Abstract
This study was conducted to measure the concentrations of strontium (Sr), barium (Ba), cadmium (Cd), copper (Cu), zinc (Zn), manganese (Mn), chromium (Cr), antimony (Sb), selenium (Se), and lead (Pb) in canine liver, renal cortex, and renal medulla, and the association of these concentrations with age, gender, and occurrence of chronic kidney disease (CKD). Tissues from 50 dogs were analyzed using inductively coupled plasma mass spectrometry. Cu, Zn, and Mn levels were highest in the liver followed by the renal cortex and renal medulla. The highest Sr, Cd, and Se concentrations were measured in the renal cortex while lower levels were found in the renal medulla and liver. Female dogs had higher tissue concentrations of Sr (liver and renal medulla), Cd (liver), Zn (liver and renal cortex), Cr (liver, renal cortex, and renal medulla), and Pb (liver) than male animals. Except for Mn and Sb, age-dependent variations were observed for all element concentrations in the canine tissues. Hepatic Cd and Cr concentrations were higher in dogs with CKD. In conclusion, the present results provide new knowledge about the storage of specific elements in canine liver and kidneys, and can be considered important reference data for diagnostic methods and further investigations.
High concentrations of trace elements, alkaline earth metals, heavy metals, and metalloids in the organs of animals can be considered an indication of environmental pollution and the amounts of these elements in feed. In the present study, we measured the concentrations of strontium (Sr), barium (Ba), cadmium (Cd), copper (Cu), zinc (Zn), manganese (Mn), chromium (Cr), antimony (Sb), selenium (Se), and lead (Pb) in the liver and kidneys of dogs. Up to now, little has been known about the retention of these elements in dogs. However, studies in humans or other species demonstrated that levels of these elements in the organism depend upon exposure or intake, and can vary in age- and gender-depend manners.
Radioactive 90Sr was released by overhead nuclear weapon tests in the 1950s and 1960s, and this element is mainly incorporated by an organism via ingestion of contaminated food. 90Sr is particularly incorporated in the skeleton. The long half-life and associated high retention of this isotope in bones lead to a permanent radiation burden in the organism [8].
Comparable to Sr, Ba is also preferentially stored in bones. After an intravenous injection of 133Ba2+ into dogs [7], the highest specific activity was found in the sternum followed by the sacral bone, vertebral column, ribs, upper arm, and upper leg. A study in rats demonstrated that Ba was almost completely absorbed in the gastrointestinal tract of young animals, while the absorption rate decreased with age [34]. In humans, Ba accumulation was found to increase with age and predominantly accumulated in the lungs, aorta, kidneys, and spleen [31]. Children had higher concentrations in the aorta, heart, kidneys, liver, spleen, and muscles compared to adults.
Cd is one of the most important environmental contaminants. Cd concentrations in the soil depend on the use of mineral-based (e.g., phosphate) fertilizers as well as contamination with industrial air pollution or sewage sludge. Cd can either be incorporated through the lungs or gastrointestinal tract. Intestinal absorption of Cd depends on several factors such as the chemical binding form, the animal species or breed, biochemical reactions of Cd within the gastrointestinal tract, the age, health, and metabolic activity of the animal, feed composition, and the amount of synergistic or antagonistic compounds in the feed [13]. The absorbed Cd persists or is only partly eliminated, and stored in the organism in various organs such as the liver and kidneys. Cd concentrations therefore increase in the storage organs over time. Under stressful conditions, Cd can be partly remobilized, resulting in signs of intoxication. Long-lasting intake can cause kidney damage accompanied by mineral metabolism disorders. In addition, hepatic dysfunction or changes in the bone structure can appear.
Pb is another important environmental contaminant. Depending on environmental pollution, varying Pb concentrations can be found in foods derived from plants and animals, resulting in variations of Pb uptake [5]. Since it has been assumed that non-dietary factors are of minor importance for overall Pb exposure in Europe [6], measurement of Pb accumulation in organs might provide not only important reference data for clinical diagnostics but also an indication of Pb exposure of dogs through food.
