Abstract
Ginseng gintonin is an exogenous ligand of lysophosphatidic acid (LPA) receptors. Accumulating evidence shows LPA helps in rapid recovery of corneal damage. The aim of this study was to evaluate the therapeutic efficacy of gintonin in a rabbit model of corneal damage. We investigated the signal transduction pathway of gintonin in human corneal epithelium (HCE) cells to elucidate the underlying molecular mechanism. We next evaluated the therapeutic effects of gintonin, using a rabbit model of corneal damage, by undertaking histochemical analysis. Treatment of gintonin to HCE cells induced transient increases of [Ca2+]i in concentration-dependent and reversible manners. Gintonin-mediated mobilization of [Ca2+]i was attenuated by LPA1/3 receptor antagonist Ki16425, phospholipase C inhibitor U73122, inositol 1,4,5-triphosphate receptor antagonist 2-APB, and intracellular Ca2+ chelator BAPTA-AM. Gintonin facilitated in vitro wound healing in a concentration-dependent manner. When applied as an eye-drop to rabbits with corneal damage, gintonin rapidly promoted recovery. Histochemical analysis showed gintonin decreased corneal apoptosis and increased corneal cell proliferation. We demonstrated that LPA receptor activation by gintonin is linked to in vitro and in vivo therapeutic effects against corneal damage. Gintonin can be applied as a clinical agent for the rapid healing of corneal damage.
Ginseng has diverse physiological and pharmacological effects as a tonic [13]. There is much evidence to show that in vitro or ex vivo treatments with a ginseng extract can promote skin wound healing. For example, Pazyar et al. [23] reported that ginseng extract improves the healing of experimentally induced skin wounds and burns. In addition, ginseng extract is known to increase epidermal cell proliferation [17], although the exact underlying molecular mechanism and ingredients active in ginseng extract-induced wound healing are relatively undescribed. In contrast, lysophosphatidic acid (LPA), a well-characterized phospholipid, is known as a lipid-derived growth factor and a mediator of wound healing [29]. LPA exerts its wound-healing effects through its G-protein-coupled LPA receptor [31]. In the peripheral system, LPA is involved in the healing of skin wounds and the restoration of epithelial damage in the gastrointestinal system by stimulating cell migration and proliferation [26]. Furthermore, recent studies have shown that damaged corneas exhibit increased secretions of LPA, which could be involved in corneal wound healing [27].
Ginseng plants contain a specific form of LPA called gintonin, which differs from the LPA produced in animals [111322]. Being both a ginseng protein and an LPA complex, gintonin consists of LPA C18:2, LPA C16:0, LPA C18:1, and ginseng proteins [11]. Gintonin consists of about 9.5% LPAs and induces [Ca2+]i transients through plasma membrane LPA receptor signal transduction with high affinity [11]. In addition, gintonin regulates various Ca2+-dependent ion channels and receptors [625]. Other studies have shown that gintonin-induced mobilization of [Ca2+]i is linked to neurotransmitter release and cell proliferation [9]. In addition, previous reports have suggested that gintonin mediates increases in cytosolic free Ca2+, and the ensuing intra- and intercellular communications via ion channels and receptors might be related to the therapeutic effects of gintonin; however, it is unknown whether the activation of LPA receptors by gintonin is directly linked to its therapeutic effects.
The corneal epithelium of eyes in animals is exposed to various environmental and artificial threats, including elective eye surgery in humans, that result in corneal wounds [18]. Rapid corneal wound healing is necessary for maintaining a healthy eye. Although endogenous LPA participates in corneal wound healing processes, the ability of exogenous LPAs, such as those in gintonin, to cure corneal wounds have not been directly demonstrated. In the present study, we used human corneal epithelium (HCE) cells to determine the in vitro molecular mechanism of corneal wound healing and used a rabbit model to obtain in vivo therapeutic information on that process. We found that gintonin induces large increases in cytosolic free Ca2+ in HCE cells via LPA receptor signal transduction pathways. Moreover, gintonin increased phosphorylation of extracellular signal-regulated kinase (ERK) and increased cell migration and proliferation. In the in vivo model, gintonin promoted rapid corneal wound healing. In this article, we discuss the pharmacological mechanisms underlying gintonin-mediated corneal wound healing and show that gintonin can be used as a specifically targeted therapeutic agent for corneal damage.
The edible gintonin fraction was prepared from Panax ginseng by applying a previously described method [7]. Gintonin was dissolved in saline and diluted with medium before use. RPMI 1640 medium, DMEM, fetal bovine serum (FBS), horse serum (HS), penicillin, and streptomycin were obtained from Invitrogen (USA). LPA (1-oleoyl-2-hydroxy-sn-glycero-3-phosphate; 857130P) was obtained from Avanti Polar Lipids (Alabaster, USA). All other reagents used in the experiments, including BAPTA-AM and N-2-hydroxyethylpiperazine-N′-2-ethanesulfonic acid (HEPES), were obtained from Sigma-Aldrich (USA).
