Abstract
The purpose of the present study was to establish normal electroretinogram (ERG) parameters using 56 normal eyes of four dog breeds common in Thailand: poodle, Labrador retriever, Thai ridgeback, and Thai Bangkaew. Standard ERG findings were bilaterally recorded using a handheld multi-species ERG unit with an ERG-jet lens electrode for 28 dogs under preanesthesia with diazepam, anesthesia with propofol, and anesthesia maintenance with isoflurane. There were significant differences in the mean values of ERG amplitudes and implicit times among the four dog breeds (p < 0.05) except for the b-wave implicit time of the photopic 30 Hz flicker response with 3 cd.s/m2 (p = 0.610). Out of the four breeds, Thai Bangkaew had the longest implicit time (p < 0.001) of scotopic low intensity responses, b-wave of scotopic standard intensity responses (3 cd.s/m2), a-wave of the higher intensity response (10 cd.s/m2), and a-wave of the photopic single flash response (3 cd.s/m2). For the b/a ratio, only the ratio of the Cone response was significantly different among the different breeds. In this summary, normal ERG parameters for four dog breeds were reported. Data from the investigation supported the hypothesis that determination of breed-specific limits of normality for ERG responses is necessary for individual clinics and laboratories.
Flash electroretinogram (ERG) enables the evaluation of changes in electrical potential that originate in the retina where the eye is stimulated by light [3]. This technique is useful for the early diagnosis and prognosis of inherited progressive retinal atrophy (PRA) or characterizing retinopathy due to other causes. ERG is also tremendously useful when performed before cataract surgery as well as diagnosing specific blinding disorders in dogs such as sudden acquired retinal degeneration from optic neuritis or cortical blindness [21].
There are numerous factors that can distort the accuracy and reliability of an ERG recording. Many researchers have focused on different factors including the stage of retinal adaptation [29,33], electrode type and position [18], anesthesia technique [11,14,22]; the age, and breeds of the animal [7,10]; the environment and body temperature [19], pupil diameter [17], eye movement [20], intraocular pressure [6], diabetes [27], condition of the anterior segment, cataract stage, lens-induced uveitis [15], and the effects of hypercapnia [31]. Moreover, environmental illumination, excessive ambient electrical noise, intensity of the flash, retinal conditions, quality of the recording equipment, and viscosity of the solution used between the contact lens electrode and cornea are additional important factors that impact ERG parameters [3,8,12,17]. Thus, each laboratory or clinic should establish technical procedures that provide reproducible ERG results under specific conditions in order to use the data for diagnostic purposes.
ERG parameters of a few dog breeds have been previously reported. Normal ERG parameters of 15 healthy beagles were established using an ERG contact lens electrode with built-in diode light sources (LED-electrode) [16]. Itoh et al. [10] found that the ERG amplitudes for healthy Shi-Tzus are lower than those for beagles after stimulation with the same light intensities. Therefore, it is important to determine normal ERG values for each dog breed when using ERG for making diagnoses. The actual cause of breed-related differences in ERG recordings is not fully understood.
Four dog breeds, poodle, Labrador retriever, Thai ridgeback, and Thai Bangkaew, are common in Thailand. Poodles and Labrador retrievers are predisposed to hereditary retinal degeneration [30,34] and cataracts [24,25]. The Thai ridgeback and Thai Bangkaew have been recognized by the Kennel Club of Thailand as the national dogs of Thailand. In 1993, the Federation Cynologique Internationale (FCI) registered the Thai ridgeback as a pure-breed dog and Thai Bangkaew was similarly registered in 2011. ERG parameters for these four dog breeds have never been studied. Therefore, the objective of the present study was to establish normal ERG parameters for poodles, Labrador retrievers, Thai Bangkaew, and Thai ridgebacks between 2 to 8 years old which was the time of fully complete function of the retina and before aging degeneration [7] using the HMsERG system with a contact lens electrode. Additionally, differences in ERG recordings among these breeds were assessed.
Both eyes of 28 ophthalmoscopically normal, healthy dogs of four different breeds (nine poodles, six Labrador retrievers, eight Thai ridgebacks, and five Thai Bangkaew) were included in the study. These dogs were privately owned by different owners in the central part of Thailand. The mean age of all dogs was 4.64 ± 0.29 years (mean ± standard error [SE]) and ranged from 2 to 8 years as shown in Table 1. There was no significant difference among the average ages of each breed according to a one-way ANOVA. All dogs were housed and food was given in different quantities by the owners at their individual houses. The animals were considered to be in good health with no history of visual deficit or ocular abnormality. Vision was evaluated by the perfect scotopic/photopic obstacle test and positive menace response. Dazzle and pupillary light reflexes were tested in all dogs. The anterior segment was considered normal based on results of an ocular examination with a portable slit lamp biomicroscope (SL-15; Kowa Optimed, Japan). Intraocular pressure was measured using a tonometer (TonoVet; ICare Finland, Finland). Fundus examination was conducted using a binocular indirect ophthalmoscope (Vantage; Keeler Ophthalmic Instruments, UK) in a dark room after ERG examination.
Each dog underwent a physical examination. A complete blood cell count and standard biochemistry profile were also obtained. Food and water were withheld for 10 h before the test. The dogs were kept in a quiet, dimly lit room for 1 h before performing the ERG procedure. Prior to anesthesia, the pupils of both eyes were maximally dilated by applying one drop of 1% tropicamide (Midriacyl; Alcon-Couvreur, Belgium) twice with a 15-min interval between the drops. Pupil size was periodically evaluated to ensure full dilation, especially at the beginning and end of ERG recording. Animals were premedicated with 0.3 mg/kg diazepam (GPO, Thailand) delivered intravenously. Induction of anesthesia was performed with 4 mg/kg propofol (Anepol; Hana Pharm, Korea) administered intravenously. The dogs were endotracheally intubated and inhalation was maintained with 2.0% isoflurane (Aerrane; Baxter International, USA). Anesthesia was induced under ambient light. The depth of anesthesia was kept constant during the procedure. Proper oxygenation and ventilation of the anesthetized dog were maintained throughout ERG recording via intubation. The body temperature was monitored and maintained at 38~39℃ by a rectal thermometer every 10 min.
