Abstract
Ovarian stimulation with commercial preparations of equine chorionic gonadotropin (eCG) produces extremely variable responses in domestic animals, ranging from excessive stimulation to practically no stimulation, when applied on the basis of their declared unitage. This study was conducted to analyze four commercial preparations from different manufacturers via reversed-phase HPLC (RP-HPLC) in comparison with a reference preparation and an official International Standard from the World Health Organization. The peaks obtained by this qualitative and quantitative physical–chemical analysis were compared using an in vivo bioassay based on the ovarian weight gain of prepubertal female rats. The RP-HPLC data showed one or two peaks close to a main peak (tR = 27.9 min), which were related to the in vivo bioactivity. Commercial preparations that have this altered peak showed very little or no in vivo activity, as demonstrated by rat ovarian weight and in peripubertal gilts induced to ovulate. Overall, these findings indicate that RP-HPLC can be a rapid and reliable tool to reveal changes in the physicochemical profile of commercial eCG that is apparently related to decreased biological activity of this hormone.
Equine chorionic gonadotropin (eCG), which was originally known as pregnant mare serum gonadotropin (PMSG), was first described by Cole and Hart [13]. This compound belongs to a family of glycoprotein hormones produced between day 36 and 120 of pregnancy by endometrial cups developed from cells of the chorionic girdle and plays an important role in mare gestation maintenance [226]. This hormone has a relatively long half-life (t1/2 ∼ 45.6 h in cows) because of its high carbohydrate content, 10% of which is composed of sialic acid [1124]. These characteristics, together with the singular property of having follicle-stimulating (FSH) and luteinizing (LH) activities in species other than equine species, has led to widespread use of eCG in veterinary medicine for the treatment of ovarian inactivity [6], anestrous [30] and for superovulation induction in embryo transfer programs [9]. More recently, eCG has been widely applied to the optimization of hormonal protocols directed toward estrus and ovulation synchronization aimed at artificial insemination in fixed time (TAI) in beef and dairy cattle [63133]. Conversely, an eCG dose that is recommended in superovulation (2,000–3,000 IU/animal) and for the TAI (200–600 IU/animal) protocols frequently induces extremely variable responses, with excessive ovarian stimulation in some animals, but total lack of response in others [917]. This variation in response can be due to different factors related to the animal (species, breed, physical condition, ovarian status, etc.), or to the eCG dosage [917]. Moreover, the inconsistency of field results observed with the use of some commercial preparations or batches of eCG warrants particular analysis because these aspects might introduce an additional source of variation into the ovarian response, as shown by Henderson et al. [18] for commercial pituitary porcine FSH.
Currently, the conventional purification process of eCG involves fractional precipitation, the removal of impurities, pH adjustment and final precipitation [22]. The standardization of the biological potency of commercial eCG products or batches into international units (IU) is conducted by tests that measure the ovarian weight increase of prepubertal female rats due to increasing doses of the hormone using the International Standard of eCG (the World Health Organization [WHO]) as the reference material [12]. In principle, the quality tests carried out by different manufacturers of commercial eCG should guarantee the required potency of the products [20]. However, little is known about the characteristics of purified eCG preparations and limitations in the quality control process. Additionally, improper preservation conditions might be responsible for large variations or losses in the bioactivity of the product.
Our research group at IPEN-CNEN/São Paulo has placed special emphasis on chromatographic methods based on reversed-phase high-performance liquid chromatography (RP-HPLC) to determine purity levels or structural alterations in gonadotropins, such as human chorionic gonadotropin (hCG), luteotropin (hLH) or menotropin (hMG), which can be strongly related to their biological activity [35]. The possibility of an equivalence, or at least of a relationship, between the physical–chemical characteristics and the in vivo bioactivity of a molecule, makes RP-HPLC an invaluable tool for the rapid and precise assessment of the potency of eCG. Thus, the specific goal of the present study was to study the relationship between the biological activity and the physical–chemical properties of different commercial preparations of eCG, especially based on RP-HPLC analysis, as well as to identify the isoforms responsible for most of its in vivo potency.
