prevalence of MAP infection, it has not generally eliminated MAP infection. Moreover, general diagnostic methods such as enzyme-linked immunosorbent assay (ELISA), fecal polymerase chain reaction (PCR), and fecal culture often fail to identify the subclinical stage of infection due to variations in PTB progression and MAP shedding, resulting in intermittent detection [21].
Vertical transmission is another cause of difficulty in the eradication of PTB. Vertical transmission is the acquisition of infection by new born calves from dams and can be divided into prenatal and postnatal infections. MAP is reported to be capable of producing a prenatal infection with evidence supporting that capability including the presence of MAP in placenta or sex organs [33]. Postnatal infection occurs mainly by exposure to bacteria present in colostrum or fecal material. In the calf, the highest susceptibility to MAP occurs during the first day of life (within 24 h after birth) [3240]. Therefore, a key element of PTB eradication strategies, so far, has been periodic MAP screening of farms, isolation of calves from dams right after parturition, and the provision of sterilized colostrum [3033].
However, in the Korean native cattle (Hanwoo breed) industry, most farms use a conventional breeding system, which involves feeding calves through natural breast milking without dam separation for a period of 3 to 4 months. Thus, the calves live in same herd as their mother until weaning. Many previous studies have warned that rearing the calf with the dam serves as an intervening factor in infection [3263036]. Current rearing systems have had these problems pointed out in previous studies; regardless, there is a need to investigate the risk of vertical transmission in the current Korean Hanwoo rearing system. In addition, studies on the transmission of MAP infections in Korean Hanwoo farms have not been conducted in Korea.
A biomarker can be used as an indicator of normal biological states, pathogenic conditions, or therapeutic responses to treatment [31]. In that regard, biomarkers have been used for the diagnosis of diseases such as cancers, metabolic diseases, autoimmune diseases, and infectious diseases [1015]. In particular, host biomarkers such as transcripts, microRNA (miRNA), and metabolites have been proposed as diagnostic indicators of chronic infectious diseases in humans and animals [911]. In recent years, transcriptomics and proteomics have been used to analyze the expression of genes and proteins in attempts to identify biomarkers of early stage MAP infection [131819]. In our previous microarray method-based studies of cells and animal models, several biomarkers for diagnosis of early stage of PTB were proposed [242829].
In this study, we used several diagnostic tools to confirm the MAP infection status of calves immediately after weaning in order to determine the degree of infection transmitted to calves born from dams that were positive for MAP in the conventional Korean Hanwoo rearing system. To that end, we analyzed differences in expression levels of several biomarkers selected from our previous study to identify whether MAP-infected calves could be successfully diagnosed.
About 1,000 Hanwoo cattle were raised in a Korean native cattle farm located in a mountainous area at an approximate 800 m altitude. The cattle were put out to pasture except during the winter season. Fifteen Hanwoo cows previously diagnosed as positive for PTB by using a commercial ELISA kit (IDEXX Laboratories, USA) and 8 calves borne from them were included in the study (Table 1). Control animals (n = 5), 2 to 6 years old, were selected from other farms and diagnosed as negative for PTB according to results of serum ELISA and fecal PCR testing performed 4 times within a 6-month interval. Age of the Hanwoo cows varied from 2 to 10 years and their parity ranged between 0 and 7. None of the seropositive cows exhibited any of the typical clinical signs of MAP infection. The calves were raised at the same farm with natural breast feeding from their mothers until the age of 4 to 5 months.