Each anesthetized dog was placed in a sternal recumbent position on a wooden table with the head on a towel (Fig. 1). Topical anesthetic eye drops (0.5% tetracaine hydrochloride ophthalmic solution; Alcon) were applied. Both eyelids were opened widely with a lid speculum (Barraquer eye speculum; Elcon Medical Instruments, Germany) and a conjunctival stay suture was applied to obtain proper globe position. A corneal electrode (ERG-jet; Fabrinal, Switzerland) was positioned with artificial tear gel (Methocel 2%; Omni Vision, Germany) applied between the corneal surface and contact lens. Skin needle electrodes were used as the reference and ground electrodes. The reference electrode was placed approximately 3 cm caudal to the lateral canthi while the ground electrode was positioned over the external occipital protuberance. All electrodes were connected to a preamplifier, and the signals were amplified with a bandpass filter of the HMsERG between 0.3 and 300 Hz (Xenotec, USA). Placement of the electrodes was performed in a room with ambient lighting. The mini-Ganzfeld was positioned as close to the eye as possible without touching the dog.
Before the ERG results were recorded, impedance was measured. Baseline tests were also performed to evaluate noise levels in the environment, and ensure proper contact between electrodes and tissues. Lights in the examining room were then turned off at the commencement of the first session of the scotopic ERG procedure (dark adaptation). ERG results were recorded automatically using the standard protocol, Dog Diagnostic, that was a part of the software package for the HMsERG unit and recommended by the European College of Veterinary Ophthalmologists [4]. According to a user manual for the HMsERG instrument, model 1000, retinal signals were obtained under scotopic and photopic conditions for a total of 33.81 min. For scotopic ERG procedures, three different responses were recorded: scotopic low intensity responses using 0.01 cd.s/m2 of light stimuli during 20 min of dark adaptation, an average of 10 flashes with an interval of 2 seconds for every 4 min to evaluate rod function designated Rod1, Rod2, Rod3, Rod4, and Rod5; scotopic standard intensity responses (Std R&C) using 3 cd.s/m2 of light intensity for stimulus, and scotopic higher intensity responses (Hi-int R&C) using 10 cd.s/m2 of light intensity to stimulate both rods and cones. The photopic ERG procedure measured two different responses: a photopic single flash response (Cone) using 3 cd.s/m2 of light after 10 min of light adaptation with 30 cd.s/m2 of background light to assess the cone, and a photopic 30 Hz flicker response (Flicker) using 3 cd.s/m2 for cone evaluation while in the light adapted state. ERG data for each dog were recorded in the right eye followed by the left eye.
ERG waveforms were analyzed by measuring a- and b-wave amplitudes and implicit times as well as the b/a ratio. The a-wave amplitude was measured from the baseline to the trough of the first negative peak while the b-wave amplitude was measured from the trough of the a-wave to the peak of the next positive wave. The a- and b-wave implicit times were calculated from the onset of the light stimulus to the peak of the respective waves. For the scotopic low intensity responses and photopic 30 Hz flicker responses, only the b-wave amplitude and implicit time were measured. All reference ERG values were defined as the median value and range between the 5th and 95th percentiles (lower and upper limits). To compare the mean amplitudes, implicit times, and b/a ratios of the four dog breeds, statistical analyses were performed using NCSS 8 [23]. P values less than or equal to 0.05 were considered significant. A Bonferroni test as a post-hoc test of the one-way ANOVA was performed when the data had a normal distribution. Kruskal-Wallis tests together with Dunn's test were used when the data was not normally distributed and/or variances were not equal.
The ophthalmic examination and blood test results for all dogs were normal. ERG outputs from the 56 eyes of the 28 dogs were successfully recorded using a portable ERG machine. Impedance of the active and reference electrodes was less than 5 kΩ in each eye of all subjects. Anesthetic maintenance with isoflurane inhalation provided good immobilization, and had sufficient duration for light stimulation and recording.
Normal ERG waveforms for each dog breed were compared (Fig. 2). The a-wave amplitude was undetectable for scotopic low intensity responses in all dogs as expected. The b-wave amplitudes of the scotopic low intensity response steadily increased after 4, 8, 12, 16, and 20 min of light stimulation (panels A-E in Fig. 2). ERG waveforms of the scotopic standard and high intensity responses were similar (panels F-G in Fig. 2) with the ERG a- and b- waves clearly identified. The small a- and b-waves were detectable in the photopic single flash response while only the b-wave was detectable in the photopic flicker response. The median and limits of normality for the ERG parameters using the 5th and 95th percentiles of all recordings are summarized in Tables 2 and 3 according to the guidelines for clinical electroretinography in dogs [4].
Mean values and standard error (SE) of the amplitudes, implicit times, and b/a ratios obtained for the four breeds are summarized in Figs. 3, 4, and 5, respectively. For the amplitudes, there were significant differences in all parameters: Rod1 (p = 0.002), Rod2 (p < 0.001), Rod3 (p = 0.006), Rod4 (p = 0.001), Rod5 (p = 0.002), a-wave of Std R&C (p = 0.024), b-wave of Std R&C (p = 0.014), a-wave of Hi-int R&C (p = 0.029), b-wave of Hi-int R&C (p = 0.01), a-wave of Cone (p = 0.023), b-wave of Cone (p < 0.001), and Flicker (p = 0.011). For the implicit time, a significant difference was observed for all parameters except Flicker (p = 0.610). Significant differences among the implicit times were observed for Rod1 (p < 0.001), Rod2 (p < 0.001), Rod3 (p < 0.001), Rod4 (p < 0.001), Rod5 (p < 0.001), a-wave of Std R&C (p = 0.003), b-wave of Std R&C (p < 0.001), a-wave of Hi-int R&C (p = 0.004), b-wave of Hi-int R&C (p = 0.002), a-wave of Cone (p < 0.001), and b-wave of Cone (p = 0.04).