Cu is an essential trace element and serves as a biocatalyst within an organism. Important functions include hematopoiesis, the development of bones and connective tissue, keratinization of hairs, pigmentation of hair and skin, myelination of nerves in the spinal marrow, reproduction, and growth [23]. The primary storage organ for Cu is the liver. Thus, hepatic Cu concentrations can be an indicator of Cu intake via feed and Cu levels in the animal.
Similar to Cu, Zn is also an essential trace element. It is particularly important for the immune system, skin, hair, and reproduction [23]. In humans, Zn status can be measured in the ribs, hair, serum, and milk [12]. In dogs and cats, excessive Zn intake leads to increased Zn concentrations in the liver, kidneys, and bones while discontinuing Zn supplementation results in the normalization of Zn levels [4]. Another study showed that a diet with low Zn concentrations results in reduced Zn concentrations in the plasma and liver of cats [15]. An interaction between Cd and Zn was demonstrated in dogs when Cd supplementation resulted in increased Zn concentrations in the kidneys, liver, and sternum [35].
Se plays an important role in many functions in the organism including antioxidation, muscle activity, and reproduction [21]. A deficiency in dietary Se and vitamin E has been reported to result in anorexia, depression, muscular weakness, dyspnea, subcutaneous edema, and coma in dogs [37]. Se toxicosis has been experimentally induced in dogs [26,38,40]. However, no clinical cases resulting from high dietary Se intake have been reported up to now, possibly due to the low bioavailability of Se in commercial dog foods [36]. It has been demonstrated that Se absorption in the gastrointestinal and respiratory tract depends on the chemical form of the element while excess Se is mainly excreted by the kidneys [36,39].
Mn is primarily absorbed in the gastrointestinal tract [22] while pulmonary intake is of minor importance. A study in humans demonstrated that erythrocytes incorporate small amounts of 54Mn while higher concentrations are found in the liver, kidneys, and pancreas [20]. Another study was performed to evaluate whether specific organs could be used to determine the Mn status in animals [2]. The authors found that Mn deficiency in goats could be identified by analyzing the liver. In cattle and sheep, Mn status can be assessed based on concentrations of the element in the liver, kidneys, hair, and cerebrum.
Cr is an essential trace element as a trivalent compound while the tetravalent compounds act as carcinogens. Cr (III) is important for glucose and lipid metabolism as well as protein synthesis [24]. Little is known about the metabolism of Cr. The element is absorbed in the gastrointestinal tract when metalliferous food and drinking water are ingested. A small amount of Cr is also transported into the lungs via metalliferous vapors and dusts.
Few data are available about the metabolism of Sb and its storage in the organism. Animal studies demonstrated an accumulation of Sb in the lungs and a distribution of Sb in the liver, kidneys, thyroid gland, bones, spleen, pancreas and gastrointestinal tract when Sb was inhaled by the animals [10]. Sb may also be a factor that promotes the development of lung cancer. Employees in the metal smelt industry with this disease were found to have Sb concentrations in lung tissues that were twelve-fold higher than that observed in the healthy control group [9]. Carcinogenic effects have also been demonstrated after a long-term Sb trioxide inhalation in female rats [10].
According to the literature, the concentrations of Sr, Ba, Cd, Cu, Zn, Mn, Cr, Sb, Se, and Pb in the organs of animals are not completely known. In particular, few data have been reported for dogs. Moreover, many previous studies were performed several decades ago. This could be a critical point since the intake of the elements may have changed over time because of variations in feed. The present study was conducted to measure the concentrations of Sr, Ba, Cd, Cu, Zn, Mn, Cr, Sb, Se, and Pb in canine liver, renal cortex, and renal medulla. Age- and gender-based differences among the dogs along with associations between concentrations of the elements and occurrence of chronic kidney disease (CKD) were evaluated. Inductively coupled plasma mass spectrometry (ICP-MS) was used for this investigation. Data from the current study enhance the knowledge about the storage of these elements in dogs and provide a reference for future investigations.
Tissue samples were collected during routine post-mortem examinations of dogs at the Institute of Pathology, Department of Veterinary Medicine, Freie Universität Berlin (Germany). The dogs were euthanized by the attending veterinarian; none of the authors participated in this procedure. Euthanasia was performed exclusively for medical reasons and not tissue sampling or other research goals. Euthanasia was carried out according to the German Tierschutzgesetz (animal welfare Act) although details about the drugs administered were unspecified.
