Abstract
During 2006, H5N1 HPAI caused an epizootic in wild birds, resulting in a die-off of Laridae in the Novosibirsk region at Chany Lake. In the present study, we infected common gulls (Larus canus) with a high dose of the H5N1 HPAI virus isolated from a common gull to determine if severe disease could be induced over the 28 day experimental period. Moderate clinical signs including diarrhea, conjunctivitis, respiratory distress and neurological signs were observed in virus-inoculated birds, and 50% died. The most common microscopic lesions observed were necrosis of the pancreas, mild encephalitis, mild myocarditis, liver parenchymal hemorrhages, lymphocytic hepatitis, parabronchi lumen hemorrhages and interstitial pneumonia. High viral titers were shed from the oropharyngeal route and virus was still detected in one bird at 25 days after infection. In the cloaca, the virus was detected sporadically in lower titers. The virus was transmitted to direct contact gulls. Thus, infected gulls can pose a significant risk of H5N1 HPAIV transmission to other wild migratory waterfowl and pose a risk to more susceptible poultry species. These findings have important implications regarding the mode of transmission and potential risks of H5N1 HPAI spread by gulls.
Influenza A virus infections are a significant problem affecting the health of domestic animals and public health [29]. The genetic diversity of avian influenza viruses (AIV) is maintained by their circulation in wild aquatic birds. The highest prevalence of the 16 haemagglutinin (H1–H16) and nine neuraminidase (N1–N9) subtypes was observed in Anseriformes (geese, ducks and swans) and Charadriiformes (gulls, terns and shorebirds) [23]. Many of these species are highly migratory and can transport AIV, including certain highly pathogenic avian influenza viruses (HPAIV) such as H5N1, over long distances, possibly between continents. These viruses, including strains with pandemic potential [1617], are occasionally transmitted to other species such as domestic poultry and mammals [3740]. During 2003–2015, outbreaks of the H5N1 HPAI virus were reported among different types of wild and domestic birds. Despite intensive control, H5N1 HPAI continues to cause major economic problems in affected countries, especially in South-East Asian countries and China. Millions of infected domestic and wild birds have died or been culled throughout the world [2331]. It should be noted that H5N1 HPAI in gulls Laridae has been reported in different countries [27161920222630]. In addition, this strain is considered to be potentially pandemic for humans. Since 2003, the number human cases of avian influenza A (H5N1) reported from 15 countries worldwide is 850, 449 of which were fatal (as of 4 April 2016) [38].
Although multiple experimental trials have been conducted with low pathogenic avian influenza (LPAI) and HPAI viruses in wild birds, most of these have used mallards (Anas platyrhynchos domesticus) and other mallard-type domestic ducks as experimental models [3414212833]. However, the results obtained for mallards cannot be extrapolated to all Anseriformes or any Charadriiformes species. Nevertheless, few experimental studies of Charadriiformes species have been reported [34510111324]. Moreover, the information about susceptibility and viral shedding of AIVs in different host species is very important for understanding virus transmission and maintenance in the wild bird reservoir. It can be used for optimization of sampling methods during the AIV surveillance [7912].
A H5N1 HPAIV, A/common gull/Chany/P/2006 (the intravenous pathogenicity index = 1.7) was isolated from a clinically healthy common gull in Russia in 2006 [27]. Because the common gull (Larus canus) is one of the most numerous species of gulls in Eurasia and has extended habitats, we were interested in better understanding the infectious process in this species.
In the present study we infected common gulls with a higher dose of the same virus we used in our previous study [40] to see if infection accompanied by more severe disease could be induced. We then determined the morbidity, mortality, levels of virus shedding, virus distribution in tissues, microscopic lesions, and transmission to susceptible birds during 28 days after experimental H5N1 HPAIV infection. The results presented herein have important implications regarding mode of transmission and potential risks of H5N1 HPAI spread by gulls.
The Asian lineage (clade 2.2) H5N1 HPAI virus, A/common gull/Chany/P/2006 H5N1 used in this study was obtained from the virus repository at the State Research Center of Virology and Biotechnology, Vector (SRC VB Vector), Federal Service on Customers' Rights Protection and Human Well-being Surveillance, Koltsovo, Novosibirsk region, Russian Federation. Stocks of virus strain were prepared by the second passage in 9-day-old embryonated chicken eggs (ECE) using standard procedures [3239]. Allantoic fluid from the infected eggs was diluted in phosphate-buffered saline to yield a final titer of 107 median egg infectious doses (EID50) per mL.
All activities associated with this study were conducted in accordance with all applicable laws in Russian Federation and the International Guiding Principles for Biomedical Research Involving Animals were followed. All birds used in this study were cared for in accordance with the guidelines approved by the Committee on Biomedical Ethics Research Center of Clinical and Experimental Medicine, Siberian Division of the Russian Academy of Medical Sciences, Novosibirsk (protocol No. 25 from 19.11.2012) and the Use Committee at SRC VB Vector.