For all standard low intensity responses, the Thai Ridgeback had higher b-wave amplitudes than the Labrador retriever. Poodles had higher b-wave amplitudes than Labrador retrievers for all responses except for the Rod responses. There was no significant difference among amplitudes for all responses when comparing the Thai ridgeback and Thai Bangkaew. Compared to all breeds, the Thai Bangkaew had a more prolonged implicit time for the b-waves of Rod1, Rod3, Rod4 and Std R&C waves, including for a-waves of Hi-int R&C and Cone (Fig. 4). Additionally, only the b/a ratio of the Cone response was significantly different (p = 0.035) among the dog breeds.
In this study, normal ERG parameters for four dog breeds were identified. These findings are supported by data from an earlier study of different ERG parameters for different breeds [10]. In the present investigation, factors associated with dog age, examination environment, anesthesia protocol, and equipment were standardized as much as possible so that breed-related factors were the main variable. The actual cause of differences in ERG recordings for each breed is not fully understood. It was speculated that resistance and voltage of the ERG signal vary widely due to a large variation in skull conformation [4]. The skull width of poodles is less than half the length of the head. The entire skull appears oval when seen from above and the side appears slightly convex. Additionally, the axes of the poodle skull and muzzle are slightly divergent. The Labrador retriever has a broad skull. The brow is pronounced and the ridges of the brow giving the stop a defined look. The cheeks are shallow. The muzzle and skull are approximately the same length. The Thai Bangkaew has a wedge-shaped skull and a medium-length muzzle with a broad base that tapers towards a black nose. Finally, the Thai ridgeback has a skull that is flat between the ears but appears slightly rounded from the side. The skull is slightly longer than the wedge-shaped muzzle. These various skull conformations might be the cause for breed-specific differences in ERG parameters.
The Thai Bangkaew had a significantly prolonged ERG implicit time compared to the other breeds. In general, a prolonged implicit time of the b-wave amplitude concurrent with a low amplitude indicates retinal degeneration in canines [9]. However, all Thai Bangkaew dogs in the present study had normal ocular appearance and vision, and the a- and b-wave amplitudes of these animals were not considered low when compared to those of the other breeds. Therefore, prolongation of the implicit time should be expected as an actual reference parameter for the Thai Bangkaew.
The eyes of puppies open 10~15 days after birth. No ERG can be performed until the animal is 2 weeks old. However, at this age an ERG result can only be recorded as a small negative wave that does not exceed 5 µV. From 5~8 weeks of age, the photoreceptor segments differentiate and other retinal layers develop [7]. Different ERG variables might be caused by ocular media alterations that decrease the effective intensity of the stimulus while decreasing photoreceptor density and bipolar or Müller cell death [1,2,32]. Age of the subjects recruited for the present study ranged from 2 to 8 years (4.64 ± 0.29 yr) at which time development of the retina has been completed and the late-onset form of PRA can occur.
The Dog Diagnostic protocol is a standard ERG procedure in veterinary medicine [21]. This technique was established based on the standard ERG protocol used for humans. It is a complex, diagnostic procedure that provides complete information about photoreceptor function separately, which is needed for detecting subtle changes in rod and cone function especially in cases of hereditary retinal degeneration [4].
General anesthesia using various anesthetic agents and protocols affects ERG recordings. Previous studies have shown that diazepam alters retinal function solely by affecting amacrine-ganglion cell interactions [5,26,28]. Propofol has been found to have little effect on gamma aminobutyric acid. The effect of this compound on the retina is significant when administered as a continuous infusion. Increasing the propofol infusion rate elevates the b-wave amplitude, while a decreased rate produces a parallel reduction in the b-wave amplitude of normal beagles [13]. Isoflurane has been found to decrease the amplitude of the a- and b-waves compared to tiletaminezolazepam in dark-adapted dogs [14]. In addition, isoflurane administration results in slightly shorter implicit times for higher flash intensity stimulation in rats [20]. Therefore, different effects of various anesthetic agents should be considered when interpreting ERG parameters.
In conclusion, normal ERG parameters were obtained for four dog breeds common in Thailand with an HMsERG. Significant differences in the parameters were identified and described. All processes including the anesthesia protocols were safe and appropriate for long periods of ERG recording. Findings from this investigation demonstrated that normal ERG values for each dog breed should be obtained before interpreting ERG data.
Figures and Tables
Fig. 1
Photograph of the electroretinogram (ERG) setup using a handheld multi-species electroretinograph with a 5-year-old Thai Bangkaew.
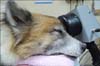
Fig. 2
Comparison of normal ERG traces for the four dog breeds: scotopic low intensity responses (Rod1~Rod5) observed 4, 8, 12, 16, and 20 min after dark conditions (A~E); scotopic standard intensity responses (Std R&C; F), higher intensity responses (Hi-int R&C; G), photopic single flash responses (Cone; H), and photopic flicker responses (Flicker; I). The flash onset stimulus is indicated by arrows.
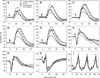
Fig. 3
Mean and standard error (SE) of amplitudes recorded for the four dog breeds. Letters a, b, and c indicate significant differences among the breeds. ERG amplitudes of all responses were significantly different among the breeds (p < 0.05).