Male rabbits (New Zealand White, 8 weeks) were purchased from Koatech (Korea). The rabbits were housed one per cage, allowed access to water and food ad libitum, and maintained under constant temperature (23℃ ± 1℃) and humidity (55% ± 5%) under a 12 h light/dark cycle (lights on 07:00 to 19:00 h). All experiments in this study were conducted in accordance with the Guide for the Care and Use of Laboratory Animals by the National Institutes of Health. The study protocol was approved by Konkuk University (permit No. 13-187).
Cytosolic calcium concentration was determined by performing dual excitation spectrofluorometric analysis of cell suspensions loaded with Fura-2 AM (Sigma-Aldrich, USA) as previously described [12]. Briefly, HCE cells (either untreated or treated with nerve growth factor) were harvested with trypsin/EDTA solution and resuspended in HEPES-buffered saline solution (HBS; 120 mM NaCl, 5 mM KCl, 1 mM MgCl2, 1.5 mM CaCl2, 10 mM glucose, 25 mM HEPES, pH 7.4). The cells were incubated with Fura-2 AM (final concentration 2.5 µM) in HBS at 37℃ for 30 min. Centrifugation was used to remove extracellular Fura-2 AM. Each aliquot of 3 × 106 cells was put into a cuvette, and cytosolic calcium mobilization was measured by using a RF-5301PC spectrofluorophotometer and Supercap software (excitation wavelengths, 340 nm and 380 nm; emission wavelength, 520 nm; Shimadzu, Japan).
HCE cells were washed with cold phosphate-buffered saline (PBS), collected, and lysed with buffer (20 mM Tris-HCl, pH 7.5, 2 mM EDTA, 5 mM dithiothreitol, 0.32 M sucrose, and a protease inhibitor cocktail) to obtain membrane fractions. The HCE cell lysates were centrifuged at 12,000 × g for 30 min at 4℃; the pellets were resuspended in the same lysis buffer (1% Triton X-100, incubated on ice for 45 min) and centrifuged again at 12,000 × g for 30 min at 4℃. The supernatants were collected and used as membrane fractions. Total cell protein was measured by the BCA method (Thermo Scientific, USA). HCE cell membrane fractions were separated by performing sodium dodecyl sulfate polyacrylamide gel electrophoresis (SDS-PAGE) on 10% gels and then transferred to polyvinylidene difluoride membranes. After that, horseradish peroxidase-conjugated goat anti-mouse secondary antibody (Millipore, USA) and 16B12 monoclonal antibody (Millipore) were added, and the results were visualized by enhanced chemiluminescence assay (GE Healthcare, UK).
HCE cells were first incubated in 150 mm dishes, serum-starved in M199 containing 1% FBS for 6 h, and treated with M199 containing 1% FBS in the presence of gintonin. Subsequently, the HCE cells were washed with cold PBS (pH 7.4), collected, and lysed with modified radioimmunoprecipitation assay buffer (50 mM Tris-HCl, pH 8.0, 150 mM NaCl, 1% NP-40, 0.25% sodium deoxycholate, 1 mM ethylene glycol tetraacetic acid [EGTA], 2 mM sodium orthovanadate, a protease inhibitor cocktail, and a phosphatase inhibitor cocktail). The HCE cell lysates were analyzed by performing SDS-PAGE and immunoblotting with rabbit anti-phospho-ERK polyclonal antibody (1:1,000) (Cell Signaling Technology, USA). The resulting blot was stripped and re-probed with rabbit anti-ERK polyclonal antibody (1:1,000) (Cell Signaling Technology). Data collecting processes were performed with an LAS-3000 luminescent image analyzer and Multi Gauge software (Fujifilm, Japan).
Proliferation of HCE cells was determined by using the XTT (2,3-bis-(2-methoxy-4-nitro-5-sulfophenyl)-2H-tetrazolium-5-carboxanilide) incorporation assay, which measures DNA synthesis. HCE cells were seeded at 3 × 103 cells per well into 96-well plates coated with a 0.1% gelatin solution. After 48 h, cells were washed with DMEM/F12 and incubated for 6 h with DMEM/F12 without FBS. The HCE cells were washed with fresh DMEM/F12 again and incubated with gintonin at the indicated concentrations. After 48 h, cell proliferation was assessed with 25 µL/well of XTT solution, according to the supplier's instructions (Sigma-Aldrich). Briefly, XTT reagent in DMEM/F12 was added to the cells and followed by an additional 4 h incubation. After incubation, optical densities were measured by an Opsys microplate reader (Thermo LabSystems, USA) at 450–490 nm, with a 630 nm reference filter.
A cell migration experiment was performed as previously described [16]. The chemotactic motility of HCE cells was measured by using modified Boyden chambers in a 48-well plate (Neuro Probe, USA). The polycarbonate membrane with 8 µm pores was coated with 0.1 mg/mL collagen for 30 min, followed by drying for more than 1 h. Gintonin in M199 (0.1% bovine serum albumin) was added to the lower wells of the chambers. The chambers were assembled with the membrane coated with collagen type I (BD Biosciences, USA). Cells (5 × 104 cells/well) were added to the upper wells of the chambers and incubated for 70 to 80 min at 37℃. The HCE cells on the membrane were fixed and stained with Diff Quik (Sysmex, Japan) and mounted on slide glass. Non-migrated cells were removed with Kimwipes. The migrated HCE cells were counted in four fields under a light microscope at a magnification of 200×.
