Abstract
Thirty-four dogs with no deep pain perception due to acute thoracolumbar intervertebral disc disease underwent decompression surgery within 1 week of diagnosis. All dogs underwent hemilaminectomy. Adipose derived mesenchymal stem cells (AD-MSCs) were transplanted into the injured spinal cord parenchyma for the AD-MSCs transplant dogs. Long-term outcome was evaluated at the end of the follow-up period (> 6 months). AD-MSCs combination treatment showed better recovery outcomes compared to decompression surgery alone. These results indicate that this stem cell therapy is a potential therapeutic strategy to overcome the limitations of treatment for spinal cord injury in clinical medicine.
Thoracolumbar intervertebral disc disease (IVDD) is a common disorder in dogs, mainly middle-aged-dogs of chondrodystrophic breeds [1]. Clinical signs range from mild back pain to hind limb paraplegia. Neurologic dysfunction is classified into grades 1 to 5 corresponding to the degree of spinal cord damage [14]. Previous research studies indicated that dogs in grade 1 to 4 had good outcomes after medical or surgical treatment, and that dogs in grade 5 with no deep pain perception (DPP) and severe spinal cord injury showed some improvement after the surgical treatment [4610]. Approximately 60% of decompression surgeries had a successful outcome, but there was little evidence of successful outcome from the medical treatment [1].
Functional recovery is highly related to the degree of injury of the spinal cord, which is determined by the velocity of disc extrusions, mass of extruded material, ratio of the spinal canal and cord diameter, duration of neurological deficits, and existence of DPP [4811]. The absence of DPP is the primary factor that causes poor clinical outcome. DPP pathways are located bilaterally in the deep part of the spinal cord. The nonexistence of DPP suggests that there is severe spinal cord damage.
Cell transplantation therapy using adult stem cells has recently been identified as a potential therapeutic modality in spinal cord injury [579]. The results of these studies indicated that transplanted mesenchymal stem cells (MSCs) produce several neurotropic factors and are differentiated into various neural cells. Moreover, these findings demonstrated the improvement of motor function in spinal cord injured animal models.
The purpose of this study was to improve treatment of dogs diagnosed as having thoracolumbar disc disease with no DPP. We demonstrated the comparative clinical outcomes from two treatments: decompression surgery alone and the combination of decompression surgery along with transplantation of allogenic adipose derived mesenchymal stem cells (AD-MSCs) to the injured spinal cord.
This research was approved by the Institutional Animal Care and Use Committee of Seoul National University (SNU-130807-1). All dog owners agreed to and signed the informed consent form. Medical records of dogs examined at the Seoul National University Veterinary Teaching Hospital from April 2006 to July 2014 were reviewed. Dogs were included in the study if they had a history of acute onset (< 24 h) paraplegia with no DPP resulting from thoracolumbar disc disease and were operated on within 1 week. Dogs with vertebral fracture or luxation and myelomalacia were excluded from the study. For all dogs included in the study, physical and neurological examinations were performed at the time of hospital admission. Acute thoracolumbar disc disease was confirmed on the basis of magnetic resonance imaging or computed tomography (Fig. 1). The degree of neurologic dysfunction of each dog was classified as follows [14]: grade 1, signs of pain only with no abnormal neurologic signs; grade 2, ambulatory paraparesis; grade 3, nonambulatory paraparesis; grade 4, paraplegia with deep pain perception; and grade 5, paraplegia and no deep pain perception. Dogs assessed as clinically normal were assigned a grade of 0.
AD-MSCs of dog hip fat were isolated and characterized as previously described [9]. Briefly, adipose tissues were washed with phosphate buffered saline (PBS), then digested with collagenase type I with intermittent shaking. The suspension was filtered through a 100 mm nylon mesh and centrifuged to separate floating adipocytes from stromal cells. Preadipocytes in the stromal vascular fraction were plated in T175 flasks at 8,000 to 10,000 cells/cm2 overnight in Dulbecco's modified Eagle medium (Gibco-BRL, USA) supplemented with 1% penicillin and streptomycin and 10% fetal bovine serum at 37℃ in an atmosphere of 5% humidified CO2. Unattached cells and residual non-adherent red blood cells were removed after 24 h by washing with PBS, then replaced with fresh cell medium.
The dogs were medicated and anesthetized with tramadol (4 mg/kg, intravenously; Samsung Pharm, Korea), hydromorphone (0.05 mg/kg, intravenously; Hana Pharm, Korea), propofol (6 mg/kg, intravenously; Claris Lifesciences, Australia) and atropine sulfate (0.05 mg/kg, subcutaneously; Jeil Pharmaceutical, Korea). Anesthesia was maintained with isoflurane (Choongwae Pharmaceutical, Korea) at 1.5 minimum alveolar concentration (MAC) throughout the procedure.
A standard hemilaminectomy was performed, and extruded disc material was removed [12]. Methylprednisolone sodium succinate (30 mg/kg, intravenously, once; Kunhwa Pharmaceutical, Korea) was administered immediately prior to the initial surgical incision. For the allogenic AD-MSCs transplant dogs, a suspension of 1 × 107 AD-MSCs in 150 µL of PBS was transplanted into the injured spinal cord parenchyma through the dura before closing the operation site. A 30 gauge spinal needle was used to inject the cells at all three locations (the middle of the injury site and the proximal and distal margins). Postoperative management included bladder management, nursing care to prevent decubital ulcers, cage rest, physical rehabilitation therapies such as passive range of motion, electro-acupuncture and therapeutic laser therapy. Dogs received electro-acupuncture treatment twice per week until motor function recovery. Therapeutic laser therapy was conducted once a week after the motor function of the dogs returned.
Outcome was evaluated at the end of the follow-up period by review of medical records for the long-term (> 6 months) outcome. The neurological outcome was classified as full recovery (normal neurologic state; grade 0), improved (regained DPP and recovery of ambulation, but still had mild ataxia; grade 1–2) and unsuccessful (did not regain DPP or the ability to walk without support; grade 3–5). The full recovery and improved outcomes were considered clinically successful.