Infection status of cows and calves were analyzed by using commercial ELISA and fecal PCR assays even though the cows were identified as seropositive. In addition, detection of MAP was carried out in mesenteric lymph node, ileum, and Peyer's patch of calves by using PCR and acid-fast staining. For fecal PCR, fecal samples were collected individually from rectum. Collected samples were subjected to DNA extraction carried out by using the mGITC/SC method [25]. For downstream analysis, IS900 real-time PCR and ISMAP02 nested PCR were conducted to identify specific genomic materials of MAP. Both of those PCR methods were performed by using previously described methods [1627], with a slight modification in the nested PCR. The PCR reaction mixture for nested PCR was set up in a volume of 20 µL, using 1 µL of template DNA, nuclease-free water, 2 µL of i-Taq 10× PCR buffer (Intron Biotechnology, Korea), 2.5 mM MgCl2, 0.25 mM deoxyribonucleotide triphosphates, 500 nM of each primer, and 2.5 U i-Taq DNA polymerase. Samples were run according to the following protocol: initial denaturation at 94℃ for 5 min, 35 cycles of 94℃ for 15 sec, 58℃ for 15 sec, and 72℃ for 20 sec, followed by final extension at 72℃ for 7 min. For the second step of the nested PCR, 1 µL of amplicon was used as the template and the reaction run according to the same conditions as the first step PCR, but with the number of cycles reduced from 35 to 30. Fresh tissues (mesenteric lymph node, Peyer's patch, and ileum) were obtained during necropsy of the eight calves. One gram of each tissue sample was prepared for DNA extraction. The samples were homogenized by mortar and pestle and, except for large particles, suspended in 1× phosphate buffered saline. Suspended samples were then vortexed and centrifuged at 13,000 × g for 2 min. Pellets were re-suspended in L6 lysis buffer followed by vortexing and incubation in a heat block at 95℃ for 15 min. The remaining steps were the same as described in the mGITC/SC method [25]. Tissue DNA was then analyzed by performing IS900 real-time PCR and ISMAP02 nested PCR. Histopathological examination and acid-fast staining were performed as previously described [28].
Seven genes identified as prognostic biomarkers for MAP infection in previous research [5242829] were selected for use in this study. From whole blood collected from the jugular vein, total RNA was extracted by using a FavorPrep Blood/Cultured Cell Total RNA Mini kit (Favorgen Biotech Corporation, Taiwan) as previously described [24]. The extracted RNA was stored at −80℃ until use. Expression levels of the genes were analyzed by quantitative real-time RT-PCR using the QuantiTect Reverse Transcription kit (Qiagen, USA) according to the manufacturer's instructions. In brief, 100 ng of total RNA was mixed with 7× gDNA Wipe Out buffer and incubated at 42℃ for 2 min. After incubation, the mixture was mixed with reverse transcriptase and RT primer mix. The reverse transcription reaction was performed by incubating the samples at 42℃ for 15 min with the reaction stopped by incubation at 95℃ for 3 min. Quantitative real-time RT-PCR reactions were performed with 1 µL of cDNA using the Rotor-Gene SYBR Green PCR kit (Qiagen) and the Rotor-Gene Q real time PCR cycler (Qiagen). Amplification was conducted by subjecting the samples to 40 cycles of 95℃ for 15 sec followed by 45 sec at 60℃. Expression levels were determined by applying the 2−ΔΔCt method with housekeeping gene β-actin as the reference. Relative gene expression level was compared with the control group to determine the fold changes in the expression of each gene.
Serological diagnosis using commercial ELISA revealed two cows were positive and four cows were suspect for MAP infection (Table 1) even though all cows were seropositive in previous diagnoses. Based on ELISA, all calves were seronegative. Fecal MAP detection results were negative, except for two cows (Table 1; cows 1 and 9) that were ISMAP02 positive. The IS900 gene was detected in the tissues from all calves. In addition, tissue of one calf (Table 1; calf 8-1) was positive for both IS900 and ISMAP02. Acid-fast bacilli were not detected in formalin-fixed tissue samples, and none of the samples showed histopathological indications such as granulomatous lesion or immune cell infiltration.
Two genes (KLRB1 and FGF2) were up-regulated and five genes (S100A9, HGF, TIMP1, LTF, and SERPINE1) were down-regulated in the cow and calf groups. Of the upregulated genes, FGF2 showed the greatest expression change in the cow group (0.57 fold up-regulation) and KLRB1 showed the greatest expression change in the calf group (1.43 fold up-regulation). Five genes (HGF, S100A9, TIMP1, LTF, and SERPINE1) were down-regulated in the cow and calf groups. Of the downregulated genes, HGF showed the greatest expression change in the cow group (4.35 fold downregulation) and SERPINE1 showed the greatest expression change in the calf group (4.52 fold down-regulation). Of these seven genes, two genes (HGF and SERPINE1) showed significant expression changes in both cows and calves and four genes (KLRB1, S100A9, LTF, and TIMP1) only showed significant expression changes in calves (Fig. 1).