All procedures utilized for animal breeding and sacrificing followed the international recommendations for animal use and were approved by the Ethical Committee of the Animal Production Institute of the São Paulo Agency for Agribusiness Technology (license No. IZ-159).
Commercial preparations of eCG from Syntex (Argentina), Intervet International (Netherland), Biomega (Uruguay) and Hebei Ynuo Animal Husbandry Science and Technology (China) were purchased from a regional veterinary drugstore. The codes I, II, III and IV were randomly assigned to the four commercial products, and each was analyzed via bicinchoninic acid (BCA), RP-HPLC and an in vivo bioassay. The declared units per ampoule of preparations I, II, III and IV were 5,000, 6,000, 5,000 and 1,000 IU, respectively. Gonadotropin from pregnant mares' serum (product No. G4877; Sigma, USA) with 1,000 IU/ampoule and the International Standard of Equine Serum Gonadotropin (code: 62/001; National Institute for Biological Standards and Control, UK) with 1,600 IU per ampoule were used as reference control.
The total protein content of commercial eCG products and the reference preparation (Sigma) was determined by the BCA colorimetric method described by Smith et al. [32] based on the absorbance at 562 nm using bovine serum albumin (BSA) for the standard curve.
The identity and purity of the commercial and reference preparations of eCG were investigated by RP-HPLC using a methodology derived from those previously established in our laboratory for the analysis of human LH, FSH and hCG, which have confirmed the system precision [342129]. A Shimadzu Model SCL-10AHPLC apparatus with a SPD-10AV UV detector and a Vydac 214TP C4 54 column (25 cm × 4.6 mm; pore diameter 300 Å and particle diameter of 5 µm; Grace, USA) coupled to a Vydac 214FSK 54 guard pre-column (1 cm × 4.6 mm; Grace) was used. Solutions A (0.05 M ammonium phosphate pH 8.6) and B (acetonitrile) were utilized in a 40 min gradient using a concentration of B in A that increased from 15% to 60% and a 10 min isocratic mode with 60% B. The sample volume was 100 µL and the running time was 50 min with a flow rate of 0.5 mL/min. The column temperature was maintained at 25℃ and detection was by UV absorbance at 220 nm. The precision of this specific quantitative methodology based on direct eCG determinations provided CVs of 1.3% and 3.1% for intra- and inter-assay, respectively. From a qualitative point of view, we calculated retention times (tR) with CVs < 0.36% and < 3% for intra-day and inter-day determinations, respectively. For what concerns a complete validation of the linear dose-response curve we can refer to the data obtained in an analogous RP-HPLC assay based on the same methodology for human hormones (hCG and hLH) [3]. In that case, the equation of the curve was: Yarea = 959.4 Xµg − 27.6 (r = 0.9999; p < 0.001; n = 20), the sensitivity was 34 ng, and the average recovery was 99.7 ± 3.04%.
Three main peaks corresponding to different retention times (tR = 27.9, 34.4 and 36.2 min) were eluted and collected from eCG preparations I and Sigma. Based on the total protein content, aliquots of 1.43 µg that theoretically corresponded to 12 IU were obtained from each peak. The biological activity of the different eCG peaks was determined by an ovarian augmentation test carried out in prepubertal female rats according to Cole and Erway [12]. Briefly, approximately 21-day-old female Wistar rats (Ratus norvegicus) weighing 65.5 ± 1.2 g were purchased from the Multidisciplinary Center for Biological Investigation of Laboratory Animal Science (CEMIB-UNICAMP, Campinas, Brazil) and randomly separated into groups of three animals each, which received the equivalent of 12 IU/animal of eCG dissolved in 100 µL of saline buffer solution intraperitoneally (i.p). The control group only received saline. Forty-eight hours after injection, the rats were euthanized via i.p. injection of a mixture of 80 mg ketamine with 15 mg xylazine and the genital tract was removed. The ovaries were then weighed separately on an analytical balance (0.1 mg precision).
The biological activity of the different commercial eCG preparations (I, II, III, IV, Sigma and saline) was determined by an ovarian augmentation test utilizing immature rats (n = 6 in each treatment), as described in the previous section.
Eighteen 6-month-old peripubertal gilts (Pen Ar Lan Lineage, Brazil) with a mean body weight of 88.1 ± 8.4 kg were randomly distributed among three groups of six animals each. Groups 1 and 2 were treated, by intramuscular (i.m.) route, with 750 IU of commercial eCG preparation I and II, respectively, whereas group 3 (control) was treated with saline. Seventy-two hours later, all groups received an i.m. injection of 500 IU of hCG (Chorulon; MSD Animal Health, Brazil). Six days after the hCG injection, gilts were slaughtered and the ovaries were recovered to assess their weight and the presence of corpora lutea.