Fig. 4
Mean and SE of implicit times recorded for the four dog breeds. Letters a, b, and c indicate significant differences among the breeds (p < 0.05). Implicit times of all responses were significantly different among the breeds except for Flicker (p = 0.610).

Fig. 5
Mean and SE of the b/a ratio recorded for the four dog breeds. Letters above the columns (a and b) indicate significant differences among the breeds. There was no statistical difference in the b/a ratio of the Std R&C (p = 0.476) and Hi-int R&C (p = 0.214) responses. A significant difference (p = 0.035) was observed for the b/a ratio of the Cone.

Acknowledgments
This research was supported by the Center of Excellence on Agricultural Biotechnology, Science and Technology Postgraduate Education and Research Development Office, Commission on Higher Education, Ministry of Education (AG-BIO/PERDO-CHE) and the Kasetsart University Research and Development Institute (KURDI), Thailand.
References
1. Birch DG, Anderson JL. Standardized full-field electroretinography. Normal values and their variation with age. Arch Ophthalmol. 1992; 110:1571–1576.
2. Curcio CA, Millican CL, Allen KA, Kalina RE. Aging of the human photoreceptor mosaic: evidence for selective vulnerability of rods in central retina. Invest Ophthalmol Vis Sci. 1993; 34:3278–3296.
3. Ekesten B. Ophthalmic examination and diagnostics. In : Gelatt KN, editor. Veterinary Ophthalmology. 4th ed. Oxford: Wiley;2007. 1:p. 520–535.
4. Ekesten B, Komáromy AM, Ofri R, Petersen-Jones SM, Narfström K. Guidelines of clinical electroretinography in the dog: 2012 update. Doc Ophthalmol. 2013; 127:79–87.