Fifteen cows previously diagnosed as MAP positive and their eight calves had their MAP infection status investigated to determine whether the infection had spread from dam to calf. Evidence of infection in the calves was obtained from tissue samples by using tissue-based PCR targeting the same MAP specific genes as those assessed via fecal-based PCR. The tissue PCR results revealed one calf to have positive PCR results for both IS900 and ISMAP02, whereas the remaining seven calves only showed positive tissue PCR results for IS900. Although this single double positive might be the result of the calf being infected with other mycobacteria with IS900-like sequences [7], it could also have occurred because the number of MAP was very low in the tissue sample or due to differences in sensitivity between the IS900- and ISMAP02-targeting PCR. In the current study, the Ct values of the IS900 real-time PCR in calves were very high (34.95–41.27), indicating that MAP were present at very low levels in the tested tissues [25]. A similar phenomenon was observed in the cows' fecal PCR results in which two cows were positive in ISMAP02 nested PCR and the remainders were negative in both IS900 real-time PCR and ISMAP02 nested PCR. Taken together, the results indicate that 100% of the calves were infected with MAP, even though the cows did not excrete the bacteria through their feces or excreted only a very small amount of bacteria during the period up to weaning. Commercial ELISA results in the tested cows revealed only two cows were MAP positive, four cows had doubtful results, and the remaining nine were MAP negative. It is possible that ELISA produced false-negative results because all cows had previously shown positive antibody responses against MAP when the same ELISA was used. False-negative results are common in cattle when they are in silent or subclinical PTB stages particularly in calves, heifers, and first lactation cows [33]. However, except for the positive results in some older cattle, most of the results were not related to age, which seems to be related to the sensitivity of the current ELISA. Sensitivity of commercial ELISA in cattle with subclinical PTB has differed according to farm conditions (e.g., herd PTB prevalence, presence of high MAP shedders) [37]. Test sensitivity of serum ELISA was estimated to be only 12% to 15% in low PTB prevalence herds and/or in low MAP shedders [81734]. Fecal MAP shedding of all cattle on the study farm was not tested, but the fecal PCR results from the tested animals indicated low or no MAP shedding. Longitudinal studies have also reported MAP detection fluctuations in ELISA results [35]. Those authors proposed the possibility that these phenomena could be divided into false-positive results, false-negative results, and actual fluctuations in antibody production. Fecal PCR and ELISA results of the dams in this study showed that MAP infection could be transmitted to the offspring even if PTB in the dams had not progressed very far.
Limitation of this study is that there was no information on MAP infection status of dams at parturition even though it has been often stated that calves are most susceptible to MAP in the first day of life. However, it is difficult to distinguish between prenatal infection and first day exposure infection, even if the condition of the dam at parturition is known. Based on the observation that the first day after birth is the time of greatest susceptibility, it is inferred that the MAP is present in the environment of the pen, in colostrum supplied from the mother, or has been transmitted in utero. Since the farm on which the experiment was conducted is large and the pens for parturition are isolated, it is highly likely that the infection was transmitted from the mother with the route of infection being in utero or oral. The important consideration is that calves born from infected cows have 100% MAP detection in their tissues. In another study, calves born from an infected mother showed about 30% bacterial shedding in the heifer state, regardless of the infection condition at the time of parturition [12]. However, as in this study, it is expected that almost all calves born from infected dams have MAP infections, but many of them may remove the bacteria through immune system responses, or they may proceed to a silent stage of infection, which is difficult to diagnose with current diagnostic tools. More data derived from calf tissue samples are required to investigate this hypothesis.