Continuous variables (ovarian weight) were analyzed by one-way ANOVA, with differences among groups identified by Duncan's test. The normality of the residual error and the homogeneity of variances of the data were corroborated by Levene's test. The categorical variables (ovulating vs. non-ovulating gilts) were analyzed utilizing Fisher's exact test.
The total protein content determined for the four commercial preparations and for the reference preparation from Sigma via BCA is shown in Table 1.
Normalizing the protein content to 1,000 IU for each preparation revealed that the same declared biological activity corresponded to very different protein masses. Different specific bioactivities ranging from 0.61 IU/µg (preparation II) to 8.33 IU/µg (preparation I) were calculated. This was somewhat arbitrary since it is based on a nominal activity that should be confirmed; nevertheless, it provides a preliminary approximation of the different purity levels of the eCG preparations.
The RP-HPLC analysis of eCG reference preparations confirmed the presence of an isolated major peak with practically identical retention times (tR = 27.94 and 27.96 min for Sigma and WHO, respectively; Fig. 1).
The three commercial preparations (I, III and IV) also showed similar tR values of 27.90, 27.76 and 27.84 min, differing from the WHO eCG by −0.06 and −0.2 min, respectively. These data strongly suggest that this peak represents the active form of the hormone. However, preparation II showed a difference of +0.41 min for the tR of the peak that was assumed to be active, as well as two higher peaks with quite different tR values (Fig. 2).
The bioassay in prepubertal female rats conducted to compare the biological activity of the main peak (tR = 27.9 min) with that of the other peaks (tR = 34.4 or 36.2 min) obtained via preparative RP-HPLC of eCG from Sigma and commercial preparation I showed a significant biological effect (p < 0.01) of the main peak (tR = 27.9 min), whereas the biological activity of the other peaks (tR = 34.4 or 36.2 min) did not differ from that of the control (Table 2).
The results of the ovarian weight-gain bioassay carried out with all commercial eCG preparations are shown in Table 3. Again, the ovarian weight of preparation II was not different from that of the negative control (p = 0.298), whereas that of preparations I, III and IV was significantly greater than the control value (p < 0.05).
The purest eCG commercial preparation I was then compared to the least pure preparation II to evaluate the ovarian response in young gilts. Preparation I induced ovulation in five of six animals (83.3%), against one (16.6%) for preparation II and none (0%) for saline (p < 0.05). When compared with saline, the ovarian weight was significantly different in gilts treated with eCG from preparation I (p < 0.05), but not from those treated with preparation II (p > 0.05) (Table 4).
In the present study, RP-HPLC was applied to test the quality of different commercial preparations of eCG for the first time. As shown in Figs. 1 and 2, this physical–chemical approach can be used as a rapid, useful and inexpensive tool for detecting potential structural alterations that can compromise the bioactivity of eCG preparations.
The eCG from Sigma can be used as a substitute reference for the WHO International Standard of eCG in this type of analysis. Commercial preparations I and III showed nearly the same activity as the standards, even though preparation III was about two-fold less pure, as can be seen in Table 1. Preparation IV was even less pure (∼five-fold) than preparation I, but appeared to possess a slightly higher bioactivity per ampoule than declared. Preparation II, which was nominally the most impure, showed the most altered tR of the active peak and little or no bioactivity.
Peaks with very different tR values from those shown by preparation II might have been caused by protein impurities, but could also have reflected different excipients added to the drug formulation [5]. Furthermore, the different tR values of the analogous hormonal preparations might have been due to structural alterations mostly related to the carbohydrate moiety, with a subsequent alteration in the hydrophobicity and possibly in the bioactivity of the molecule [29].
Few publications have reported the use of different HPLC modes for human-CG analysis and/or purification [7814151923]. Moreover, to the best of our knowledge, only one report has dealt with eCG analysis, in which high-performance size-exclusion chromatography (HPSEC) was used to compare the elution characteristics of the zebra chorionic gonadotropin with that of purified eCG [25].