Thirty three common gulls (Larus canus) were used in this study. One to 2-day-old nestling gulls were hand-caught in the Chany Lake region (Novosibirsk region, Russian Federation) with the assistance of personnel of the Institute of Systematics and Ecology of Animals. When they were 6-weeks-old, gulls were transported to the Biosafety Level 3 vivarium facilities at the SRC VB Vector for further experiments. All experiments with live viruses were performed in the State Research Center of Virology and Biotechnology certified Biosafety Level 3. Gulls were kept in separate cages and fed ad libitum.
Oropharyngeal and cloacal swabs were collected from each bird before infection to ensure they were not actively infected and shedding avian influenza virus at the start of the study. The absence of AIV was confirmed by real-time reverse transcription polymerase chain reaction (RT-PCR). In addition, pre-infection serum was collected from each bird to confirm by the haemagglutination inhibition test that they were serologically negative to the avian influenza H5N1 virus subtype (A/common gull/Chany/P/2006), as well as to A/teal/Chany/7119/2008 (H15N4) and A/teal/Chany/444/2009 (H8N8) strains isolated earlier from Chany lake, A/mallard/Russia/633/2008 (H4N6) and A/garganey/Altai/1216/2007 (H3N8) strains, which are reportedly widespread in nature, and to A/laridae/Khabarovsk/874/2009 (H13N6) and A/mew gull/Kamchatka/131/2008 (H11N8) strains circulating among Larids [162530]. None of the gulls used in infection experiments were positive for avian influenza type A virus by real-time RT-PCR or had antibodies against the avian influenza subtype tested. We used chickens (Rhode Island ISA brown, 6 weeks old) as infection controls with A/common gull/Chany/P/2006 (H5N1) virus.
In accordance with Ethics statement, birds that showed severe clinical symptoms including neurological signs (muscle tremors, turning on the side), severe weight loss, debilitating diarrhea, and bleeding, were euthanized and counted as dead the next day. All birds were euthanized by cervical dislocation.
We divided 31 gulls into three groups (A, B, C). In addition, two gulls served as negative non-inoculated controls (housed separately). Additionally, 10 chickens (group D) were used for positive control of H5N1 infection and two chickens were used as negative non-inoculated controls (housed separately).
All birds except group C were infected with 107 EID50 per mL/bird dose of A/common gull/Chany/2006 H5N1 HPAI virus applied via mucosa of the nose, oropharynx, and conjunctiva.
Group A: 12 inoculated common gulls were housed in a separate cage for sampling. Cloacal and tracheal swabs were taken on day 2, 4, 6, 8, and 14 after inoculation. Sera were taken on day 7, 14, 21, and 28 post inoculation. Sera from euthanized birds were also collected. All birds were monitored daily for clinical signs of disease or death during 28 days post inoculation. Oropharyngeal and cloacal swabs were taken in 1 mL transport medium with antibiotics. Samples were also taken from bird bath water for real-time RT-PCR analysis during first 7 days post infection of the main bird group A.
Group B: 16 inoculated common gulls were housed in a separate cage for collection of organs. Three gulls were euthanized and sampled at 2, 4, 6, 8, and 14 days post inoculation to evaluate histological lesions and virus presence in the cerebrum, cerebellum, lung, liver, heart, intestine, trachea, and pancreas. On each sampling day, we selected birds that showed the most severe signs of disease.
Group C: On 4 days post inoculation (DPI), three naive common gulls were added into the cage in which the infected gulls of group A were housed. The birds had contact opportunities with shared water, feed, and litter. Oropharyngeal and cloacal swabs of these gulls since the first day after adding were sampled. Only intestinal, lung, tracheal and spleen samples were taken from contact birds. Ten percent tissue suspensions in 0.9% NaCl and aliquots were prepared for virus isolation in chicken embryos.
Group D: Ten infected chickens were housed in separate cages and served as positive controls of viral infection with A/common gull/Chany/2006 (H5N1). Chickens were observed over 10 days and swabs from the cage were taken.
Necropsy and histological analyses: Birds were examined for gross lesions at necropsy and sections of brain, lung, heart, liver, pancreas, and intestine were collected for histopathology. Tissues were fixed in 10% neutral buffered formalin, embedded in paraffin, sectioned at 3 to 5 µm, stained with methyl green-pyronin stain (for detection of plasma cells), Perls prussian blue stain (for detection of hemosiderosis), and hematoxylin and eosin stain, and examined by light microscopy.
Oropharyngeal and cloacal swabs and tissue suspensions were stored at -70℃ after collection until virus isolation and titrations were performed. Virus isolation from tissues was performed in 9-day-old ECE using previously described procedures [3239]. Positive samples were also titrated in 9-day-old ECE. Viral titers were expressed as median egg infectious doses (EID50)/mL. The minimal detectable limit of this assay was 101.7 EID50/mL. Oropharyngeal and cloacal washes were examined by real-time RT-PCR only.
Sera was analyzed for the presence of antibodies by a hemagglutination inhibition (HI) assay using goose and horse erythrocytes as described earlier [3239]. The A/common gull/Chany/P/2006 virus treated with Betapropiolacton (BPL) according to the protocol was used as an antigen. Before use, sera were treated with receptor destroying enzyme (RDE) according to the protocol [32].