MAP infections have been observed in 3- to 4-month-old calves during their weaning period, and these infected individuals are likely to develop into potential fecal MAP shedders at any time; however, complete eradication of MAP may still be possible if accurate diagnostic methods for the detection of infected calves in this period have been added to the current PTB eradication strategy that includes isolating new born calves and feeding them sterilized colostrum.
In this study, several prognostic biomarkers established from our previous study were applied to dams and calves to confirm whether early detection of MAP infection is possible. Current MAP diagnostic techniques are insufficient to detect subclinical infections due to low assay sensitivity, especially in young animals [4]. PCR-based detection has poor sensitivity in clinical samples and is not recommended as a screening tool for direct detection [38]. Similarly, ELISA is considered inadequate for diagnosis of animals with subclinical infections due to poor assay sensitivity [39]. Therefore, a diagnostic method that uses genetic biomarkers can be considered as an effective alternative for the early detection of MAP infection.
In this study, gene expression profiles of biomarkers that were differentially expressed in MAP-infected animals in previous studies [5242829] were analyzed. Three genes (S100A9, LTF, and TIMP1) were significantly down-regulated in the calf group and one gene (KLRB1) was significantly up-regulated in the cow group. These conflicting results between the calf and cow groups may be explained by differences in the immune systems of young and adult animals. Gene expression of biomarkers varied in the infected animals, and expressions of two genes (HGF and SERPINE1) were significantly decreased in both of MAP-infected cattle and their offspring (p < 0.01). Hepatocyte growth factor (HGF) is a multifunctional protein that modulates immune responses [22], and has been shown to inhibit the pro-inflammatory immune response and enhance the anti-inflammatory immune response [6]. Significant down-regulation of HGF could be related to an acute immune response in early stage MAP infections. SERPINE1 is a key element of the fibrinolytic system [14], and it induces apoptosis of injured cell [2]. A previous study showed anti-apoptotic responses induced in various strains of bovine macrophage that are infected with MAP [1]. Significant down-regulation of SERPINE1 seems to reflect an insufficient innate immune response against MAP infection. Taken together, these observations suggest that examination of biomarker gene expression profiles might be useful in the detection of early stage MAP infections. In this study, the risk of transmission of MAP infection from cow to calf and the possibility of using biomarkers as a new diagnostic tool have been described. The results show that culling of infected cattle is the primary concern in preventing the transmission of MAP infection to calves. In conclusion, it is crucial to develop a novel method based on using biomarker data that will provide MAP infection diagnoses that are more accurate and more sensitive than those provided by existing methods.
Figures and Tables
Fig. 1
Gene expression profiles of cows and calves infected with Mycobacterium avium subsp. paratuberculosis compared to those of non-infected controls. Expression levels were normalized by using the 2−ΔΔCT method relative to the beta-actin expression level and compared to the control group. Fold changes are presented as log2 ratios. *p < 0.05, **p < 0.01.
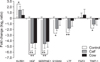
Acknowledgments
This work was carried out with the support of the Cooperative Research Program for Agriculture Science & Technology Development (project No. PJ00897001) Rural Development Administration, the BK21 PLUS Program for Creative Veterinary Science Research, and the Research Institute for Veterinary Science, Seoul National University, Korea.
References
1. Abendaño N, Juste RA, Alonso-Hearn M. Anti-inflammatory and antiapoptotic responses to infection: a common denominator of human and bovine macrophages infected with Mycobacterium avium subsp. paratuberculosis. Biomed Res Int. 2013; 2013:908348.
2. Al-Fakhri N, Chavakis T, Schmidt-Wöll T, Huang B, Cherian SM, Bobryshev YV, Lord RS, Katz N, Preissner KT. Induction of apoptosis in vascular cells by plasminogen activator inhibitor-1 and high molecular weight kininogen correlates with their anti-adhesive properties. Biol Chem. 2003; 384:423–435.