Liver and kidney samples were collected from a total of 50 dogs (26 females, 23 males, and one of unknown gender). The age of the dogs varied between 3 days and 15 years. The liver and kidney samples were stored in polyethylene bags (neoLab-Drawstring bag; neoLab, Germany) and frozen at -18℃ before further analysis. For the pathomorphological investigation, representative tissue samples were fixed in 4% neutral-buffered formaldehyde and embedded in paraffin. The tissues were cut into 4-µm sections (Sliding Microtome Microm HM 430; Thermo Scientific, Germany), mounted on glass slides (StarFrost Adhesive; Waldemar Knittel Glasbearbeitung, Germany), and stained with haematoxylin (Hemalaun solution acc. Mayer; Carl Roth, Germany) and eosin (Waldeck; Germany).
The liver and kidney samples were defrosted at room temperature and digested in a microwave-system (MLS Ethos 1600; MLS, Germany). For this wet-chemical digestion, 0.5 g of each tissue sample was mixed with 5 mL concentrated nitric acid (65%) in a quartz vessel (MLS) and subsequently transferred to a Teflon capsule (MLS) filled with 5 mL bi-distilled water and 2 mL hydrogen peroxide. The Teflon capsule was placed into a pressure vessel (MLS) to undergo wet-chemical digestion in a microwave (MLS). Acid digestion was conducted for 1 h at a maximum temperature of 200℃. The samples were allowed to cool and subsequently pipetted into graduated flasks containing bi-distilled water. The flasks were then filled with bi-distilled water to a total volume of 50 mL.
The concentrations of Sr, Ba, Cd, Cu, Zn, Mn, Cr, Sb, Se, and Pb in canine liver and kidney were measured using ICP-MS (Thermo Scientific ELEMENT 1; Thermo Scientific, Germany). The results are presented as µg/kg fresh weight of the organs. For quality assurance, bovine liver (NBS SRM biological standard 1577; Spex Industries, Germany) was digested and analyzed in the same manner as the liver and kidney samples. Results of the analysis of the reference material are presented in Table 1. For quality control (QC), a solution of digested horse liver (Roßschlächterei Plaumann, Germany) was analyzed (20 g digested in 20 parts of 1 g and diluted in 1 L water containing 6% nitric acid). The cycle for sample measurement was blank, sample, sample, blank, QC solution, sample, sample, etc. During the entire analysis, variation of the element concentrations in the QC solution was within 10%. Detection limits for the elements are presented in Table 2 and were estimated based on the mean value of the blank, including the standard deviation of the blank.
Concentrations of the elements measured in the canine liver, renal cortex, and renal medulla are presented in Table 3. In general, the variability of all parameters was high, indicating large individual differences. The highest concentration of Sr was present in the cortex of the kidney [128 (0.00 - 3,221) µg/kg] while markedly lower concentrations were measured in the renal medulla [68.4 (0.00 - 578) µg/kg] and liver [62.1 (0.00 - 525) µg/kg, p < 0.05]. The same trend was observed for Cd and Se (p < 0.05). The highest concentrations of Cu, Zn, and Mn were measured in the liver followed by the renal cortex and medulla (p < 0.05). No tissue-dependent differences were found for the levels of Ba, Cr, Sb, or Pb.
The tissue concentrations of Sr, Cd, Zn, Cr, and Pb differed somewhat depending on the gender of the dogs (Table 4). Differences were found for the liver (Sr, Cd, Zn, Cr, and Pb) as well as the renal cortex (Zn and Cr) and renal medulla (Sr and Cr). Higher concentrations were constantly observed in female dogs compared to male animals. No gender-specific differences in the amounts of Ba, Cu, Mn, Sb, and Se in canine liver and kidney were found.