Viral RNA was extracted from cloacal and oropharyngeal swabs using Ribo-sorb Kit (SRC SC, Russia) according to the manufacturer's protocols. Tissue samples were macerated in viral transport media and viral RNA was extracted as previously described. Real-time RT-PCR was then performed using published procedures for the detection of H5 influenza isolates [14]. Real-time RT-PCR assays were conducted using reagents provided in the AmpliSense Influenza virus A H5N1-FL AI Viral RNA Isolation kit (SRC SC) on a Rotor-Gene 6000 thermal cycler (Corbett Research, Australia). For each run, samples were prepared and processed in parallel with several negative and positive control samples. Quantification of viral shedding of the H5N1 subtype was performed by comparing Ct values of swab samples with standard curves generated for viral RNA extracted from the initial virus stock.
Diarrhea, an increase of the amount of mucus in the pharynx, and conjunctivitis were observed in virus-inoculated gulls starting at 2 DPI. Behavioral changes, including lethargy and dysorexia, were observed on 3 and 4 DPI. Bird A7 died on day 6 with neurological signs including twitching of the head and falling on the side. Two birds were recumbent, had shortness of breath, anorexia, and severe catarrhal conjunctivitis, and died on 8 DPI. Necropsy revealed emaciation and pneumonia. Birds A4 and A6 showed similar signs of disease, including severe shortness of breath and neurological signs (turning on the side). These birds had a significant reduction in weight, cyanotic throat, the head turned on the side, and incoordination and were therefore euthanized and counted as dead on 18 DPI. Gull A2 showed no significant signs of disease and appeared to be recovering, but at 20 DPI its condition became worse, its head turned to the side, and it developed incoordination; therefore, it was euthanized and counted as dead on 21 DPI. Virus was not detected in the tissues of these birds at this time point. From 10 DPI, all remaining inoculated gulls became visibly active and gained appetite.
No behavioral changes or disease manifestations were observed in contact gulls of group C. As stated above, 6 out of the 12 infected common gulls of group A died (50%). None of the co-housed gulls of group C died during the experiment. As expected, 100% of the positive-control chickens (group D) became sick and died between 4 and 7 dpi (Fig. 1). There were small petechial hemorrhages on the legs of one chicken and diarrhea became more severe in chickens on day 2. Additionally, there was cyanosis of the comb/wattles, and legs, anorexia, pale-colored spleen at necropsy and parenchymal viscera filled with blood when examined on day 3. Later, hemorrhages on the legs and comb and wattles became larger. At necropsy, the following changes were noted: bleeding in the liver, clouding in the pericardium, foci of inflammation in the edematous pancreas, ventriculi with a high content of mucus and bile, swelling on the border between proventriculus and ventriculus, serous inflammation of the intestine and colliquation of the intestinal mucosa.
Viruses were found in tissues from three gulls (A7, A9, A11) that died during the observation period, but were not isolated from gulls A2, A4 and A6, which also died. Viral titers were expressed as a common logarithm of median egg infectious doses in 1 mL (lg EID50/100 µL) of 10% tissue sample suspension.
The influenza virus was found in several tissues from infected gulls euthanized at 2, 4, 6 and 8 DPI, which is suggestive of generalized infection. The virus was isolated at high titers from the brain starting from day 4, and was detected in the brain up to 6 DPI. Most commonly, the virus was detected in the lungs, abdominal air sac and mucosa of the nasal cavity. Except for detection of viruses in the lungs of one bird on 8 DPI, it was detected in parenchymal organs of live birds for up to 6 days after infection. The virus was rarely found in tissues of the digestive system, and was only found at 2 DPI in the small intestine. Additionally, the virus was found more often in the large than in the small intestine. At 14 and 28 DPI, the virus was not detected in tissues of dead or euthanized birds. The virus was also not found in tissue samples collected on 28 DPI from birds of group C.
The data presented in Table 1 suggest that the virus was consistently isolated in high titers from the oropharynx during the first 5 DPI, and during the first 8 DPI in some birds. At the same time, the virus was detected sporadically and in lower titers in cloacal swabs. In oropharyngeal swabs, the viral RNA was detected by real-time RT-PCR at up to 25 DPI. These data confirm the results of our previous study [40].
The virus was also detected in swabs in group C. The virus was found in oropharyngeal swabs from two in contact birds as early as one day post exposure (DPE); however, at 6 DPE, simultaneously with the appearance in cloacal swabs, the virus was also found in a third bird. In group A, the virus was detected in oropharyngeal swabs in higher titers than in cloacal swabs (Table 2). These findings suggest that A/common gull/Chany/2006 (H5N1) influenza virus strain can travel via contact transmission between infected and naive gulls placed in direct contact.
Serum collected from all infected gulls in group A at 7, 14 and 21 DPI showed hemagglutination inhibition, indicating the appearance of antibodies to influenza HA viral antigens at 7 DPI, with a maximum titer at 14 DPI (Table 3). One common gull (A5) did not have antibodies until 28 DPI. Antibodies in the serum of inoculated gulls started to appear at 7 DPI in titers of 1:80 (in one of two gulls euthanized for sampling). On day 7, the antibodies were detected in 11 of 14 gulls with a geometric mean titer of 1:124.35 (range, 1:40–640). On day 14, the geometric mean titer was 1:304.54 (range, 1:80–640) and the antibodies were found in 14 of 15 gulls. On day 21, the titers decreased to the geometric mean of 242.51 (range, 1:80–640) in 10 of 12 gulls (group A).