6. Grozdanic SD, Kecova H, Harper MM, Nilaweera W, Kuehn MH, Kardon RH. Functional and structural changes in a canine model of hereditary primary angle-closure glaucoma. Invest Ophthalmol Vis Sci. 2010; 51:255–263.


7. Gum GG, Gelatt KN, Samuelson DA. Maturation of the retina of the canine neonate as determined by electroretinography and histology. Am J Vet Res. 1984; 45:1166–1171.
8. dos Santos Hansho C, Oria AP, da Veiga Monteiro Lazaro Júnior LP, Neto FD, Laus JL. The organization of flash electroretinography unit in veterinary medicine. Cienc Rural. 2004; 34:1097–1104.


9. Hertil E, Bergström T, Kell U, Karlstam L, Ekman S, Ekesten B. Retinal degeneration in nine Swedish Jämthund dogs. Vet Ophthalmol. 2010; 13:110–116.


10. Itoh Y, Maehara S, Yamasaki A, Tsuzuki K, Izumisawa Y. Investigation of fellow eye of unilateral retinal detachment in Shih-Tzu. Vet Ophthalmol. 2010; 13:289–293.


11. Jeong MB, Narfström K, Park SA, Chae JM, Seo KM. Comparison of the effects of three different combinations of general anesthetics on the electroretinogram of dogs. Doc Ophthalmol. 2009; 119:79–88.


12. Komáromy AM, Brooks DE, Dawson WW, Källberg ME, Ollivier FJ, Ofri R. Technical issues in electrodiagnositic recording. Vet Ophthalmol. 2002; 5:85–91.
13. Kommonen B, Hyvätti E, Dawson WW. Propofol modulates inner retina function in Beagles. Vet Ophthalmol. 2007; 10:76–80.


14. Lin SL, Shiu WC, Liu PC, Cheng FP, Lin YC, Wang WS. The effects of different anesthetic agents on short electroretinography protocol in dogs. J Vet Med Sci. 2009; 71:763–768.


15. Maehara S, Itoh N, Wakaiki S, Yamasaki A, Tsuzuki K, Izumisawa Y. The effects of cataract stage, lens-induced uveitis and cataract removal on ERG in dogs with cataract. Vet Ophthalmol. 2007; 10:308–312.