The low amount of bioactive eCG evidenced by the low ovulation induction in gilts treated with commercial preparation II might be responsible for the variability in ovarian response in domestic and laboratory animals. In a study of heifers superovulated with 1,000 IU of three different batches of commercial eCG, Newcomb et al. [27] were unable to identify differences in the ovulation rate between batches. However, one of the batches possessed, on average, 16.7% (maximum 41%) lower total gonadotrophic potency. Although the difference was non-significant, heifers treated with this batch had a lower ovarian response (6.2 ovulations) than those treated with other batches (9.4 ovulations). Upon analysis of different commercial products of eCG in female mice, Ciller et al. [10] found differences in the weight of ovaries that were associated with subtle differences in the pH of eCG isoforms.
Drugs that are adequately freeze-dried (lyophilized) remain stable for several years, even when stored without refrigeration [28]; however, degradation can occur because of inadequate purification or lyophilization processes [34]. Additionally, there is evidence that the biological potency of eCG might be related to differences in the eCG titer of serum collected from pregnant mares [1]. Although sera with different eCG titers showed no qualitative differences based on immunoassay, the eCG isolated from the serum of mares with a low titer of this hormone had lower amounts of carbohydrates and sialic acid and showed a significantly lower biological response than high-titer serum. The positive influence of sialic acid content on the in vivo biological activity might be due to the eCG residence time in the bloodstream. Cole and Erway [12] noted that the experimental removal of 80% of the sialic acid from the eCG molecule resulted in the disappearance of eCG from the blood within 1 h, whereas intact eCG was still present after 120 h. We can speculate that if preparation II was affected by a partial desialylation or alteration of isoforms, their persistence in the systemic circulation would be insufficient to cause significant changes in ovary development.
The eCG manufacturing process generally consists of fractional precipitation, removal of impurities, pH adjustment, final precipitation in the presence of increasing concentrations of acetone or alcohol and lyophilization before sealing [22]. These steps can introduce contaminants such as residual sanitizing agents, materials that leach from product contact surfaces, and contaminants from the environment of the production area [34]. Although many process-related impurities are routinely monitored, some types of contaminants, such as hormone-related forms, are generally not investigated, especially in the final product. This is because the level of these contaminants in a drug product is often too low to be detected by traditional analytical methods, and does not lead to serious safety concerns [34].
The results of this study demonstrate the extreme care that must be exercised in the quality control of eCG preparations and confirms that changes in the physical–chemical profile of different eCG batches from diverse manufacturers can be related to the large variation in ovarian response observed in domestic animals treated with eCG. Clearly, a single type of physical–chemical analysis cannot predict all aspects of the in vivo biological activity of a hormone. However, there is a growing tendency to substitute in vivo bioassays with physical chemical methods (notably RP-HPLC) to validate methods or batches of manufactured hormones that could complement or even replace the classical in vivo bioassay [16].
In conclusion, RP-HPLC is a rapid and reliable tool for monitoring changes in the physicochemical profile of eCG that are apparently related to the lack of biological activity of this hormone.
Figures and Tables
Fig. 1
Reversed-phase high-performance liquid chromatography (RP-HPLC) analysis. (A) Reference Preparation of eCG from Sigma (13 µg). (B) International Standard of eCG from WHO (50 µg). The amount of protein applied, corresponded nominally to 100 IU.
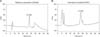
Fig. 2
RP-HPLC analysis of the four commercial preparations of eCG. The amount of eCG protein corresponded nominally to 100 IU for each preparation.
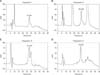
Table 1
Protein content of different equine chorionic gonadotropin (eCG) preparations determined by bicinchoninic acid
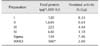
Table 2
Ovarian weight (mean ± SEM) of prepubertal female Wistar rats treated with 1.43 µg protein recovered from one of three RP-HPLC elucted peaks from eCG (Sigma and preparation 1)
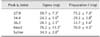
Acknowledgments
The authors thank the staff at the Swine Research Centre (UPD-Tanquinho), Maria de Lourdes Oliveira and Isabel Cristina Rovay Manarin for their assistance with the gilts experiment. This study was funded by FAPESP (grant No. 2011/13096-0).
References
1. Aggarwal BB, Farmer SW, Papkoff H, Seidel GE Jr. Biochemical properties of equine chorionic gonadotropin from two different pools of pregnant mare sera. Biol Reprod. 1980; 23:570–576.


