Abstract
Claudins, which are known as transmembrane proteins play an essential role in tight junctions (TJs) to form physical barriers and regulate paracellular transportation. To understand equine diseases, it is helpful to measure the tissue-specific expression of TJs in horses. Major equine diseases such as colic and West Nile cause damage to TJs. In this study, the expression level and distribution of claudin-1, -2, -4, and -5 in eight tissues were assessed by Western blotting and immunohistochemistry methods. Claudin-1 was primarily identified in the lung, duodenum, and uterus, claudin-2 was evenly observed in equine tissues, claudin-4 was abundantly detected in the liver, kidney and uterus, and claudin-5 was strongly expressed in the lung, duodenum, ovary, and uterus, as determined by Western blotting method. The localization of equine claudins was observed by immunohistochemistry methods. These findings provide knowledge regarding the expression patterns and localization of equine claudins, as well as valuable information to understand tight junction-related diseases according to tissue specificity and function of claudins in horses.
Tight junctions (TJs) serve as a barrier to regulate paracellular transportation. These proteins are expressed throughout the body, particularly in epithelial cells. Paracellular or transcellular barriers dynamically maintain homeostasis by regulating the selective exchange of molecules across the epithelial cell sheet barrier, and are essential to the recycling of ions and survival of complex organisms.
Claudins (20–27 kDa) have four transmembrane structures with a short internal N-terminal sequence, two extracellular loops, and an internal C-terminal domain [28]. The first extracellular loop governs paracellular charge selectivity, while the second one serves as a receptor for bacterial toxin [28] and participates in heterogeneous claudin-to-claudin interactions [10]. In epithelial cells and tissues, most TJs contain one or more types of claudins, and form complexes with different claudins [14].
Claudin-1, which is the first identified claudin [58], is crucial to epidermal barrier formation in mammals by forming TJ strands. Claudin-2 decreases the tightness of claudin-based junctional strands in Madin-Darby canine kidney (MDCK I) cells [6], contributes to formation of cation-selective channels, and transforms TJs into leaky junctions [1]. Claudin-4 plays a role in cation-barrier formation and leads to increased transepithelial resistance and decreased Na+ permeability [27]. Claudin-5 is predominantly expressed in endothelial TJs, and is closely associated with vascular diseases [21].
Dysregulation of TJs or genetic defects evokes numerous diseases. Increased paracellular transport of solutes and water occurs in intestinal inflammatory diseases, such as Crohn's disease, ulcerative colitis, and celiac disease, with increased levels of pro-inflammatory cytokines and claudin-2 [18]. Ions and water diffuse from blood to the lumen, resulting in leak-flux diarrhea, a distinct form of diarrhea. Inflammatory bowel diseases are related to the uptake of luminal pathogens, including food antigens and bacterial lipopolysaccharides, due to increased permeability to large molecules [18].
Horses have long been used for transportation, agriculture as well as in numerous competitions ranging from racing to Olympic sports. Hundreds of specialized breeds have been developed. Moreover, humans have also obtained many products from horses including meat, milk, hides, hair, bones, and pharmaceuticals extracted from the urine of pregnant mares. Equus ferus caballus, the domestic horse, is an economically important animal. Common equine diseases are closely related with TJ disorders. For example, West Nile disease, a representative zoonosis between horse and humans, causes decreased expression of claudins in vivo and in vitro [19]. Colonic obstruction, a major etiology of equine colic, is related to TJ deficiency or severe structural changes [12].
The claudin family has different expression patterns, is composed of different TJs, and is associated with various diseases. However, few studies have investigated equine TJs. In the present study, we identified the expression level and distribution of four claudins via Western blotting and immunohistochemistry, respectively, in various equine tissues including those from the liver, kidney, lung, duodenum, heart and male/female reproductive organs.
We obtained equine tissues from slaughterhouse at Jeju island. Eight kinds of tissues were collected from four horses (Table 1), liver, kidney, lung, duodenum, heart, testis, ovary and uterus. All procedures were approved by the Committee on the Ethics of Animal Experiments of the Chungbuk National University (approval No. CBNUA-257-1004-01; approval date 30 November 2010).