Microscopic lesions in the euthanized gulls were less necrotizing and more inflammatory than in the birds that died during the study. Sixteen infected common gulls (group B) and two controls were examined at necropsy. The controls had greater amounts of subcutaneous and mesenteric fat than most of the birds that succumbed to infection. The fat reserves in infected gulls were minimal at 4–6 DPI. The most prominent histologic lesions in the infected common gulls were observed in the brain, lungs, pancreas, heart and liver. Examination of the hematoxylin and eosin (HE)-stained sections of the brain revealed damage to the choroid plexus (part of the blood-brain barrier) and ependymal neuroglia at 2–4 DPI, hemorrhages at 2 and 5 DPI, and mild lymphoplasmacytic encephalitis and perivascular cuffing at 4–8 DPI. After 8 DPI, we observed a decrease in histologic lesions in the brain. Examination of methyl green-pyronin (MGP) stained sections of the brain revealed a 10-fold increase of plasma cell number in the brain at 5–8 DPI (panels A–C in Fig. 2). Examination of HE-stained sections of the liver revealed hemorrhages in the parenchyma at 4–8 DPI (panel A in Fig. 3) and multifocal foci of a lymphocytic hepatitis from 2 to 28 DPI (panel B in Fig. 3). Perls prussian blue-stained sections revealed hemosiderosis in the liver at 4–8 DPI (panel C in Fig. 3). Examination of HE-stained sections of lung revealed hemorrhages in the lumen of parabronchi at 2–4 DPI (panel A in Fig. 4), interstitial pneumonia at 4–8 DPI (panel B in Fig. 4) and destruction of the bronchus mucous membrane at 4–6 DPI. However, the histologic lesions disappeared by 8–14 DPI. At 18–28 DPI, we detected secondary bacterial interstitial pneumonia (panel C in Fig. 4). Examination of HE-stained sections of heart revealed mild lymphocytic myocarditis (panel A in Fig. 5) at 4–8 DPI. However, after 8 DPI, we detected a decrease in histologic lesions in the heart. Based on MGP-stained sections, we also observed a 15-fold increase of plasma cell number in the heart at 6–8 DPI.
In addition to the described lesions, at 2–4 DPI, infected gulls had moderate to marked multifocal to confluent necrotizing pancreatitis (panel B in Fig. 5) that was accompanied by heterolymphoplasmacytic serositis at 6–21 DPI (panel C in Fig. 5). No inflammation or necrosis was observed in HE-stained sections of the small intestine.
The representatives of Charadriiformes, particularly those belonging to the Laridae family, play an important role in the ecology of influenza A viruses. Influenza viruses of H16 and H13 subtypes have been shown to be mostly gull-specific (order Charadriiformes) [8]. However, other avian H1, H2, H4, H6, H9, and H11 subtypes have also been isolated from different gulls sporadically. Numerous viruses isolated from gulls and shorebirds have been studied in Russia, including H5N1, H6N1, H11N8, H13N6, H13N2, H13N8 and H16N3 subtypes [19252930]. Fourteen isolates of influenza A viruses of four different subtypes were obtained from wild birds belonging to the Laridae family in the territory of the Russian Far East Region, suggesting that gulls are a natural reservoir for influenza viruses [25]. The susceptibility of Laridae to influenza A virus has been shown in a number of studies [34524]. In addition, gulls involved in H5N1 outbreak with gull-specific viruses can take part in reassortment events that lead to the appearance of novel pathogenic variants [3435]. The common gull is a widespread species of wild bird capable of long-term migrations and having close contact with many wild bird and poultry species [27].
This is one of the few studies to investigate experimental infection of European/Eurasian gull species using highly pathogenic influenza H5N1 virus [34510111524]. In this study, we concentrated on different widespread Siberian species, the common gull and the H5N1 virus previously isolated from this species. This model was selected because common gulls (Larus canus) are one of the most numerous species of gulls in Eurasia and have extended habitats. Moreover, in 2006, a H5N1 HPAIV, A/common gull/Chany/P/2006, was isolated from a clinically healthy common gull in Russia [27]. We suggested that this species could play an active role in H5N1 spread during 2005–2009 epizootics in Russia.
A number of factors have to be considered when evaluating the risk of transportation and H5N1 HPAIV transmission with gulls in Eurasia and the virus spread across the continent. In our studies, we attempted to reproduce natural infection of wild birds of aquatic and semi-aquatic origin through application of the virus via mucosae of the nasal passage, conjunctiva and mouth cavity. The A/common gull/Chany/P/2006 (H5N1) HPAI virus was isolated from Larus canus without visible signs of infection. Thus, reproduction of infection in the natural host is of the utmost interest. A high degree of susceptibility of this species to the investigated strain of influenza virus was demonstrated [40]. However, during epizootics in wild birds, die-off of Laridae was also observed; therefore, we used a higher dose of the virus in the present study to see if we could reproduce this effect.
It is well known that birds of Laridae family can eat carrion and obtain a high dose of virus with their feed through the consumption of dead birds when the epizootics occur [45]. In the present study, we used a dose of 107 EID50. Six of twelve infected gulls (50%) died in response to treatment with this dose. The death of birds occurred from 6 DPI and the specificity of deaths was confirmed up to 8 DPI (three of the dead birds died during the first 8 days and the virus was found in the tissues). However, the death of birds during the early stages after infection was probably caused by heart and lung damage as evidenced by serious changes revealed during histological evaluation.