2. Allen WR, Moor RM. The origin of the equine endometrial cups. I. Production of PMSG by fetal trophoblast cells. J Reprod Fertil. 1972; 29:313–316.
3. Almeida BE, Oliveira JE, Carvalho CM, Dalmora SL, Bartolini P, Ribela MTCP. Analysis of human luteinizing hormone and human chorionic gonadotropin preparations of different origins by reversed-phase high-performance liquid chromatography. J Pharm Biomed Anal. 2010; 53:90–97.


4. Almeida BE, Oliveira JE, Damiani R, Dalmora SL, Bartolini P, Ribela MTCP. A pilot study on potency determination of human follicle-stimulating hormone: a comparison between reversed-phase high-performance liquid chromatography method and the in vivo bioassay. J Pharm Biomed Anal. 2011; 54:681–686.


5. Almeida BE, Oliveira JE, Damiani R, Dalmora SL, Bartolini P, Ribela MTCP. Qualitative and quantitative reversed-phase high performance liquid chromatographic analysis of glycoprotein hormones in the presence of a large excess of human serum albumin. J Pharm Biomed Anal. 2012; 63:160–164.


6. Baruselli PS, Reis EL, Marques MO, Nasser LF, Bó GA. The use of hormonal treatments to improve reproductive performance of anestrous beef cattle in tropical climates. Anim Reprod Sci. 2004; 82-83:479–486.


7. Birken S, Berger P, Bidart JM, Weber M, Bristow A, Norman R, Sturgeon K, Stenman UH. Preparation and characterization of new WHO reference reagents for human chorionic gonadotropin and metabolites. Clin Chem. 2003; 49:144–154.


8. Birken S, Maydelman Y, Gawinowicz MA. Preparation and analysis of the common urinary forms of human chorionic gonadotropin. Methods. 2000; 21:3–14.


9. Bó GA, Mapletoft RJ. Historical perspectives and recent research on superovulation in cattle. Theriogenology. 2014; 81:38–48.


10. Ciller UA, McFarlane JD, McFarlane JR. Commercial equine chorionic gonadotrophin isoform composition, immunoactivity, and bioactivity in a murine model using ovarian augmentation and testicular interstitial cell bioassays. Reprod Fertil Dev. 2009; 21:Suppl 9. 111–111.


11. Cole HH, Bigelow M, Finkel J, Rupp GR. Biological half-life of endogenous PMS following hysterectomy and studies on losses in urine and milk. Endocrinology. 1967; 81:927–930.


12. Cole HH, Erway J. 48-hour assay test for equine gonadotropin with results expressed in International Units. Endocrinology. 1941; 29:514–519.


13. Cole HH, Hart GH. The potency of blood serum of mares in progressive stages of pregnancy in effecting the sexual maturity of the immature rat. Am J Physiol. 1930; 93:57–68.


14. Forman RG, Marshall J, Robinson J, Cederholm Williams SA. A comparative analysis of two preparations of human chorionic gonadotrophin. Eur J Obstet Gynecol Reprod Biol. 1992; 46:123–127.


15. Gam LH, Tham SY, Latiff A. Immunoaffinity extraction and tandem mass spectrometric analysis of human chorionic gonadotropin in doping analysis. J Chromatogr B Analyt Technol Biomed Life Sci. 2003; 792:187–196.