The rabbits were divided into three groups (four rabbits in each group) to evaluate corneal wound healing: ethanol exposure + control (saline 1 drop/eye), ethanol exposure + gintonin (200 µg/eye), and ethanol exposure + Solcorin (1 drop/eye) (Solcoseryl 120 concentrate; Hanlim Pharmaceuticals, Korea) treatment groups. To remove the corneal epithelium from each group, a method using ethanol and an Amoils epithelial scrubber (Innovative Excimer Solutions, Canada) was adopted [15]. Before the removal of the corneal epithelium, topical anesthetics, proparacaine (Alcaine; Alcon, Belgium) and antibiotic, ofloxacin (Effexin; Ildong Pharmaceutical, Korea) eye drops were applied. The eyes were prepared for surgery with 0.2% povidone-iodine solution to sterilize the ocular surface. Absolute ethanol (500–1000 µL/eye) was dropped into the cornea with a disposable syringe; thus, all corneal epithelia were de-epithelialized. The de-epithelialized epithelia were removed with an Amoils epithelial scrubber. Fluorescein (Fluorets; Chauvin, France) was used to visualize the area of epithelial denudement. An impregnated strip with fluorescein was applied to the conjunctiva overlying the dorsal sclera via the application of a drop of saline. Next, the corneal surface was flushed carefully with 10 mL of sterile saline to remove the remnant fluorescein stain. The stained area was photographically recorded by 8× portable slit-lamp biomicroscopy (Hawk Eye; Dioptrix, France) with manual retaining of the rabbit's eye under cobalt blue light.
For 48 h after operation, topical antibiotic eye drops (Effexin, Ildong Pharmaceutical) were applied to rabbits in all groups every 6 h. In addition to antibiotic eye drops, gintonin or Solcorin was also applied in the ethanol exposure + gintonin (200 µg/eye) or ethanol exposure + Solcorin (1 drop/eye) treatment groups, respectively, every 6 h. The ethanol exposure + control groups were only treated with saline (1 drop/eye) every 6 h. After 48 h, corneal re-epithelialization was evaluated by applying the fluorescein staining test after using local anesthetic eye drops (Alcaine; Alcon). After staining the cornea with fluorescein, the ocular surface was photographed by using 8× slit-lamp biomicroscopy under cobalt blue light. In the obtained images, the Image-Pro Plus program (ver. 4.5.0.29; Media Cybernetics, USA) was used to measure the area of fluorescein uptake in the cornea. The area of the healed corneal epithelium was determined by subtracting the area of postoperative fluorescein staining from the area of preoperative fluorescein staining.
The rabbits were divided into five groups (four rabbits in each group) for the evaluation of corneal wound healing: normal control, ethanol exposure + control (saline 1 drop/eye), ethanol exposure + gintonin (200 µg/eye), ethanol exposure + Solcorin (drop/eye), and gintonin (200 µg/eye) alone groups. Topical antibiotic eye drops (Effexin, Ildong Pharmaceutical) were applied to rabbits in all groups every 6 h for 48 h. In addition to antibiotic eye drops, gintonin or Solcorin was also applied to the ethanol exposure + gintonin (200 µg/eye), ethanol exposure + Solcorin (1 drop/eye) or gintonin (200 µg/eye) alone treatment groups, respectively, every 6 h. The normal control and ethanol exposure + control groups were only treated with saline (1 drop/eye) every 6 h. After 48 h, euthanasia was achieved via intravenous injection of KCl (Potassium Chloride 40 inj; DAI HAN Pharm, Korea) under propofol anesthesia (6 mg/kg intravenously; Provive 1%; Myungmoon Pharm, Korea). Corneas from individuals of each group were then removed, fixed with 4% paraformaldehyde solution, and embedded in paraffin. Five-micrometer-thick paraffin sections were prepared and stained by hematoxylin and eosin (H&E) as previously described [19]. To detect DNA fragmentation, an ApopTag Peroxidase In Situ Apoptosis Detection Kit (S7100; Millipore) was used to analyze the paraffin sections [14]. To assess the degree of cell proliferation in corneal wound healing, immunohistochemical analysis for proliferating cell nuclear antigen (PCNA) was performed on the paraffin sections by using mouse anti-PCNA antibody (1:2,000; Santa Cruz Biotechnology, USA) as previously described [3]. The H&E stained sections, DNA fragments, and immunohistochemistry results were analyzed under a light microscope.
The peak increase of cytosolic free Ca2+ at different concentrations of gintonin were plotted to obtain a concentrationresponse curve of the effects of gintonin. Origin software (OriginLab, USA) was used to fit the data to the Hill equation: y/ymax = [A]nH/([A]nH + [EC50]nH), where y is the peak at a given concentration of gintonin, ymax is the maximal peak in the absence of gintonin, EC50 is the concentration of gintonin producing a half-maximal effect, [A] is the concentration of gintonin, and nH is the Hill coefficient. All values are presented as the mean ± SEM. Differences between control vehicle and treatment values were analyzed by using Student's t-test.
For the corneal wound healing study in rabbits, all results obtained are expressed as mean ± SD. Statistical analysis was undertaken by using ANOVA with Tukey's honestly significant difference test to evaluate differences in the area of healing between each groups. In each test, p < 0.05 was considered significant. Statistical tests were performed with SPSS (ver. 22; IBM, USA).
Initially, we examined the effects of gintonin on cytosolic free Ca2+ in HCE cells. Gintonin treatment induced [Ca2+]i mobilization in HCE cells and the effects of gintonin occurred in reversible and concentration-dependent manners (panels A and B in Fig. 1). The EC50 was 0.04 ± 0.01 µg/mL. Gintonin-mediated [Ca2+]i mobilization began without a detectable lag and reached peak values within a few seconds; then, the extent of [Ca2+]i mobilization decreased and approximately returned to its basal level. We observed that treatment of LPA C18:1 to HCE cells also induced [Ca2+]i mobilization, similar to that observed with gintonin (data not shown). The repeated treatment of HCE cells with gintonin did not induce desensitization to [Ca2+]i mobilization (panel A in Fig. 1). These results show that both gintonin and LPA C18:1 can induce [Ca2+]i mobilization in HCE cells.