To extract cellular protein, tissues were lysed by Pro-prep (catalog No. 17081; iNtRON Biotechnology, Korea), then homogenized by 20 strokes with a Dounce homogenizer, after which samples were centrifuged at 15,300 × g for 20 min at 4℃. Next, 50 µg of protein was loaded onto a 12.5% sodium dodecyl sulfate polyacrylamide gel, then transferred to nitrocellulose membrane (catalog No. IPVH00010; Millipore, USA). The blot was blocked with tris-buffered saline containing 0.5% tween-20 (TBS-T) including 5% skim milk for 1 h at room temperature (RT), then probed with the following primary antibodies at 4℃ overnight: anti-claudin-1 (1 : 1,000; 37-4900; Invitrogen, USA), anti-claudin-2 (1 : 1,000; 51-6100; Invitrogen), anti-claudin-4 (1 : 1,000; 36-4800; Invitrogen), anti-claudin-5 (1 : 1,000; 34-1600; Invitrogen), and anti-GAPDH (1 : 1,000; SC-137179; Santa Cruz Biotechnology, USA). After washing three times with TBS-T, the blots were treated with horseradish peroxidase-conjugated secondary antibody (bs-0296G-HRP; Bioss, USA; mouse IgG 1:3000 or sc-2004; Santa Cruz Biotech; rabbit IgG 1:3000) in TBS-T containing 5% skim milk for 2 h at RT. The blots were subsequently exposed to enhanced chemiluminescence reagent (SC-2048; Santa Cruz Biotech) and developed via GeneGnome5 (MOL5405; Syngene, USA). Signal specificity was confirmed through blotting without primary antibody.
The densities of each band obtained upon western blotting were measured using the NIH Image J software and were normalized to the corresponding density of GAPDH bands. Data are presented as the means ± standard deviation (SD). Groups were compared by one-way analysis of variance (ANOVA) followed by Tukey's multiple comparison test. All statistical analyses were performed using the Prism 6.01 (GraphPad Software, USA). P values < 0.05 were considered to be significant.
Pieces fixed with 10% formalin solution were embedded in paraffin, then cut to 4 µm in thickness and mounted on glass slides. The sections were subsequently deparaffinized with xylene and hydrated in descending graded ethanol solutions. Next, these sections were boiled in basic citrate buffer (pH 9.0) for 5 min to retrieve antigen, treated in TBS-T with 3% hydrogen peroxide for 30 min to block endogenous peroxidase activity, and then incubated with 10% normal goat serum for 1 h to prevent nonspecific reaction. Samples were then probed with primary antibody (1 : 250) at RT overnight, after which they were washed three times with TBS-T. The tissue-sections were then reacted with biotinylated secondary antibodies (rabbit IgG 1 : 500 or BA9200; mouse IgG 1 : 500; catalog No. BA-1000; Vector laboratories, USA) for 1 h at 37℃, washed with TBS-T, and exposed to a VECTASTAIN Elite ABC HRP Kit (catalog No. PK-6100; Vector laboratories) for 1 h at 37℃. After washing three times with TBS-T, sections were exposed to diaminobenzidine (D4293; Sigma, USA), counterstained with hematoxylin, and mounted in Richard-Allan Scientific Cytoseal 60 (REF 8312-4; Thermo Scientific, UK). The specificity of antibodies was confirmed through negative condition without either primary or secondary antibody.
Claudin-1 was strongly expressed in the equine lung, duodenum and uterus when measured by Western blot (panels A and B in Fig. 1; Table 2). Claudin-1 was very low in the kidney, heart and testis compared to other tissues investigated. Immunohistochemical labeling showed that claudin-1 was predominantly present in the epithelia of equine tissues (panels A-J in Fig. 2). Claudin-1 was detected in hepatocytes (panel A in Fig. 2), proximal (panel B in Fig. 2, red arrowhead) and distal (panel B in Fig. 2, black arrowhead) renal tubules, pulmonary bronchiolar (panel D in Fig. 2, red arrowheads) and alveolar cells (panel D in Fig. 2, black arrowheads), and duodenal villi (panel E in Fig. 2). Claudin-1 was also identified in Leydig cells of the testis (panel G in Fig. 2), and theca cells of the ovary (panel H in Fig. 2, red arrowheads). However, it was not detected in cardiac or uterine smooth muscle.
Claudin-2 was expressed in all eight analyzed equine tissues (panels A and C in Fig. 1; Table 2) and was immunohistochemically detected in various cell types. Claudin-2 showed the lowest expression in equine heart, while it was localized in hepatocytes (panel A in Fig. 3), proximal (panel B in Fig. 3, red arrowhead) and distal (panel B in Fig. 3, black arrowhead) renal tubules, pulmonary bronchiolar (panel D in Fig. 3, red arrowhead) and alveolar (panel D in Fig. 3, black arrowhead) cells, duodenal villi (panel E in Fig. 3, red arrowheads) and Brunner's glands (panel E in Fig. 3, black arrowhead), Leydig cells (panel G in Fig. 3, red arrowheads), spermatids (panel G in Fig. 3, black arrowheads), ovarian follicles (panel H in Fig. 3), and uterine glands (panel I in Fig. 3) and myocytes (panel J in Fig. 3). Unlike other members of the claudin family, claudin-2 was detected in Brunner's gland of the duodenum.