3. Aly SS, Thurmond MC. Evaluation of Mycobacterium avium subsp paratuberculosis infection of dairy cows attributable to infection status of the dam. J Am Vet Med Assoc. 2005; 227:450–454.


4. Britton LE, Cassidy JP, O'Donovan J, Gordon SV, Markey B. Potential application of emerging diagnostic techniques to the diagnosis of bovine Johne's disease (paratuberculosis). Vet J. 2016; 209:32–39.


5. Cha SB, Yoo A, Park HT, Sung KY, Shin MK, Yoo HS. Analysis of transcriptional profiles to discover biomarker candidates in Mycobacterium avium subsp. paratuberculosis-infected macrophages, RAW 264.7. J Microbiol Biotechnol. 2013; 23:1167–1175.


6. Coudriet GM, He J, Trucco M, Mars WM, Piganelli JD. Hepatocyte growth factor modulates interleukin-6 production in bone marrow derived macrophages: implications for inflammatory mediated diseases. PLoS One. 2010; 5:e15384.


7. Cousins DV, Whittington R, Marsh I, Masters A, Evans RJ, Kluver P. Mycobacteria distenct from Mycobacterium avium subsp. paratuberculosis isolated from the faeces of ruminants possess IS900-like sequences detectable IS900 polymerase chain reaction: implications for diagnosis. Mol Cell Probes. 1999; 13:431–442.


8. Dargatz DA, Byrum BA, Barber LK, Sweeney RW, Whitlock RH, Shulaw WP, Jacobson RH, Stabel JR. Evaluation of a commercial ELISA for diagnosis of paratuberculosis in cattle. J Am Vet Med Assoc. 2001; 218:1163–1166.


9. De Buck J, Shaykhutdinov R, Barkema HW, Vogel HJ. Metabolomic profiling in cattle experimentally infected with Mycobacterium avium subsp. paratuberculosis. PLoS One. 2014; 9:e111872.
10. de Gonzalo-Calvo D, Kenneweg F, Bang C, Toro R, van der Meer RW, Rijzewijk LJ, Smit JW, Lamb HJ, Llorente-Cortes V, Thum T. Circulating long-non coding RNAs as biomarkers of left ventricular diastolic function and remodelling in patients with well-controlled type 2 diabetes. Sci Rep. 2016; 6:37354.


11. Dehnad A, Ravindran R, Subbian S, Khan IH. Development of immune-biomarkers of pulmonary tuberculosis in a rabbit model. Tuberculosis (Edinb). 2016; 101:1–7.


12. Eisenberg SW, Rutten VP, Koets AP. Dam Mycobacterium avium subspecies paratuberculosis (MAP) infection status does not predetermine calves for future shedding when raised in a contaminated environment: a cohort study. Vet Res. 2015; 46:70.
13. Farrell D, Shaughnessy RG, Britton L, MacHugh DE, Markey B, Gordon SV. The identification of circulating MiRNA in bovine serum and their potential as novel biomarkers of early Mycobacterium avium subsp paratuberculosis infection. PLoS One. 2015; 10:e0134310.
15. Hsieh SC, Tsai CY, Yu CL. Potential serum and urine biomarkers in patients with lupus nephritis and the unsolved problems. Open Access Rheumatol. 2016; 8:81–91.
16. Irenge LM, Walravens K, Govaerts M, Godfroid J, Rosseels V, Huygen K, Gala JL. Development and validation of a triplex real-time PCR for rapid detection and specific identification of M. avium sub sp. paratuberculosis in faecal samples. Vet Microbiol. 2009; 136:166–172.


17. Kalis CH, Barkema HW, Hesselink JW, van Maanen C, Collins MT. Evaluation of two absorbed enzyme-linked immunosorbent assays and a complement fixation test as replacements for fecal culture in the detection of cows shedding Mycobacterium avium subspecies paratuberculosis. J Vet Diagn Invest. 2002; 14:219–224.


18. Lamont EA, Janagama HK, Ribeiro-Lima J, Vulchanova L, Seth M, Yang M, Kurmi K, Waters WR, Thacker T, Sreevatsan S. Circulating Mycobacterium bovis peptides and host response proteins as biomarkers for unambiguous detection of subclinical infection. J Clin Microbiol. 2014; 52:536–543.


19. Malvisi M, Palazzo F, Morandi N, Lazzari B, Williams JL, Pagnacco G, Minozzi G. Responses of bovine innate immunity to Mycobacterium avium subsp. paratuberculosis infection revealed by changes in gene expression and levels of microRNA. PLoS One. 2016; 11:e0164461.
20. McKenna SL, Keefe GP, Tiwari A, VanLeeuwen J, Barkema HW. Johne's disease in Canada part II: disease impacts, risk factors, and control programs for dairy producers. Can Vet J. 2006; 47:1089–1099.
21. Merkal RS, Thurston JR. Comparison of Mycobacterium paratuberculosis and other mycobacteria, using standard cytochemical tests. Am J Vet Res. 1966; 27:519–521.
22. Molnarfi N, Benkhoucha M, Funakoshi H, Nakamura T, Lalive PH. Hepatocyte growth factor: a regulator of inflammation and autoimmunity. Autoimmun Rev. 2015; 14:293–303.