The virus was not found in tissues of birds that died during later periods, suggesting that birds died as a result of bacterial co-infection (bacterial interstitial pneumonia was found in three birds), or that the influenza virus induced irreversible visceral changes that disturbed organ function and caused emaciation.
Our results show that the most apparent microscopic lesions observed in infected common gulls in this study were necrosis of the pancreas, mild encephalitis, mild myocarditis, liver parenchymal hemorrhages, multifocal foci of a lymphocytic hepatitis, parabronchi lumen hemorrhages and interstitial pneumonia. This finding is especially intriguing because these HPAI viruses-induced lesions have been demonstrated in multiple avian species and in response to various HPAI viruses [181824303435]. We detected virus by isolation in the cerebrum, cerebellum, nasal septum, lung, abdominal air sac, trachea, esophagus, liver, heart, kidney, spleen, bursal sac and colon of infected gulls at 2–8 DPI. These findings illustrate that A/common gull/Chany/2006 (H5N1) strain can cause generalized infection in gulls. The isolation of H5N1 virus from oropharyngeal and cloacal swabs over 25 days indicates that replication of the investigated virus also likely occurred within the upper respiratory and enteric tracts.
More continuous and stable viral shedding in high titers (during 5 days and up to 8 days in certain birds) was registered in oropharyngeal swabs, probably because of the higher infectious dose applied. However, in cloaca, the virus was detected sporadically and in lower concentrations. In oropharyngeal swabs, the virus was found for up to 25 days inclusive (the period of observation was 28 days). Thus, infected gulls in our experiment shed a significant amount of virus through the oropharynx and a much lesser amount through the cloaca. These findings are consistent with data from studies of other wild bird species [4561125]. Thus, infected common gulls can pose a significant risk of H5N1 HPAIV transmission to other wild migratory waterfowl and pose a risk to other, more susceptible species.
The results of the current study showed that all gulls added into cages became infected. In addition, virus shedding from the oropharynx occurred as early as the first day after addition into the cage. These findings suggest that common gulls can actively participate in the spread of highly pathogenic avian influenza virus during migration. If an infected bird survives and sheds virus it means that, in large populations of migratory gulls, a significant number of infected birds have the potential to spread viruses across Eurasia and other continents. Thus, it is important to determine if infected common gulls can migrate after infection with H5N1 HPAIV, and if so, how far. Infected gulls may still be able to fly and could therefore spread the virus during viral shedding; however, additional studies are needed confirm this.
The results of this study will enable investigation of the role of other gull species. Studies to identify the level and duration of viral shedding, mechanisms of transmission, survival, and, eventually, the potential risk of influenza infection in these important species are critical. Cases of influenza H5N1 are regularly reported in animals, particularly in birds of the Laridae family, which makes further investigation of the gull's role in influenza A virus ecology a problem of current concern. Additional studies are needed to further evaluate the susceptibility of other gull species and other birds to different strains of avian influenza H5N1 virus.
Figures and Tables
Fig. 2
(A) Damage of the blood-brain barrier at 2 days post infection (DPI). (B) Mild lymphoplasmacytic encephalitis at 8 DPI. (C) Increasing plasmacytes number in brain at 8 DPI. H&E stain (A and B) and methyl green-pyronin stain (C). 40× (A–C).
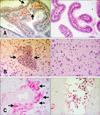
Fig. 3
(A) Bleeding in the liver parenchyma at 5 DPI. (B) Locus of a lymphocytic hepatitis at 2 DPI. (C) Hemosiderosis in the liver at 8 DPI. H&E stain (A and B) and Perl's Prussian blue stain (C). 40× (A–C).
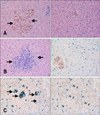
Fig. 4
(A) Hemorrhages in a lumen of parabronchi at 2 DPI. (B) Interstitial pneumonia at 6 DPI. (C) Secondary bacterial interstitial pneumonia at 18 DPI. H&E stain. 20× (A–C).
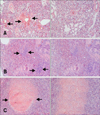
Fig. 5
(A) Mild lymphocytic myocarditis at 4 DPI. (B) Necrotizing pancreatitis at 4 DPI. (C) Heterolymphoplasmacytic serositis of the pancreas at 8 DPI. H&E stain. 40× (A–C).
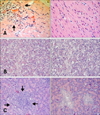
Table 1
Oropharyngeal shedding of H5N1 virus by experimentally infected common gulls (group A) based on real-time reverse transcription-polymerase chain reaction (RT-PCR)
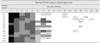
Acknowledgments
This study was supported by USDA grant No. 58-0210-2-040F and the Ministry of Education and Science of the Russian Federation (project No. RFMEFI61315X0045). The authors thank to Eugeny Sherstkov for technical assistance with the histological experiments.
References
1. Abdullazyanova ER, Zajkovsmaya AV, Shestopalova LV, Shestopalov AM. Study of pathogenetic features infectious caused by highly pathogenic influenza virus A/H5N1 in wild ducks and domestic chickens. Bulletin ESSC SB RAMS. 2012; 5:163–165.
3. Bahl AK, Pomeroy BS. Experimental exposure of Franklins' gulls (Larvus pipixcan) and mallards (Anas platyrhynchos) to a turkey influenza A virus A/Turkey/Minn/BF/72 (Hav6Neq2). J Wildl Dis. 1977; 13:420–426.