Quantification of claudin-4 by Western blotting revealed that it was more abundantly expressed in the liver, kidney, and uterus than other tissues, such as the lung, duodenum, heart, and ovary (panels A and D in Fig. 1; Table 2). The expression level of claudin-4 in the duodenum is lower than that of other claudins and differed between individual horses (panel A in Fig. 1). Similar to other analyzed claudins, claudin-4 was identified in the epithelial cells of tissues including hepatocytes (panel A in Fig. 4), proximal (panel B in Fig. 4, red arrowhead) and distal (panel B in Fig. 4, black arrowhead) renal tubules, duodenal villi (panel E in Fig. 4), Leydig cells (panel G in Fig. 4), and uterine glands (panel I in Fig. 4). Claudin-4 showed the lowest expression in lung tissue.
Claudin-5 was predominantly expressed in the lung, duodenum, ovary, and uterus (panels A and E in Fig. 1; Table 2). Claudin-5 was also detected in all hearts except for that of the old mare (horse C, panel A in Fig. 1). Immunohistochemistry revealed that claudin-5 was detected in all analyzed tissues, and was localized in the bile ductules (panel A in Fig. 5, red arrowheads), proximal (panel B in Fig. 5, red arrowheads) and distal (panel B in Fig. 5, black arrowhead) renal tubules, pulmonary bronchiolar (panel D in Fig. 5, red arrowhead) and alveolar (panel D in Fig. 5, black arrowheads) cells, and duodenal villi (panel E in Fig. 5). It was also expressed in coronary arteries of the heart (panel F in Fig. 5, red arrowheads), Leydig cells of the testis (panel G in Fig. 5), ovarian epithelial cells (panel H in Fig. 5), and uterine glands (panel I in Fig. 5) and myocytes (panel J in Fig. 5, red arrowheads).
Claudins, which were first identified in TJs, consist of four transmembrane domains including a long, first extracellular loop, a highly conserved domain, and a shorter second extracelluar loop [526]. The claudin family consists of 27 members, all of which are expressed between epithelial cells except for claudin-6, -8, -11, and -19. The amino acid sequence homology between the claudin family members is approximately 30% [2021]. Claudin members possess different properties and functions, and are differentially expressed in various tissues. The expression of claudins is regulated by many factors, including hormones, various cytokines, and epithelial-mesenchymal transition (EMT)-related transcription factors.
The liver is a digestive and detoxifying organ that produces bile, which consists of a highly enzymatic and oxidative mixture [2]. Bile flow and cholestasis are regulated by interactions between gap and TJs of hepatocytes [17]. Claudin-1, -2, and -4 were detected in hepatocytes, while claudin-5 was found in bile ductules. Since horses do not have a gall bladder and hepatic bile constantly flows through the duct, observation of claudin-5 in ductules plays an important role as an impermeable barrier to fluid. In this study, claudin-5 protein was expressed in the coronary arteries of the equine heart, while very little was detected in the heart of a 14 years old mare. Claudin-5 is predominantly expressed in endothelial tight junctions such as the vascular endothelium of the blood-brain barrier to form tight junctions that are impermeable to macromolecules [14]. Moreover, claudin-5 functions as a small molecular sieve as indicated by claudin-5 knockout mice showing impaired permeability against particularly small molecules of less than 800 Da though the formation of morphologically normal blood vessels with claudin-12 [23]. Claudin-5 was also shown to be age-dependently reduced in the murine kidney and pancreas [3]. Decreased claudin-5 levels have been reported in the hearts of the dystrophin/utrophin-deficient mouse model and human patients with Duchenne muscular dystrophy and Becker muscular dystrophy, and occurs in the very early step of disease progression with reduction of the physiological and histological indicator (dystrophin) of heart failure [4]. These findings indicate that claudin-5 localized in the artery endothelium can act as an important indicator of coronary artery disease.