Statistical analysis was performed using a commercially available statistical software program (SPSS ver. 21.0; IBM, USA). A Chi Squared or Fischer's exact test was used to compare outcomes and the nonparametric Mann-Whitney U test was used to compare recovery times between groups. A p < 0.05 was considered significant.
One hundred forty five operation case records were reviewed, 34 of which met the criteria for inclusion in the study. The decompression surgery (DSX) group consisted of 25 dogs (18 males and 7 females) that included 8 Dachshunds, 7 Cocker Spaniels, 3 Pekingese, 3 Maltese, 2 Beagles, 1 Poodle and 1 Pug. Their average age was 5.67 ± 2.85 (mean ± SD) and their average body weight was 8.04 ± 3.06 kg. The main region of the affected disc spaces was T10/11 (n = 1), T11/12 (n = 2), T12/13 (n = 6), T13/L1 (n = 6), L1/2 (n = 3), L2/3 (n = 4), L3/4 (n = 1) and L4/5 (n = 2). The AD-MSCs transplant (AD-MSCs) group consisted of 9 dogs (5 males and 4 females) that included 6 Dachshunds, 2 Poodles and 1 Cocker spaniel. The average age of this group was 5.32 ± 1.87, and the average body weight was 7.62 ± 3.70 kg. The main region of affected disc spaces was T11/12 (n = 2), T12/13 (n = 2), T13/L1 (n = 2), L1/2 (n = 1), L2/3 (n = 1) and L3/4 (n = 1).
According to the clinical outcomes, the success rate of the AD-MSCs group was 77.8% (7/9), which was significantly higher than that of the DSX group [52.0%, (13/25)] (p < 0.05). The results of the AD-MSCs group revealed that 55.6% of the dogs were in full recovery, 22.2% showed improved outcomes and 22.2% had unsuccessful recovery. However, the results for the DSX group were 16.0%, 36.0% and 48.0%, respectively (Table 1). The recovery time required to achieve grade 4 was 5.55 ± 3.56 days and 2.57 ± 1.51 days for the DSX and AD-MSCs groups, respectively. The time needed to achieve grade 3 was 11.92 ± 6.97 days and 10.50 ± 8.96 days, respectively. The time to grade 2 was 36.67 ± 25.95 days and 25.67 ± 13.37 days, respectively. The full recovery time in the DSX and AD-MSCs group was 62.25 ± 27.48 days and 59.67 ± 4.73 days, respectively (Fig. 2).
After physical and mechanical trauma, the spinal cord undergoes direct cell death and hemorrhage in response to the initial mechanical impact. Vascular disruption promotes ischemia, microvascular damage, glutamatergic excitotoxicity, oxidative stress and inflammation [513]. These inflammatory environments operate to change astrocytes into reactive astrocytes in the spinal cord. The reactive astrocytes also produce pro-inflammatory cytokines and other signaling molecules that can lead to upregulation of inhibitory molecules, so that these inflammatory processes and demyelinated axons lead to axonal degeneration. Following these acute pathological changes, the proteoglycans, which are connective tissue elements and glial cells such as reactive astrocytes and oligodendrocytes, were cross linked to form the glial scar [3]. This glial scar tissue mechanically obstructs the extension of regenerative axons.
MSCs, which can be isolated from various sources, display multipotency and differentiate into a variety of cell lineages such as chondrocytes, adipocytes, and osteoblasts. Current data suggests that MSCs may not only replace diseased tissues, but that they also exert several trophic, regenerative and anti-inflammatory effects [2]. According to our previous study, there is a multiplicity of interventions to promote recovery through modification of the spinal cord injury mechanisms [79]. Transplanted MSCs directly decrease the factors related to inflammation such as interleukin (IL)-1, IL-6, cyclooxygenase-2 and tumor necrosis factor-α, then limit the inflammation cascade. MSCs also modify the inflammatory environment, and these events accompany decreased neuronal cell death, stimulating axonal growth, promoting new growth of guidance molecules and blocking molecules that inhibit regeneration. In addition, decreased astrogliosis related signal molecules such as phosphorylated signal transducer and activator of transcription and increased neuronal regeneration markers including Tuj-1, nestin, microtubule associated protein 2, and neurofilament M provide evidence of regulating signal molecules related to astrogliosis and neuronal extension.
A relatively low number of cases of DPP loss are caused by IVDD [410]. We investigated 34 cases that met this criteria. However, it should be noted that this study is limited by this small number of patients.
In conclusion, our results showed good outcomes of decompression surgery combined with AD-MSCs transplantation compared to decompression surgery alone in dogs with no DPP. Improved clinical signs after transplantation of MSCs are primarily attributed to a modified inflammatory environment and enhancement of the survival of endogenous nerve cells. Moreover, transplanted MSCs reduce regulatory signal molecules related to glial scar formation and can partially differentiate into neural cells. Our results demonstrated that AD-MSCs therapy was a potential therapeutic strategy to overcome the limitations of treatment for spinal cord injury in clinical medicine.
Figures and Tables
Fig. 1
Transverse T2-weighted magnetic resonance image (A) and transverse computed tomograph image (B) of dogs with intervertebral disc disease. Extruded disc materials (white arrow in A, black arrow in B) compressed the spinal cord.
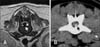
Fig. 2
Days (mean ± SD) recovered to each grade in 34 dogs with acute intervertebral disc disease and absence of deep pain perception. n, number of recovered cases; DSX, decompression surgery alone; AD-MSCs, combination of decompression surgery and the transplantation of adipose derived mesenchymal stem cells.
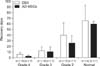
Acknowledgments
This work was partially supported by the Research Institute for Veterinary Science, Seoul National University, and the National Research Foundation of Korea (NRF-2013R1A1A2004506).
References
1. Davis GJ, Brown DC. Prognostic indicators for time to ambulation after surgical decompression in nonambulatory dogs with acute thoracolumbar disk extrusions: 112 cases. Vet Surg. 2002; 31:513–518.