4. Brown JD, Stallknecht DE, Beck JR, Suarez DL, Swayne DE. Susceptibility of North American ducks and gulls to H5N1 highly pathogenic avian influenza viruses. Emerg Infect Dis. 2006; 12:1663–1670.


5. Brown JD, Stallknecht DE, Swayne DE. Experimental infections of herring gulls (Larus argentatus) with H5N1 highly pathogenic avian influenza viruses by intranasal inoculation of virus and ingestion of virus-infected chicken meat. Avian Pathol. 2008; 37:393–397.


6. Costa TP, Brown JD, Howerth EW, Stallknecht DE. Variation in viral shedding patterns between different wild bird species infected experimentally with low-pathogenicity avian influenza viruses that originated from wild birds. Avian Pathol. 2011; 40:119–124.


7. Ellis TM, Bousfield RB, Bissett LA, Dyrting KC, Luk GSM, Tsim ST, Sturm-Ramirez K, Webster RG, Guan Y, Malik Peiris JS. Investigation of outbreaks of highly pathogenic H5N1 avian influenza in waterfowl and wild birds in Hong Kong in late 2002. Avian Pathol. 2004; 33:492–505.


8. Fouchier RAM, Munster V, Wallensten A, Bestebroer TM, Herfst S, Smith D, Rimmelzwaan GF, Olsen B, Osterhaus ADME. Characterization of a novel influenza A virus hemagglutinin subtype (H16) obtained from black-headed gulls. J Virol. 2005; 79:2814–2822.