Because HCE cells express several subtypes of endogenous LPA receptors [2730], we examined the effects of gintonin on [Ca2+]i mobilization in the absence or presence of the LPA1/3 receptor antagonist Ki16425 (10 µM). Treatment of Ki16425 with gintonin clearly blocked gintonin-mediated [Ca2+]i mobilization (panel C in Fig. 1). The active phospholipase C inhibitor U-73122 (10 µM), inositol 1,4,5-trisphosphate receptor antagonist 2-APB (100 µM), and intracellular Ca2+ chelator (BAPTA-AM, 100 µM) also attenuated gintonin-mediated [Ca2+]i mobilization in HCE cells (panel D in Fig. 1). These results show that gintonin, via activation of the LPA receptor-phospholipase C-intracellular IP3 receptor-Ca2+ signaling transduction pathway, induces the mobilization of intracellular Ca2+ stores to increase [Ca2+]i in HCE cells.
In addition, we examined whether gintonin treatment in HCE cells affects ERK1/2 phosphorylation. Gintonin treatment of HCE cells stimulated ERK1/2 phosphorylation in concentration- and time-dependent manners (panels A and C in Fig. 2). Gintonin-mediated ERK1/2 phosphorylation was saturated at 1 µg/mL gintonin (panel C in Fig. 2), and maximal ERK1/2 phosphorylation was observed after 5 min of gintonin treatment (panel B in Fig. 2). The effects of gintonin declined to approximately the basal level after 60 min (panel D in Fig. 2). These results indicate that gintonin-mediated mobilization of [Ca2+]i is linked to rapid ERK1/2 phosphorylation in HCE cells.
Next, we examined the effects of gintonin on the proliferation and migration of HCE cells. Gintonin treatment of HCE cells for 48 h stimulated cell proliferation in a concentration-dependent manner (panel A in Fig. 3A) and induced cell proliferation in time-dependent manner with a treatment of 0.3 µg/mL gintonin (panel B in Fig. 3). The EC50 of cell proliferation was 0.03 ± 0.01 µg/mL (panel A in Fig. 3). In addition, gintonin treatment of HCE cells induced cell migration in a concentration-dependent manner, but the presence of Ki16425 blocked gintonin-mediated cell migration (panel B in Fig. 4). These results indicate that gintonin stimulates HCE cell proliferation and migration through LPA receptors.
We then investigated the therapeutic effects of gintonin on corneal damage induced by ethanol in a rabbit model. Representative results of the fluorescein dye test at 48 h after operation are shown in Fig. 5 and Table 1 shows a summary of the eroded epithelium size. Compared to the ethanol-exposed control saline treatment (panel A in Fig. 5), gintonin treatment significantly increased the healing area by 50.14% ± 14.35% (panel B in Fig. 5). In addition, compared to the control saline treatment, Solcorin treatment also increased the healing area (panel C in Fig. 5 and Table 1). These results indicate that treatments with gintonin or Solcorin have therapeutic effects on corneal damage.
We investigated the histopathological effects of gintonin on ethanol-induced corneal damage in a rabbit model. Normal corneas are composed of corneal epithelium with stratified squamous epithelial cells (5–7 cell layers thick), the anterior limiting membrane (Bowman's membrane), stroma (approximately 90% of the cornea) with resident keratocytes, the posterior limiting membrane (Descemet's membrane), and the endothelium of the inner surface (Figs. 5 and 6). Two days after ethanol exposure, the epithelial cell nuclei disappeared or were markedly decreased in the damaged area of the cornea (panel B in Fig. 6); however, epithelial cells in regenerating, proliferating, and migrating stages were observed at the margin of the damaged area (arrows in panel B in Fig. 6). Additionally, infiltration of inflammatory cells was increased in the superficial stroma (panel B in Fig. 6). Interestingly, compared to the ethanol-exposed group, gintonin treatment in the form of eye drops (200 µg/eye) increased the number of epithelial cells in the superficial layer of the epithelium, as well as the epithelial thickness, but decreased the infiltration of inflammatory cells in the stroma.
Solcorin treatment showed effects similar to those of the gintonin treatment (panel D in Fig. 6). Three days after ethanol exposure, compared to that observed in the control group, the number of PCNA-immunoreactive cells decreased in the epithelium and stroma (panels F and G in Fig. 6). Moreover, compared to that observed in the ethanol-exposed group, gintonin treatment increased PCNA immunoreactivity; these results were similar to those observed in the Solcorin-treated group (panels H and I in Fig. 6). In addition, compared to the normal group, after 3 days, ethanol exposure increased the number of apoptotic cells in the epithelium and stroma (panels K and L in Fig. 6). Compared with that observed in the ethanol-exposed group, gintonin or Solcorin treatment decreased the number of apoptotic cells in the epithelium and stroma (panels M and N in Fig. 6). Treatment with gintonin alone (200 µg/eye) did not affect the histological structure of the cornea (panels E, J and O in Fig. 5). These results confirm that gintonin has therapeutic histochemical effects in a rabbit model of corneal damage.
