Abstract
Figures and Tables
Fig. 1
Transcriptional expression profiles of Wnt signaling-related genes in canine mammary tumors (CMTs). Total RNA extracted from CMT samples or normal mammary gland tissues were reverse transcribed into cDNA for quantitative PCR analysis with an RT2 Profiler PCR Array system. The transcriptional level of these genes are expressed as fold changes. (A and B) Wnt and Wnt antagonist genes. (C) Frizzled receptors and co-receptor. (D) β-catenin and destruction complex-related factors. (E) Transcription factors. (F) Target genes. (G) Upregulated genes in 4 groups of different CMT subtypes. Benign, benign tumor; LM, low malignant tumor; HM, high malignant tumor; CC, complex carcinoma; CMM, carcinoma and malignant myoepithelioma; EC, epithelial carcinoma (malignant tumors with generally only one component (epithelial)). Significant differences expressed as *p < 0.05 while highly significant differences are expressed as **p < 0.01. Asterisk(s) directly above the error bar of each column indicates a comparison between the indicated group and the benign group; while the Asterisk(s) above the drawn lines means the comparison between the tumor groups indicated by the lines.
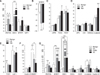
Fig. 2
The expression of β-catenin and cyclin D1 in CMTs detected via immunohistochemical staining. Tissue sections were probed with either anti-β-catenin antibodies (A–H) or anti-cyclin D1 antibodies (I–L). (A) Reduced staining of both membrane and cytoplasm; weak (D), moderate (B, E and F) and strong (C and G) staining of the cytoplasm; distinct (D), partially disappeared (C and G) or mostly disappeared (B, E and F) staining of membrane; and (H) weakly staining in cytoplasm and more than 70% of membrane staining in normal tissue section. (I–K), nuclear staining of cyclin D1. Negative control staining using PBS instead of primary antibodies was performed with normal mammary tissue (M). The red arrows indicate membrane staining of β-catenin, while the red arrowhead indicates cytoplasmic staining of β-catenin. The black arrows indicate nuclear staining of cyclin D1. The subtype and grading of the CMT samples are shown below each image. Scale bar = 20 µm.
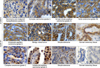
Fig. 3
Analysis of subcellular localization of β-catenin in normal mammary tissues or CMTs. The 100 CMT samples included 37 benign CMTs, 30 low malignant (LM) CMTs and 33 highly malignant (HM) CMTs, as well as 8 normal mammary tissue samples were subjected to IHS analysis. Scores of membrane-stained (A) or cytoplasm-stained (B) samples ranged from 0 (non-staining) to 3 (strongly positive stain) according to the criteria of Khramtsov et al. [11].
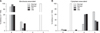
Fig. 4
Expression of β-catenin, TCF7, LEF1 and cyclin D1 in CMTs analyzed by immunoblotting. The samples include 1 normal canine mammary tissue (N), 2 complex adenomas (33 and 35), 2 simple adenomas (4 and 2), 1 complex carcinoma (43), 2 simple tubulo-papillary carcinomas (78, Grade I; 80, Grade II), 2 intraductal papillary carcinomas (86, Grade I; 84, Grade II) and 2 solid carcinomas (93, Grade II; 92, Grade III). Primary antibodies against β-catenin, TCF7, LEF1 and cyclin D1 were used for detection. β-actin was used as the reference protein. The malignant grades of the CMTs are marked as I, II, and III on the top of the image.
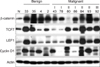
Table 2
Classification and grading for canine mammary tumor samples (N = 100)
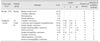
In each column, the numbers of CMT samples included in the RT2 Profiler PCR Array assays are presented in brackets. -, benign CMT samples not subjected to grading. A, absence of necrosis or lymphatic invasion. CC, complex carcinoma; CMM, carcinoma and malignant myoepithelioma; EC, epithelial carcinoma.
Table 3
Correlations of β-catenin or cyclin D1 expression and clinicopathological parameters in canine mammary tumors (CMTs)
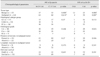
M, membrane-associated; C, cytoplasm-associated. a denotes difference among CMTs and Normal mammary gland tissues, while b denotes difference among benign and malignant CMTs. Statistical analysis was performed by using chi-squafmembrane staining in normal tissue sectionred and Fisher's exact tests. IHS, immunohistochemical scores; CC, complex carcinoma; CMM, carcinoma and malignant myoepithelioma; EC, epithelial carcinoma.
Acknowledgments
References






