Renal tubules are composed of epithelial cells, which serve as barriers against stress caused by blood filtration and urine reabsorption [11]. Claudin-1, -2, -4, and -5 were only expressed in the distal and proximal tubules, not in the glomerulus. Claudin-1, -12 and -16 form water barriers to increase transepithelial resistance (TER) in renal tubules [1618]. claudin-1-deficient mice are born normally, but die within 1 day of birth due to severe dehydration owing to transepidermal water loss [7]. Previous studies have reported that knockout of claudin-2 in mice causes a reduction in the Na+ conductance of the proximal tubule and net reabsorption of Na+, Cl−, and water [22]. It has also been suggested that claudin-2 functions as the paracellular cation pore for passive reabsorption of Na+ in the study using claudin-2 null mice. These null mice also showed hypercalciuria, indicating that claudin-2 plays a role in reabsorption of Ca2+ at the proximal tubule [14]. Western blotting revealed that claudin-4 was more highly expressed in the kidney than in other organs. Claudin-4 acts as a cation-barrier, facilitates NaCl reabsorption [15], and has tightening effect when forming TJs [27]. In the lung, claudin-4 protein was positively expressed by correlating with alveolar fluid clearance [25].
In the testis, all four claudins were observed in Leydig cells and/or the interstitial region, although the distribution patterns were slightly different, with claudin-2 detected in the spermatids. Three claudins were identified in the ovary and uterus upon immunohistochemistry analysis. The female equine organs are anatomically different from those of mice or humans. Specifically, the equine ovary has a reversed structure unlike other animals. The cortex, where follicles are developed, is located in the center of the ovary. Claudin-1 was detected in ovarian follicles, claudin-2 and -5 were found in the epithelium and claudin-4 was not expressed in any horses tested. Claudin-4 is not expressed in healthy ovaries of normal mice [24]. The equine uterus was too big to mount on a slide; therefore, it was divided into two sections; the endometrium and myometrium. In the uterine glands, claudin-2, -4, and -5 were identified along the ciliated columnar epithelium. Claudin-5 was also detected in the uterine veins.
Claudin-1, -2, and -5 were observed in bronchiolar and alveolar cells in the lung, and were found in the epithelium of the duodenal villi. Only claudin-2 was expressed in Brunner's glands of the duodenum. These glands are located in the proximal small intestinal submucosa. Alkaline juice secreted from this gland has a buffering effect against gastric acid [13]. The small intestine of claudin-2 knockout mice shows decreased transepithelial conductance and reduced permeability of Na+ [14]. Moreover, upregulation of claudin-2 is observed in human inflammatory bowel disease such as Crohn's disease, ulcerative colitis and celiac disease, as well as in animal models [18]. Overexpression of claudin-2 induces high-conductance through cation-permeable paracellular pores, then causes leak flux diarrhea due to the increased intestinal ion permeability caused by the disease.
In conclusion, the expression and distribution of four claudins were assessed in several equine tissues. Claudin-1 was strongly expressed in the lung, duodenum, and uterus, which constantly go through cycles of relaxation and contraction. Claudin-2, which is known to decrease TER [9], was evenly observed in the eight equine tissues. Claudin-4 was abundantly detected in the liver, kidney and uterus, but showed weak signals in other tissues. Unlike others, claudin-5 was identified in bile ductules and blood vessels including coronary veins and uterine veins. When compared with murine or human TJs, equine claudins play similar roles, but their tissue-specific distribution was slightly different due to distinct structures of horse organs. These findings provide knowledge about the molecular organization of equine TJs, and a better understanding of TJ-related diseases that are dependent on tissue specificity and functions of claudins in horses.
Figures and Tables
Fig. 1
Protein levels of claudin-1, -2, -4 and -5 in equine tissues. Levels were measured by western blot (A) and were normalized against GAPDH protein (B-E; claudin-1, -2, -4 and -5, respectively). The signal intensity of each band was measured using the NIH Image J software (USA). Data are presented as the means ± standard error of the mean of four equine samples. Liv, Liver; Kid, Kidney; Lu, Lung; Duo, Duodenum; Hrt, Heart; Tes, Testis; Ov, Ovary; Ut, Uterus. *p < 0.01 vs. liver; †p < 0.01 vs. kidney; ‡p < 0.01 vs. lung; §p < 0.01 vs. duodenum; ∥p < 0.01 vs. heart; ¶p < 0.01 vs. ovary; **p < 0.05 vs. uterus.
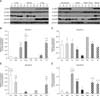
Acknowledgments
This work was supported by the National Research Foundation of Korea (NRF) grant of Korean government (MEST) (No. 2013R1A2A2A05004582).
References
1. Amasheh S, Meiri N, Gitter AH, Schöneberg T, Mankertz J, Schulzke JD, Fromm M. Claudin-2 expression induces cation-selective channels in tight junctions of epithelial cells. J Cell Sci. 2002; 115:4969–4976.