9. Gibbs SE. Avian biology, the human influence on global avian influenza transmission, and performing surveillance in wild birds. Anim Health Res Rev. 2010; 11:35–41.


10. Hall JS, Franson JC, Gill RE, Meteyer CU, TeSlaa JL, Nashold S, Dusek RJ, Ip HS. Experimental challenge and pathology of highly pathogenic avian influenza virus H5N1 in dunlin (Calidris alpina), an intercontinental migrant shorebird species. Influenza Other Respir Viruses. 2011; 5:365–372.


11. Hall JS, Krauss S, Franson JC, TeSlaa JL, Nashold SW, Stallknecht DE, Webby RJ, Webster RG. Avian influenza in shorebirds: experimental infection of ruddy turnstones (Arenaria interpres) with avian influenza virus. Influenza Other Respir Viruses. 2013; 7:85–92.


12. Hanson BA, Luttrell MP, Goekjian VH, Niles L, Swayne DE, Senne DA, Stallknecht DE. Is the occurrence of avian influenza virus in Charadriiformes species and location dependent? J Wildl Dis. 2008; 44:351–361.


13. Höfle U, Van de Bildt MWG, Leijten LM, Van Amerongen G, Verhagen JH, Fouchier RAM, Osterhaus ADME, Kuiken T. Tissue tropism and pathology of natural influenza virus infection in black-headed gulls (Chroicocephalus ridibundus). Avian Pathol. 2012; 41:547–553.


14. Jeong OM, Kim MC, Kim MJ, Kang HM, Kim HR, Kim YJ, Joh SJ, Kwon JH, Lee YJ. Experimental infection of chickens, ducks and quails with the highly pathogenic H5N1 avian influenza virus. J Vet Sci. 2009; 10:53–60.


15. Kawaoka Y, Krauss S, Webster RG. Avian-to-human transmission of the PB1 gene of influenza A viruses in the 1957 and 1968 pandemics. J Virol. 1989; 63:4603–4608.


16. Li KS, Guan Y, Wang J, Smith GJD, Xu KM, Duan L, Rahardjo AP, Puthavathana P, Buranathai C, Nguyen TD, Estoepangestie ATS, Chaisingh A, Auewarakul P, Long HT, Hanh NTH, Webby RJ, Poon LLM, Chen H, Shortridge KF, Yuen KY, Webster RG, Peiris JSM. Genesis of a highly pathogenic and potentially pandemic H5N1 influenza virus in eastern Asia. Nature. 2004; 430:209–213.


17. Lipatov AS, Krauss S, Guan Y, Peiris M, Rehg JE, Perez DR, Webster RG. Neurovirulence in mice of H5N1 influenza virus genotypes isolated from Hong Kong poultry in 2001. J Virol. 2003; 77:3816–3823.


18. Makarova NV, Kaverin NV, Krauss S, Senne D, Webster RG. Transmission of Eurasian avian H2 influenza virus to shorebirds in North America. J Gen Virol. 1999; 80:3167–3171.


19. Marchenko VIu, Sharshov KA, Silko N, Susloparov IM, Durymanov AG, Zaĭkovskala AV, Alekseev A, Smolovskaia OV, Stefanenko AP, Malkova EM, Shestopalov AM. Characterization of the H5N1 influenza virus isolated during an outbreak among wild birds in Russia (Tuva Republic) in 2010. Mol Gen Mikrobiol Virusol. 2011; 36–40.


20. Marchenko VY, Alekseev AY, Sharshov KA, Petrov VN, Silko NY, Susloparov IM, Tserennorov D, Otgonbaatar D, Savchenko IA, Shestopalov AM. Ecology of influenza virus in wild bird populations in Central Asia. Avian Dis. 2012; 56:234–237.


21. Mundt E, Gay L, Jones L, Saavedra G, Tompkins SM, Tripp RA. Replication and pathogenesis associated with H5N1, H5N2, and H5N3 low-pathogenic avian influenza virus infection in chickens and ducks. Arch Virol. 2009; 154:1241–1248.