Age-related differences were detected for the concentrations of Cd, Zn, Cr, Se, and Pb in canine liver (Table 5). The lowest Cd concentrations were measured in dogs 0~1 year old, and the highest levels were observed in animals 4.5~7 and 7.5~10 years old (p < 0.05). Zn and Cr concentrations were lower in the liver of dogs 1.5~4 years old compared to animals 4.5~7 and 7.5~10 years old (p < 0.05). Hepatic Se concentrations were lower in dogs 1.5~4 years old compared to canines 4.5~ 7 years old (p < 0.05), and the hepatic Pb concentrations were lower in dogs 0~4 years old compared to animals 7.5~10 years old (p < 0.05).
Most age-related differences were detected in the renal cortex of the dogs. Middle-aged and old dogs had approximately 2- to 3-fold higher Sr concentrations (median, 151 - 203 µg/kg) in the renal cortex compared to dogs 1.5~4 years old [70.2 (0.00 - 198) µg/kg; p < 0.05]. A unidirectional increase with age (p < 0.05) was observed for Cd concentrations in the renal cortex from 54.2 (2.41 - 282) µg/kg (0~1 year) up to 371 (148 - 690) µg/kg (> 10.5 years). Zn concentrations in the canine renal cortex were lower in dogs 1.5~4 years old compared to ones 4.5~7 and 7.5~10 years old (p < 0.05). Age-associated differences were also observed for the storage of Ba (4.5~7 years old versus 7.5~10 years old), Cu and Cr (1.5~4 years old versus 7.5~10 years old) as well as Se (1.5~4 years old versus 4.5~7 years old) in the renal cortex. In the renal medulla, Cu concentrations were the lowest [1,551 (907 - 3,855) µg/kg] in dogs 1.5~4 years old and 57~80% higher in animals 0~1 and 4.5~10 years old (p < 0.05). Similar results were observed for Zn concentrations in the renal medulla where the lowest concentrations [4,942 (2,805 - 14,119) µg/kg] were detected in dogs 1.5~4 years old and approximately 50% higher concentrations were measured in canines 0~1 and 4.5~ 7 years old.
Lower Ba concentrations (p < 0.05) were found in the renal medulla of dogs 7.5~10 years old compared to younger dogs (0~1 and 4.5~7 years old). Cd concentrations in the renal medulla were lower [47.1 (3.59 - 129) µg/kg] in dogs 1.5~4 years old compared to animals 4.5~7 [106 (20.6 - 217) µg/kg] or 7.5 - 10 years old [149 (4.90 - 757) µg/kg]. Additionally, very young dogs up to 1 year old had lower Cd concentrations in the renal medulla [45.0 (2.10 - 2,756) µg/kg] compared to animals 7.5~10 years old (p < 0.05). Comparable results were observed for Se concentrations in the renal medulla. Canines 1.5~4 years old had lower concentrations compared to ones 4.5~10 years old. Furthermore, very young dogs up to 1 year old had lower Se concentrations in the renal medulla compared to dogs between 4.5 and 7 years old (p < 0.05).
Sr concentrations in the renal medulla were the lowest in dogs 1.5~4 years old and the highest in animals 4.5~7 years old (p < 0.05). Differences were also observed for Pb concentrations in the canine renal medulla. Levels of this element were lower in the dogs 1.5~4 years old when compared to animals 7.5~10 years old (p < 0.05).
Six dogs in the study had chronic interstitial nephritis, nephrosis was observed in one dog, and one dog had membranoproliferative glomerulonephritis. The occurrence of CKD did not affect storage of the measured elements in canine kidneys (Table 6). In the liver, higher concentrations of Cd and Cr were measured in dogs with CKD compared to animals with normal kidney function (p < 0.05).
The present study has provided new knowledge about the storage of specific elements in the liver and kidneys of dogs. Gender- and age-associated differences were also identified. These results have practical relevance since they can serve as an indication of the exposure of the dogs to these elements either through the environment or food.
Based on the results, it can be concluded that Cu, Zn, and Mn are predominantly stored in the liver of dogs. Markedly lower concentrations were measured in the renal cortex and the lowest concentrations were detected in the renal medulla. These findings concur with results from another study in dogs [18]. Moreover, other investigations also found that the highest Zn accumulation occurs in canine liver [35] while the highest Mn concentrations in humans are found in the liver [28] compared to other tissues.