3. D'Souza T, Sherman-Baust CA, Poosala S, Mullin JM, Morin PJ. Age-related changes of claudin expression in mouse liver, kidney, and pancreas. J Gerontol A Biol Sci Med Sci. 2009; 64:1146–1153.
4. Delfín DA, Xu Y, Schill KE, Mays TA, Canan BD, Zang KE, Barnum JA, Janssen PM, Rafael-Fortney JA. Sustaining cardiac claudin-5 levels prevents functional hallmarks of cardiomyopathy in a muscular dystrophy mouse model. Mol Ther. 2012; 20:1378–1383.


5. Furuse M, Fujita K, Hiiragi T, Fujimoto K, Tsukita S. Claudin-1 and -2: novel integral membrane proteins localizing at tight junctions with no sequence similarity to occludin. J Cell Biol. 1998; 141:1539–1550.


6. Furuse M, Furuse K, Sasaki H, Tsukita S. Conversion of zonulae occludentes from tight to leaky strand type by introducing claudin-2 into Madin-Darby canine kidney I cells. J Cell Biol. 2001; 153:263–272.


7. Furuse M, Hata M, Furuse K, Yoshida Y, Haratake A, Sugitani Y, Noda T, Kubo A, Tsukita S. Claudin-based tight junctions are crucial for the mammalian epidermal barrier: a lesson from claudin-1-deficient mice. J Cell Biol. 2002; 156:1099–1111.


8. Furuse M, Hirase T, Itoh M, Nagafuchi A, Yonemura S, Tsukita S, Tsukita S. Occludin: a novel integral membrane protein localizing at tight junctions. J Cell Biol. 1993; 123:1777–1788.


9. Furuse M, Itoh M, Hirase T, Nagafuchi A, Yonemura S, Tsukita S, Tsukita S. Direct association of occludin with ZO-1 and its possible involvement in the localization of occludin at tight junctions. J Cell Biol. 1994; 127:1617–1626.