23. Ott SL, Wells SJ, Wagner BA. Herd-level economic losses associated with Johne's disease on US dairy operations. Prev Vet Med. 1999; 40:179–192.


24. Park HE, Shin MK, Park HT, Jung M, Cho YI, Yoo HS. Gene expression profiles of putative biomarker candidates in Mycobacterium avium subsp. paratuberculosis-infected cattle. Pathog Dis. 2016; 74:ftw022.
25. Park HT, Shin MK, Sung KY, Park HE, Cho YI, Yoo HS. Effective DNA extraction method to improve detection of Mycobacterium avium subsp. paratuberculosis in bovine feces. Korean J Vet Res. 2014; 54:55–57.


26. Pithua P, Godden SM, Wells SJ, Oakes MJ. Efficacy of feeding plasma-derived commercial colostrum replacer for the prevention of transmission of Mycobacterium avium subsp paratuberculosis in Holstein calves. J Am Vet Med Assoc. 2009; 234:1167–1176.


27. Ravva SV, Stanker LH. Real-time quantitative PCR detection of Mycobacterium avium subsp. paratuberculosis and differentiation from other mycobacteria using SYBR Green and TaqMan assays. J Microbiol Methods. 2005; 63:305–317.


28. Shin MK, Park H, Shin SW, Jung M, Lee SH, Kim DY, Yoo HS. Host transcriptional profiles and immunopathologic response following Mycobacterium avium subsp. paratuberculosis infection in mice. PLoS One. 2015; 10:e0138770.
29. Shin MK, Park HT, Shin SW, Jung M, Im YB, Park HE, Cho YI, Yoo HS. Whole-blood gene-expression profiles of cows infected with Mycobacterium avium subsp. paratuberculosis reveal changes in immune response and lipid metabolism. J Microbiol Biotechnol. 2015; 25:255–267.


30. Stabel JR. Pasteurization of colostrum reduces the incidence of paratuberculosis in neonatal dairy calves. J Dairy Sci. 2008; 91:3600–3606.


32. Sweeney RW. Pathogenesis of paratuberculosis. Vet Clin North Am Food Anim Pract. 2011; 27:537–546. v


33. Sweeney RW. Transmission of paratuberculosis. Vet Clin North Am Food Anim Pract. 1996; 12:305–312.


34. Sweeney RW, Whitlock RH, Buckley CL, Spencer PA. Evaluation of a commercial enzyme-linked immunosorbent assay for the diagnosis of paratuberculosis in dairy cattle. J Vet Diagn Invest. 1995; 7:488–493.


35. Sweeney RW, Whitlock RH, McAdams S, Fyock T. Longitudinal study of ELISA seroreactivity to Mycobacterium avium subsp. paratuberculosis in infected cattle and culture-negative herd mates. J Vet Diagn Invest. 2006; 18:2–6.


36. Sweeney RW, Whitlock RH, Rosenberger AE. Mycobacterium paratuberculosis isolated from fetuses of infected cows not manifesting signs of the disease. Am J Vet Res. 1992; 53:477–480.
37. Tiwari A, VanLeeuwen JA, McKenna SL, Keefe GP, Barkema HW. Johne's disease in Canada part I: clinical symptoms, pathophysiology, diagnosis, and prevalence in dairy herds. Can Vet J. 2006; 47:874–882.
38. Wells SJ, Collins MT, Faaberg KS, Wees C, Tavornpanich S, Petrini KR, Collins JE, Cernicchiaro N, Whitlock RH. Evaluation of a rapid fecal PCR test for detection of Mycobacterium avium subsp. paratuberculosis in dairy cattle. Clin Vaccine Immunol. 2006; 13:1125–1130.