The cornea is part of the external tunic of the eye. The corneal epithelium is the first layer of the cornea in animals and humans, and that epithelium layer is easily damaged by various chemical, microbiological, and physical hazards [2128]. In addition, recent reports have demonstrated that elective eye surgeries to improve or correct vision, such as laser-assisted in situ keratomieusis or laser-assisted subepithelial keratectomy, are accompanied by corneal damage [8]. When corneas are injured, application of agents that provide rapid healing is indispensable for preserving healthy corneas and maintaining vision. Currently, only a few types of treatment are available for rapid corneal restoration, even though the demand for therapeutic agents for corneal wound healing is increasing. In addition, relatively little is known about herbal medicine-derived agents that promote corneal wound healing.
In the present study, we observed four principal results, which indicate that gintonin-mediated mobilization of [Ca2+]i via LPA receptor activations is involved in the promotion of in vivo corneal wound healing. First, gintonin induces a large amount of [Ca2+]i mobilization even at low concentrations in HCE cells [2]. Second, [Ca2+]i mobilization in HCE cells by gintonin was blocked by the LPA1/3 receptor antagonist Ki16425. Third, gintonin-mediated mobilization of [Ca2+]i is induced via the PLC-IP3-Ca2+ pathway and is coupled with downstream ERK phosphorylation. Fourth, gintonin-mediated signal transduction via LPA receptors in HCE cells induces in vitro cell migration and proliferation and further promotes in vivo corneal wound healing in a rabbit model. The present study shows that in vitro gintonin-mediated changes in cellular activities via LPA receptors are linked to the promotion of in vivo corneal wound healing in a rabbit model.
Endogenous LPA that is present in animals is a simple phospholipid that exerts a myriad of cellular effects as a mitogen or in lipid-derived growth factor regulation in most cells types and organs [4]. Endogenous LPA exists in various body fluids, including eye fluid and plasma, in nanomolar concentrations. In addition to the role of LPA as a phospholipid growth factor, there are at least six G-protein-coupled LPA receptor subtypes (LPA1–6) expressed in most cell types across a variety of tissues, including the cornea [31]. Corneal injury induces changes in LPA receptor expression, LPA-induced [Ca2+]i mobilization [30], and LPA concentration in the cornea [2027], indicating that LPA is physiologically associated with normal corneal function and the corneal wound healing process. These results raise the possibility that exogenous LPA such as gintonin can have a key role in in vivo eye function, and shows that external supplementation of LPA to injured cornea can promote corneal wound healing. The present study provides additional evidence that external application of gintonin to injured cornea can help corneal wound healing by stimulating cell migration and division in HCE cells via the Ca2+-signaling pathway. Thus, gintonin could be used as an exogenous LPA for eye wound healing [24].
In traditional herbal medicine, people usually orally ingest ginseng or ginseng extracts to maintain body homeostasis. In previous reports, the long-term administration of gintonin via the oral route has been shown to attenuate brain inflammation and amyloid plaque formation, restore Aβ-induced memory dysfunctions in transgenic Alzheimer's disease animal models, and display anti-metastatic effects by inhibiting autotaxin activity [1011]. As a result of the present study, we deliver another use for gintonin; it could be a topically applied treatment in the form of eye drops for the rapid restoration of corneal damage. The development of topical gintonin as a therapeutic agent for corneal damage has at least two advantages over the oral administration of gintonin. First, topical gintonin can be more effective than oral administration to corneal recovery because the cornea does not have any blood vessels. Second, the topical application of gintonin as eye drops could be cost-effective, because therapeutic effects can be observed with a substantially lower amount of gintonin than that needed to observe therapeutic effects following administration via the oral route.
Regarding the mode of action of gintonin in promoting effects of corneal wound healing, Choi et al. [5] showed that GLP151 is a LPA binding protein. The phosphate group of LPA interacts with the imidazole ring of histidine residues at the C-terminus of GLP151 with hydrogen bonds, and the remaining acyl chain of LPA interacts with other amino acids through interactions with GLP151 [5], which was identified as a candidate ginseng-derived carrier or transporter of LPA, as it delivers LPA to LPA receptors [5]. The protein components of gintonin may function as carriers and/or stabilizers of LPAs and may help LPAs act as functional, high-affinity ligands of G-protein-coupled LPA receptors [5]. Currently, we are investigating further preclinical effects of gintonin on eye diseases such as corneal damage, dry eye, and eye ulcers. In addition, we previously showed that gintonin-mediated mobilization of [Ca2+]i is coupled with ERK1/2 phosphorylation in human umbilical vein endothelial cells; ERK1/2 phosphorylation is associated with primary signaling events that induce human umbilical vein endothelial cell proliferation and migration [12]. LPA also increases ERK1/2 phosphorylation in primary porcine corneal epithelial cells [30]. The present study raises the possibility that gintonin-mediated mobilization of [Ca2+]i and gintonin-ERK1/2 phosphorylation could be involved in corneal wound healing. In the future, gintonin could be developed as a specific target-oriented medicine for treatment of corneal damage.