22. Normile D. Avian influenza. Potentially more lethal variant hits migratory birds in China. Science. 2005; 309:231.


23. Olsen B, Munster VJ, Wallensten A, Waldenström J, Osterhaus AD, Fouchier RA. Global patterns of influenza A virus in wild birds. Science. 2006; 312:384–388.


24. Perkins LE, Swayne DE. Susceptibility of laughing gulls (Larus atricilla) to H5N1 and H5N3 highly pathogenic avian influenza viruses. Avian Dis. 2002; 46:877–885.


25. Sayfutdinova SG, Sharshov KA, Gerasimov YN, Shestopalov AM. Ecology of influenza A viruses, isolated from gulls of the Russian Far East. Bulletin ESSC SB RAMS. 2012; 5:316–318.
26. Sharshov K, Silko N, Sousloparov I, Zaykovskaya A, Shestopalov A, Drozdov I. Avian influenza (H5N1) outbreak among wild birds, Russia, 2009. Emerg Infect Dis. 2010; 2:349–351.


27. Sharshov KA, Kurskaia OG, Zaĭkovskaia AV, Iurlov AK, Susloparov IM, Ternovoĭ VA, Durymanov AG, Zolotykh SI, Shestopalov AM, Drozdov IG. Characteristic of high pathogenic avian influenza virus subtype H5N1 isolated from common gull (Larus canus). Zh Mikrobiol Epidemiol Immunobiol. 2010; 29–32.
28. Shestopalova LV, Shkurupiy VA, Sharkova TV, Shestopalov AM. Morphological changes in bird viscera in experimental infection by highly pathogenic H5N1 avian influenza virus. Bull Exp Biol Med. 2008; 146:770–773.


29. Sivay MV, Baranovich T, Marchenko VY, Sharshov KA, Govorkova EA, Shestopalov AM, Webby RJ. Influenza A (H15N4) virus isolation in Western Siberia, Russia. J Virol. 2013; 87:3578–3582.


30. Sivay MV, Sayfutdinova SG, Sharshov KA, Alekseev AY, Yurlov AK, Runstadler J, Shestopalov AM. Surveillance of influenza A virus in wild birds in the Asian portion of Russia in 2008. Avian Dis. 2012; 56:456–463.


31. Suzuki K, Okada H, Itoh T, Tada T, Mase M, Nakamura K, Kubo M, Tsukamoto K. Association of increased pathogenicity of Asian H5N1 highly pathogenic avian influenza viruses in chickens with highly efficient viral replication accompanied by early destruction of innate immune responses. J Virol. 2009; 83:7475–7486.


32. Swayne DE, Glisson JR, Jackwood MW, Pearson JE, Reed WM. A Laboratory Manual for the Isolation and Identification of Avian Pathogens. 4th ed. Chester County: American Association of Avian Pathologists;2006. p. 74–80. p. 150–163. p. 235–240.
33. Teifke JP, Klopfleisch R, Globig A, Starick E, Hoffmann B, Wolf PU, Beer M, Mettenleiter TC, Harder TC. Pathology of natural infections by H5N1 highly pathogenic avian influenza virus in mute (Cygnus olor) and whooper (Cygnus cygnus) swans. Vet Pathol. 2007; 44:137–143.


34. Van Borm S, Rosseel T, Vangeluwe D, Vandenbussche F, van den Berg T, Lambrecht B. Phylogeographic analysis of avian influenza viruses isolated from Charadriiformes in Belgium confirms intercontinental reassortment in gulls. Arch Virol. 2012; 157:1509–1522.


35. Velarde R, Calvin SE, Ojkic D, Barker IK, Nagy E. Avian influenza virus H13 circulating in ring-billed gulls (Larus delawarensis) in southern Ontario, Canada. Avian Dis. 2010; 54:1 Suppl. 411–419.


37. Webster RG, Bean WJ, Gorman OT, Chambers TM, Kawaoka Y. Evolution and ecology of influenza A viruses. Microbiol Rev. 1992; 56:152–179.


38. World Health Organization. Cumulative number of confirmed human cases of avian influenza A (H5N1) reported to WHO, 2003-2013. Geneva: World Health Organization;2013.
39. World Health Organization. WHO global influenza surveillance network: manual for the laboratory diagnosis and virological surveillance of influenza. Geneva: World Health Organization;2011. p. 79–82.
40. Zaĭkovskaia AV, Sharshov KA, Sherstkov EA, Iurlov AK, Shestopalov AM. Experimental infection caused by influenza A (H5N1) virus in common gull (Larus canus). Vopr Virusol. 2012; 57:43–46.