10. Furuse M, Sasaki H, Tsukita S. Manner of interaction of heterogeneous claudin species within and between tight junction strands. J Cell Biol. 1999; 147:891–903.


11. Gobe GC, Johnson DW. Distal tubular epithelial cells of the kidney: potential support for proximal tubular cell survival after renal injury. Int J Biochem Cell Biol. 2007; 39:1551–1561.


12. Grosche A, Morton AJ, Graham AS, Sanchez LC, Blikslager AT, Polyak MM, Freeman DE. Ultrastructural changes in the equine colonic mucosa after ischaemia and reperfusion. Equine Vet J Suppl. 2011; 8–15.


14. Günzel D, Yu AS. Claudins and the modulation of tight junction permeability. Physiol Rev. 2013; 93:525–569.


15. Hou J, Renigunta A, Yang J, Waldegger S. Claudin-4 forms paracellular chloride channel in the kidney and requires claudin-8 for tight junction localization. Proc Natl Acad Sci U S A. 2010; 107:18010–18015.


16. Hwang I, Yang H, Kang HS, Ahn CH, Lee GS, Hong EJ, An BS, Jeung EB. Spatial expression of claudin family members in various organs of mice. Mol Med Rep. 2014; 9:1806–1812.


17. Kojima T, Yamamoto T, Murata M, Chiba H, Kokai Y, Sawada N. Regulation of the blood-biliary barrier: interaction between gap and tight junctions in hepatocytes. Med Electron Microsc. 2003; 36:157–164.


18. Krug SM, Schulzke JD, Fromm M. Tight junction, selective permeability, and related diseases. Semin Cell Dev Biol. 2014; 36:166–176.


19. Medigeshi GR, Hirsch AJ, Brien JD, Uhrlaub JL, Mason PW, Wiley C, Nikolich-Zugich J, Nelson JA. West Nile virus capsid degradation of claudin proteins disrupts epithelial barrier function. J Virol. 2009; 83:6125–6134.


20. Mitic LL, Van Itallie CM, Anderson JM. Molecular physiology and pathophysiology of tight junctions I. Tight junction structure and function: lessons from mutant animals and proteins. Am J Physiol Gastrointest Liver Physiol. 2000; 279:G250–G254.


21. Morita K, Sasaki H, Furuse M, Tsukita S. Endothelial claudin: claudin-5/TMVCF constitutes tight junction strands in endothelial cells. J Cell Biol. 1999; 147:185–194.
22. Muto S, Hata M, Taniguchi J, Tsuruoka S, Moriwaki K, Saitou M, Furuse K, Sasaki H, Fujimura A, Imai M, Kusano E, Tsukita S, Furuse M. Claudin-2-deficient mice are defective in the leaky and cation-selective paracellular permeability properties of renal proximal tubules. Proc Natl Acad Sci U S A. 2010; 107:8011–8016.


23. Nitta T, Hata M, Gotoh S, Seo Y, Sasaki H, Hashimoto N, Furuse M, Tsukita S. Size-selective loosening of the blood-brain barrier in claudin-5-deficient mice. J Cell Biol. 2003; 161:653–660.


24. Rangel LB, Agarwal R, D'Souza T, Pizer ES, Alò PL, Lancaster WD, Gregoire L, Schwartz DR, Cho KR, Morin PJ. Tight junction proteins claudin-3 and claudin-4 are frequently overexpressed in ovarian cancer but not in ovarian cystadenomas. Clin Cancer Res. 2003; 9:2567–2575.
25. Rokkam D, LaFemina MJ, Lee JW, Matthay MA, Frank JA. Claudin-4 levels are associated with intact alveolar fluid clearance in human lungs. Am J Pathol. 2011; 179:1081–1087.


26. Tsukita S, Furuse M, Itoh M. Multifunctional strands in tight junctions. Nat Rev Mol Cell Biol. 2001; 2:285–293.