In conclusion, we have demonstrated that LPA receptor activation by gintonin treatment could be effective in corneal wound healing. We suggest that gintonin is a good candidate for the development of a novel ginseng-derived therapeutic agent for rapid corneal wound healing through topical application.
Figures and Tables
Fig. 1
Effects of gintonin (GT) on intracellular calcium transients in human corneal epithelium (HCE) cells. (A) Representative trace obtained after application of the indicated concentration of gintonin. (B) Concentration-response relationship curve for gintonin-induced [Ca2+]i transients in HCE cells. Each point represents the mean ± SEM (n = 3–4). (C) Representative traces of gintonin-mediated [Ca2+]i transients in the absence or presence of LPA1/3 receptor antagonist Ki16425. (D) Histograms representing net increases of gintonin-mediated [Ca2+]i transients calculated from traces obtained in the absence or presence of various antagonists or blockers (*p < 0.05, n = 5–6).
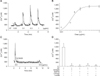
Fig. 2
Effects of gintonin on extracellular signal-regulated kinase (ERK) 1/2 phosphorylation in human corneal epithelium (HCE) cells. (A) Gintonin stimulated ERK1/2 phosphorylation in a concentration-dependent manner; however, a decrease in ERK1/2 phosphorylation was observed at a high concentration of gintonin (10 µg/mL). Cells were stimulated for 5 min with vehicle (control) or the indicated concentration of gintonin, and ERK1/2 phosphorylation was evaluated as described in the Materials and Methods section. The upper panels represent basal and different concentrations of gintonin-stimulated ERK1/2 phosphorylation obtained by immunoblotting from a representative experiment performed with HCE cells. (B) Data are presented as percentage increases of basal ERK1/2 phosphorylation, where the basal amount of ERK1/2 phosphorylation in untreated cells was assigned a value of 100% (*p < 0.01, compared with the gintonin-untreated control). (C) Gintonin-mediated ERK1/2 phosphorylation was time-dependent; ERK1/2 phosphorylation peaked 5 min after gintonin treatment and decreased after 60 min to the basal level. Cells were stimulated for the indicated time with gintonin (1 µg/mL). (D) Data are presented as fold increases of basal ERK1/2 phosphorylation, where the basal amount of ERK1/2 phosphorylation in untreated cells was assigned a value of 100% (*p < 0.01, compared with the gintonin-untreated control). Data shown represent the mean ± SEM values of duplicate analyses from each of three separate experiments.
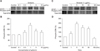
Fig. 3
Effects of gintonin (GT) on human corneal epithelium cell proliferation. (A) Gintonin stimulated cell proliferation in a concentration-dependent manner. (B) Gintonin-mediated cell proliferation was time-dependent. Cells were treated with the indicated concentrations of gintonin and subjected to XTT assay as described in the Materials and Methods section. Data are presented as the mean ± SEM (n = 6; *p < 0.05, **p < 0.01, compared with untreated controls).
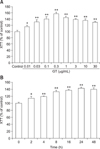
Fig. 4
Gintonin (GT) stimulates migration of human corneal epithelium cells. (A) Confluent cells were scratched and incubated with gintonin at the indicated concentrations in DMEM for 20 to 24 h. (B) The relative migration was quantified by comparing the migration of the treated cells to that of the untreated cells (control). The data are presented as the mean ± SEM (n = 3–6) (*p < 0.05, compared to untreated controls). Scale bars = 100 µm.
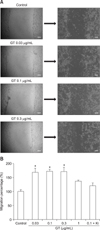
Fig. 5
Fluorescein staining of eyes at 48 h post-operation. (A) Ethanol-exposed control group. (B) Ethanol exposure + gintonin-treated group. (C) Ethanol exposure + Solcorin-treated group.
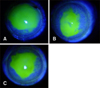
Fig. 6
Therapeutic effect of gintonin in ethanol-induced corneal damage. Paraffinized sections of corneas from normal control (A, F, and K), ethanol-exposed (B, G, and L), ethanol-exposed and gintonin (200 µg/eye)-treated (C, H, and M), ethanol-exposed and Solcorin-treated (D, I, and N), and gintonin alone-treated (E, J, and O) groups at 2 days after ethanol exposure were stained with H&E (A–E), immunostained with proliferating cell nuclear antigen (PCNA) antibody (F–J), or stained with a TUNEL kit (K–O). (A–E) Gintonin treatment partially recovered the damaged epithelium and decreased infiltration of inflammatory cells in the stroma (B and C). Solcorin showed effects similar to those of gintonin (D). Arrows in (A) indicate the epithelium in proliferating and migrating stages. (F–J) Decreased PCNA immunoreactivity was increased in the gintonin-treated group compared with that observed in the ethanol-exposed group and was similar to that of the Solcorin-treated group. (K–O) The increased number of apoptotic cells was decreased in the gintonin-treated group compared with that in the ethanol-exposed group and was similar to that of the Solcorin-treated group. (E, J, and O) Treatment with gintonin alone did not affect the histological structure of the corneas. Epithelium (e), anterior limiting membrane (a), and stroma (s). Scale bars = 100 µm.
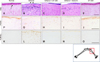
References
1. Araki-Sasaki K, Aizawa S, Hiramoto M, Nakamura M, Iwase O, Nakata K, Sasaki Y, Mano T, Handa H, Tano Y. Substance P-induced cadherin expression and its signal transduction in a cloned human corneal epithelial cell line. J Cell Physiol. 2000; 182:189–195.