16. Garthoff B, Hendriksen C, Bayol A, Goncalves D, Grauer A, de Leeuw R, van Noordwijk J, Pares M, Pirovano R, Rieth M, Ronneberger H, Spieser JM, Storring P, Vosbeck K, Weichert H. Safety and efficacy testing of hormones and related products. The report and recommendations of ECVAM Workshop 9. Altern Lab Anim. 1995; 23:699–712.


17. Gonzalez A, Wang H, Carruthers TD, Murphy BD, Mapletoft RJ. Superovulation in the cow with pregnant mare serum gonadotrophin: effects of dose and antipregnant mare serum gonadotrophin serum. Can Vet J. 1994; 35:158–162.
18. Henderson KM, Weaver A, Wards RL, Lun S, McNatty KP. Comparison of commercial gonadotrophins using bioassays. Proc N Z Soc Anim Prod. 1990; 50:161–165.
19. Huth JR, Norton SE, Lockridge O, Shikone T, Hsueh AJW, Ruddon RW. Bacterial expression and in vitro folding of the β-subunit of human chorionic gonadotropin (hCGβ) and functional assembly of recombinant hCGβ with hCGα. Endocrinology. 1994; 135:911–918.


20. Lecompte F, Roy F, Combarnous Y. International collaborative calibration of a preparation of equine chorionic gonadotrophin (eCG NZY-01) proposed as a new standard. J Reprod Fertil. 1998; 113:145–150.


21. Loureiro RF, de Oliveira JE, Torjesen PA, Bartolini P, Ribela MTCP. Analysis of intact human follicle-stimulating hormone preparations by reversed-phase high-performance liquid chromatography. J Chromatogr A. 2006; 1136:10–18.


22. Lunenfeld B. Historical perspectives in gonadotrophin therapy. Hum Reprod Update. 2004; 10:453–467.


23. Lustbader JW, Wu H, Birken S, Pollak S, Gawinowicz Kolks MA, Pound AM, Austen D, Hendrickson WA, Canfield RE. The expression, characterization, and crystallization of wild-type and selenomethionyl human chorionic gonadotropin. Endocrinology. 1995; 136:640–650.


24. Martinuk SD, Manning AW, Black WD, Murphy BD. Effects of carbohydrates on the pharmacokinetics and biological activity of equine chorionic gonadotrophin in vivo. Biol Reprod. 1991; 45:598–604.


25. McFarlane JR, Czekala NM, Papkoff H. Zebra chorionic gonadotropin: partial purification and characterization. Biol Reprod. 1991; 44:827–833.
26. Murphy BD, Martinuk SD. Equine chorionic gonadotrophin. Endocr Rev. 1991; 12:27–44.
27. Newcomb R, Christie WB, Rowson LEA, Walters DE, Bousfield WED. Influence of dose, repeated treatment and batch of hormone on ovarian response in heifers treated with PMSG. J Reprod Fertil. 1979; 56:113–118.


28. Remmele RL, Krishnan S, Callahan WJ. Development of stable lyophilized protein drug products. Curr Pharm Biotechnol. 2012; 13:471–496.


29. Ribela MTCP, Gout PW, de Oliveira JE, Bartolini P. HPLC analysis of human pituitary hormones for pharmaceutical applications. Curr Pharm Anal. 2006; 2:103–126.


30. Rostami B, Niasari-Naslaji A, Vojgani M, Nikjou D, Amanlou H, Gerami A. Effect of eCG on early resumption of ovarian activity in postpartum dairy cows. Anim Reprod Sci. 2011; 128:100–106.


31. Sá Filho MF, Torres-Júnior JRS, Penteado L, Gimenes LU, Ferreira RM, Ayres H, Castro E, Sales JNS, Baruselli PS. Equine chorionic gonadotropin improves the efficacy of a progestin-based fixed-time artificial insemination protocol in Nelore (Bos indicus) heifers. Anim Reprod Sci. 2010; 118:182–187.


32. Smith PK, Krohn RI, Hermanson GT, Mallia AK, Gartner FH, Provenzano MD, Fujimoto EK, Goeke NM, Olson BJ, Klenk DC. Measurement of protein using bicinchoninic acid. Anal Biochem. 1985; 150:76–86.