16. Maehara S, Itoh N, Itoh Y, Wakaiki S, Tsuzuki K, Seno T, Kushiro T, Yamashita K, Izumisawa Y, Kotani T. Electroretinography using contact lens electrode with built-in light source in dogs. J Vet Med Sci. 2005; 67:509–514.


17. Marmor MF, Fulton AB, Holder GE, Miyake Y, Brigell M, Bach M. ISCEV Standard for full-field clinical electroretinography (2008 update). Doc Ophthalmol. 2009; 118:69–77.


18. Mentzer AE, Eifler DM, Montiani-Ferreira F, Tuntivanich N, Forcier JQ, Petersen-Jones SM. Influence of recording electrode type and reference electrode position on the canine electroretinogram. Doc Ophthalmol. 2005; 111:95–106.


19. Mizota A, Adachi-Usami E. Effect of body temperature on electroretinogram of mice. Invest Ophthalmol Vis Sci. 2002; 43:3754–3757.
20. Nair G, Kim M, Nagaoka T, Olson DE, Thulé PM, Pardue MT, Duong TQ. Effects of common anesthetics on eye movement and electroretinogram. Doc Ophthalmol. 2011; 122:163–176.


21. Narfstrom K. Electroretinography in veterinary medicine - easy or accurate? Vet Ophthalmol. 2002; 5:249–251.


22. Norman JC, Narfström K, Barrett PM. The effects of medetomidine hydrochloride on the electroretinogram of normal dogs. Vet Ophthalmol. 2008; 11:299–305.


23. Obasi CN, Barrett B, Brown R, Vrtis R, Barlow S, Muller D, Gern J. Detection of viral and bacterial pathogens in acute respiratory infections. J Infect. 2014; 68:125–130.


24. Ofri R. Retina. In : Maggs DJ, Miller PE, Ofri R, editors. Slatter's Fundamentals of Veterinary Ophthalmology. 4th ed. St. Louis: Saunders;2008. p. 285–295.
25. Park SA, Yi NY, Jeong MB, Kim WT, Kim SE, Chae JM, Seo KM. Clinical manifestations of cataracts in small breed dogs. Vet Ophthalmol. 2009; 12:205–210.


26. Robbins J, Ikeda H. Benzodiazepines and the mammalian retina. I. Autorediographic localisation of receptor sites and the lack effect on the electroretinogram. Brain Res. 1989; 479:313–322.


27. Safatle AMV, Hvenegaard AP, Otsuki D, Martins TL, Kahvegian M, Berezovsky A, Salomão SR, Barros PSM. Comparison of full-field electroretinogram in diabetic and non diabetic dogs with cataracts. Pesqui Vet Bras. 2010; 30:1071–1076.


28. Stafanous SN, Clarke MP, Ashton H, Mitchell KW. The effect of long-term use of benzodiazepines on the eye and retina. Doc Ophthalmol. 1999; 99:55–68.
29. Tuntivanich N, Mentzer AL, Eifler DM, Montiani-Ferreira F, Forcier JQ, Johnson CA, Petersen-Jones SM. Assessment of dark-adaptation time required for recovery of electroretinographic responses in dogs after fundus photography and indirect ophthalmoscopy. Am J Vet Res. 2005; 66:1798–1804.


30. Petersen-Jones SM. A review of research to elucidate the causes of the generalized progressive retinal atrophies. Vet J. 1998; 155:5–18.


31. Varela Lopez O, Alvarez Vazquez JC, Gonzalez Cantalapiedra A, Rosolen SG. Effect of hypercapnia on the electroretinogram in sevoflurane and isoflurane anaesthetized dogs. Doc Ophthalmol. 2010; 121:9–20.


32. Weleber RG. The effect of age on human cone and rod ganzfeld electroretinograms. Invest Ophthalmol Vis Sci. 1981; 20:392–399.
33. Yanase J, Ogawa H, Ohtsuka H. Rod and cone components in the dog electroretinogram during and after dark adaptation. J Vet Med Sci. 1995; 57:877–881.


34. Zangerl B, Goldstein O, Philp AR, Lindauer SJP, Pearce-Kelling SE, Mullins RF, Graphodatsky AS, Ripoll D, Felix JS, Stone EM, Acland GM, Aguirre GD. Identical mutation in a novel retinal gene causes progressive rod-cone degeneration in dogs and retinitis pigmentosa in humans. Genomics. 2006; 88:551–563.