2. Araki-Sasaki K, Ohashi Y, Sasabe T, Hayashi K, Watanabe H, Tano Y, Handa H. An SV40-immortalized human corneal epithelial cell line and its characterization. Invest Ophthalmol Vis Sci. 1995; 36:614–621.
3. Cho IH, Hong J, Suh EC, Kim JH, Lee H, Lee JE, Lee S, Kim CH, Kim DW, Jo EK, Lee KE, Karin M, Lee SJ. Role of microglial IKKβ in kainic acid-induced hippocampal neuronal cell death. Brain. 2008; 131:3019–3033.


4. Choi JW, Herr DR, Noguchi K, Yung YC, Lee CW, Mutoh T, Lin ME, Teo ST, Park KE, Mosley AN, Chun J. LPA receptors: subtypes and biological actions. Annu Rev Pharmacol Toxicol. 2010; 50:157–186.


5. Choi SH, Hong MK, Kim HJ, Ryoo N, Rhim H, Nah SY, Kang LW. Structure of ginseng major latex-like protein 151 and its proposed lysophosphatidic acid-binding mechanism. Acta Crystallogr D Biol Crystallogr. 2015; 71:1039–1050.


6. Choi SH, Kim HJ, Kim BR, Shin TJ, Hwang SH, Lee BH, Lee SM, Rhim H, Nah SY. Gintonin, a ginseng-derived lysophosphatidic acid receptor ligand, potentiates ATPgated P2X1 receptor channel currents. Mol Cells. 2013; 35:142–150.