Since the liver is a storage site for Cu, total Cu levels in an organism are related to hepatic Cu concentrations [25]. Thus, Cu levels measured in the liver also reflect the amount of dietary Cu consumed. Unfortunately, the present study design did not allow a retrospective evaluation of the dietary regimes of the dogs. However, large differences of hepatic Cu concentrations among the individual canines indicate that daily Cu intake also varied greatly. In general, the measured Cu concentrations in the liver of the dogs [44,727 (6,629 - 242,972) µg/kg] were comparable to those recently reported (42,100 µg/kg) [18] but below those for dogs published in 1981 (200 - 400 µg/g dry matter [DM]) [16]. In contrast, other authors [14] demonstrated that hepatic Cu accumulation in dogs has increased over time when data from 1980~1997 and 1998~2010 were compared, and suggested that this increase could be a result of a higher dietary Cu intake nowadays. When compared to results from the present investigation, measured hepatic Cu concentrations were generally higher in the past [14]. Additionally, significantly higher Cu concentrations were detected in the liver of dogs with chronic hepatitis (614 µg/g DM) compared to control dogs (299 µg/g DM). However, a broad range of hepatic Cu concentrations has been reported [14], which might account for the differences between results from the literature and our data.
No age-dependent differences for the storage of Cu in the canine liver were detected. We did, however, observe age-dependent differences for renal Cu concentrations that were the lowest for dogs 1.5~4 years old compared to animals 7.5~10 years old (renal cortex) as well as dogs 4.5~10 years old and up to 1 year old (renal medulla). These results contrast with those from a recent study in which age-dependent differences for Cu storage in the kidneys of dogs were not found [18]. However, the authors observed decreasing hepatic Cu concentrations for male dogs up to age 6~9 years and a subsequent increase in older male dogs (> 10 years). This effect was less apparent for female dogs [18]. Our results also contrast with observations in humans showing that Cu concentrations in the liver, kidney, and ribs decrease with age [1]. Gender-specific differences were also reported in which women had higher Cu concentrations in the liver compared to men. This effect was not observed in the present investigation or in the study by López-Alonso et al. [18].
Hepatic Zn concentrations measured in the present study [21,837 (10,150 - 54,707) µg/kg] were lower than those previously reported [18]. We also found gender-specific differences in Zn accumulation with significantly (liver and renal cortex) or numerically (renal medulla) higher concentrations in female dogs compared to male animals. This observation concurs with previous findings in humans [1,11] and dogs [18]. The reasons for this sexual dimorphism remain unclear.
Few data have been reported for the age-dependent storage of Zn in canines. A previous study showed that Zn storage in canine liver is age-dependent, but not in the kidneys of dogs [18]. Zn concentrations decreased when comparing young male dogs (< 1 year old) to middle-aged male animals (6~9 years old) and subsequently increased in older male canines (> 10 years old). This pattern was less clear for female dogs [18]. In the present study, age-dependent differences were detected for Zn concentrations in canine liver and renal cortex. Younger dogs (1.5~4 years old) had significantly (compared to dogs 4.5~10 years old) or numerically (compared to animals >10 years old) lower Zn concentrations. Zn concentrations in the renal medulla were significantly lower in dogs 1.5~4 years old compared to ones 4.5~7 years old and canines up to 1 year old.
Renal and hepatic Mn accumulation did not appear to be age- or gender-dependent in the dogs. This is in contrast to findings from another recent study in which lower Mn concentrations were detected in the liver of male dogs compared to female animals [18]. Moreover, hepatic Mn concentrations were found to decrease in male dogs up to an age of 6~9 years and subsequently increase in older male dogs; these effects were not observed in female canines [18]. In humans, Mn levels in the kidneys and ribs decreased with increasing age, and women showed higher concentrations in both tissues compared to men [2]. However, no age-associated differences in Mn storage were found in human subjects in a different study [30]. Overall, Mn accumulation can be assumed to vary greatly among individual organisms.