2. Figueroa FE, Carrión F, Villanueva S, Khoury M. Mesenchymal stem cell treatment for autoimmune diseases: a critical review. Biol Res. 2012; 45:269–277.


3. Fitch MT, Silver J. CNS injury, glial scars, and inflammation: inhibitory extracellular matrices and regeneration failure. Exp Neurol. 2008; 209:294–301.


4. Laitinen OM, Puerto DA. Surgical decompression in dogs with thoracolumbar intervertebral disc disease and loss of deep pain perception: a retrospective study of 46 cases. Acta Vet Scand. 2005; 46:79–85.
5. Nandoe Tewarie RD, Hurtado A, Levi AD, Grotenhuis JA, Oudega M. Bone marrow stromal cells for repair of the spinal cord: towards clinical application. Cell Transplant. 2006; 15:563–577.


6. Olby N, Levine J, Harris T, Muñana K, Skeen T, Sharp N. Long-term functional outcome of dogs with severe injuries of the thoracolumbar spinal cord: 87 cases (1996-2001). J Am Vet Med Assoc. 2003; 222:762–769.


7. Park SS, Byeon YE, Ryu HH, Kang BJ, Kim Y, Kim WH, Kang KS, Han HJ, Kweon OK. Comparison of canine umbilical cord blood-derived mesenchymal stem cell transplantation times: Involvement of astrogliosis, inflammation, intracellular actin cytoskeleton pathways, and neurotrophin-3. Cell Transplant. 2011; 20:1867–1880.


8. Penning V, Platt SR, Dennis R, Cappello R, Adams V. Association of spinal cord compression seen on magnetic resonance imaging with clinical outcome in 67 dogs with thoracolumbar intervertebral disc extrusion. J Small Anim Pract. 2006; 47:644–650.


9. Ryu HH, Lim JH, Byeon YE, Park JR, Seo MS, Lee YW, Kim WH, Kang KS, Kweon OK. Functional recovery and neural differentiation after transplantation of allogenic adipose-derived stem cells in a canine model of acute spinal cord injury. J Vet Sci. 2009; 10:273–284.


10. Scott HW, McKee WM. Laminectomy for 34 dogs with thoracolumbar intervertebral disc disease and loss of deep pain perception. J Small Anim Pract. 1999; 40:417–422.


11. Seim HB 3rd. Conditions of the thoracolumbar spine. Semin Vet Med Surg (Small Anim). 1996; 11:235–253.


12. Slatter DH. Textbook of Small Animall Surgery. 3rd ed. Philadelphia: Elsevier Health Science;2003. p. 1206–1208.
13. Young W. Secondary injury mechanisms in acute spinal cord injury. J Emerg Med. 1993; 11:Suppl 1. 13–22.