7. Choi SH, Shin TJ, Lee BH, Hwang SH, Kang J, Kim HJ, Park CW, Nah SY. An edible gintonin preparation from ginseng. J Ginseng Res. 2011; 35:471–478.


8. Dawson DG, Edelhauser HF, Grossniklaus HE. Long-term histopathologic findings in human corneal wounds after refractive surgical procedures. Am J Ophthalmol. 2005; 139:168–178.


9. Hwang SH, Lee BH, Choi SH, Kim HJ, Jung SW, Kim HS, Shin HC, Park HJ, Park KH, Lee MK, Nah SY. Gintonin, a novel ginseng-derived lysophosphatidic acid receptor ligand, stimulates neurotransmitter release. Neurosci Lett. 2015; 584:356–361.


10. Hwang SH, Lee BH, Kim HJ, Cho HJ, Shin HC, Im KS, Choi SH, Shin TJ, Lee SM, Nam SW, Kim HC, Rhim H, Nah SY. Suppression of metastasis of intravenously-inoculated B16/F10 melanoma cells by the novel ginseng-derived ingredient, gintonin: involvement of autotaxin inhibition. Int J Oncol. 2013; 42:317–326.


11. Hwang SH, Shin EJ, Shin TJ, Lee BH, Choi SH, Kang JY, Kim HJ, Kwon SH, Jang CG, Lee JH, Kim HC, Nah SY. Gintonin, a ginseng-derived lysophosphatidic acid receptor ligand, attenuates Alzheimer's disease-related neuropathies: involvement of non-amyloidogenic processing. J Alzheimers Dis. 2012; 31:207–223.


12. Hwang SH, Shin TJ, Choi SH, Cho HJ, Lee BH, Pyo MK, Lee JH, Kang J, Kim HJ, Park CW, Shin HC, Nah SY. Gintonin, newly identified compounds from ginseng, is novel lysophosphatidic acids-protein complexes and activates G protein-coupled lysophosphatidic acid receptors with high affinity. Mol Cells. 2012; 33:151–162.


13. Im DS, Nah SY. Yin and Yang of ginseng pharmacology: ginsenosides vs gintonin. Acta Pharmacol Sin. 2013; 34:1367–1373.


14. Jang M, Lee MJ, Cho IH. Ethyl pyruvate ameliorates 3-nitropropionic acid-induced striatal toxicity through anti-neuronal cell death and anti-inflammatory mechanisms. Brain Behav Immun. 2014; 38:151–165.


15. Kim JY, Choi YM, Jeong SW, Williams DL. Effect of bovine freeze-dried amniotic membrane (Amnisite-BA) on uncomplicated canine corneal erosion. Vet Ophthalmol. 2009; 12:36–42.


16. Kim YM, Namkoong S, Yun YG, Hong HD, Lee YC, Ha KS, Lee H, Kwon HJ, Kwon YG, Kim YM. Water extract of Korean red ginseng stimulates angiogenesis by activating the PI3K/Akt-dependent ERK1/2 and eNOS pathways in human umbilical vein endothelial cells. Biol Pharm Bull. 2007; 30:1674–1679.


17. Kimura Y, Sumiyoshi M, Kawahira K, Sakanaka M. Effects of ginseng saponins isolated from Red Ginseng roots on burn wound healing in mice. Br J Pharmacol. 2006; 148:860–870.


19. Lee MJ, Jang M, Choi J, Lee G, Min HJ, Chung WS, Kim JI, Jee Y, Chae Y, Kim SH, Lee SJ, Cho IH. Bee venom acupuncture alleviates experimental autoimmune encephalomyelitis by upregulating regulatory T cells and suppressing Th1 and Th17 responses. Mol Neurobiol. 2016; 53:1419–1445.


20. Liliom K, Guan Z, Tseng JL, Desiderio DM, Tigyi G, Watsky MA. Growth factor-like phospholipids generated after corneal injury. Am J Physiol. 1998; 274:C1065–C1074.
21. Lu L, Reinach PS, Kao WW. Corneal epithelial wound healing. Exp Biol Med (Maywood). 2001; 226:653–664.


22. Nah SY. Ginseng ginsenoside pharmacology in the nervous system: involvement in the regulation of ion channels and receptors. Front Physiol. 2014; 5:98.


23. Pazyar N, Yaghoobi R, Rafiee E, Mehrabian A, Feily A. Skin wound healing and phytomedicine: a review. Skin Pharmacol Physiol. 2014; 27:303–310.


24. Pyo MK, Choi SH, Shin TJ, Hwang SH, Lee BH, Kang J, Kim HJ, Lee SH, Nah SY. A simple method for the preparation of crude gintonin from ginseng root, stem, and leaf. J Ginseng Res. 2011; 35:209–218.


25. Shin TJ, Kim HJ, Kwon BJ, Choi SH, Kim HB, Hwang SH, Lee BH, Lee SM, Zukin RS, Park JH, Kim HC, Rhim H, Lee JH, Nah SY. Gintonin, a ginseng-derived novel ingredient, evokes long-term potentiation through N-methyl-D-aspartic acid receptor activation: involvement of LPA receptors. Mol Cells. 2012; 34:563–572.


26. Tanaka T, Horiuchi G, Matsuoka M, Hirano K, Tokumura A, Koike T, Satouchi K. Formation of lysophosphatidic acid, a wound-healing lipid, during digestion of cabbage leaves. Biosci Biotechnol Biochem. 2009; 73:1293–1300.


27. Wang DA, Du H, Jaggar JH, Brindley DN, Tigyi GJ, Watsky MA. Injury-elicited differential transcriptional regulation of phospholipid growth factor receptors in the cornea. Am J Physiol Cell Physiol. 2002; 283:C1646–C1654.


28. Watsky MA, Griffith M, Wang DA, Tigyi GJ. Phospholipid growth factors and corneal wound healing. Ann N Y Acad Sci. 2000; 905:142–158.


29. Watterson KR, Lanning DA, Diegelmann RF, Spiegel S. Regulation of fibroblast functions by lysophospholipid mediators: potential roles in wound healing. Wound Repair Regen. 2007; 15:607–616.