In the present investigation, the highest Sr concentrations were found in the renal cortex of the dogs followed by the renal medulla and liver. We also identified gender-specific differences in which female dogs had significantly or numerically higher concentrations of Sr in the liver and kidneys. Moreover, age-dependent differences were detected with lower Sr concentrations in dogs 1.5~4 years old compared to ones 4.5~ 7 (renal medulla) or > 4.5 (renal cortex) years old. These findings are in contrast to those from a human study, which demonstrated that children up to 9 years old have higher Sr concentrations in the liver and kidneys compared to older individuals although humans older than 50 years accumulate more Sr in the kidneys [31]. Aside from species-dependent differences, variations in basic diet or living conditions may play a role for the differences detected between dogs and humans since Sr intake is mediated via air and food.
Se concentrations were higher in the renal cortex [627 (140 - 3,102) µg/kg] compared to the liver [426 (98.4 - 1,909) µg/kg] of the dogs while lowest Se concentrations were measured in the renal medulla [196 (72.2 - 2,482) µg/kg]. In general, the hepatic Se concentrations were similar to those reported by Todd et al. [36] (1.37 ± 0.13 µg Se/g DM) but lower compared to those reported by López-Alonso et al. [18] [0.761 (undetected-2.55) mg/kg). These differences might be explained by variations in Se intake since it has been demonstrated that dogs accumulate Se in the liver when higher amounts of Se are provided with food [36]. In addition, the renal Se concentrations of the dogs measured in the present study were lower than those described by López-Alonso et al. [18] [1.45 (0.657 - 2.38) mg/kg]. It can be hypothesized that these differences may also result from variations in dietary Se since Todd et al. [36] observed an increased hepatic Se accumulation associated with increased Se intake in dogs along with elevated urinary Se excretion. Renal storage of Se dependent on the dietary Se supply could explain variations in renal Se concentrations of dogs.
Only differences among individual groups were detected when evaluating age-related differences of Se storage in the liver and renal cortex of the dogs. In the renal medulla, generally lower concentrations were measured in younger dogs up to 4 years old compared to middle-aged and older dogs up to 10 years old. These results are in contrast to data from López-Alonso et al. [18] who did not observe age-associated differences for renal and hepatic Se accumulation in dogs. The authors [18] also failed to detect gender-specific differences similar to our findings.
The concentrations of Ba, Cr, Sb, and Pb in the liver and kidneys of the dogs did not differ. However, we noted some gender- or age-related variations. In particular, Cr concentrations were always higher in female dogs compared to male canines. Other groups failed to find gender-related differences in the hepatic and renal storage of Cr in dogs [18]. In humans, men were found to accumulate more Cr in the liver and kidney compared to women [33]. Additionally, younger dogs were found to have lower Cr concentrations in the liver and renal cortex compared to older dogs in the present study, but others did not observe any age-dependent differences for Cr accumulation in the liver and kidneys of dogs [18]. In other investigations, higher Cr concentrations were observed in the organs and tissues of newborn humans and young animals compared to older ones [29,33]. The reasons for these contrary data from the literature and the present study are unclear. Since Cr metabolism has been insufficiently assessed and Cr is ubiquitously present in food, air, water, and soil, no adequate conclusion can be made.
Similar to Cr, higher concentrations of Cd were detected in the liver of female dogs compared to male animals while lower concentrations were measured in the liver and kidney of younger dogs compared to older canines. In two previous studies [18,19], lower hepatic Cd concentrations (58.0 µg/kg and 56.3 µg/kg) were found in dogs while the renal Cd concentrations (175.5 µg/kg and 166 µg/kg) were between the levels measured in the renal cortex and medulla of the dogs in the present study.
The finding of higher Cd concentrations in female dogs compared to male canines is supported by previously reported data for dogs [19], horses [3], and humans [17]. Moreover, studies in horses [27] and humans [2] demonstrated that Cd concentrations in organs first increase with increasing age but subsequently decrease with more advanced age. In the present study, significant differences were only detected when younger dogs were compared with older dogs. Older animals had higher Cd concentrations in the liver and kidney. However, a numerical decrease of Cd levels was observed in the liver and renal medulla of very old dogs (> 10.5 years old). Studies by López-Alonso et al. [18,19] demonstrated that the renal Cd concentrations significantly increase with increasing age in dogs.
Similar to Cr and Cd, higher Pb concentrations were found in the liver of female dogs compared to male dogs, and lower Pb concentrations were detected in the liver and renal medulla of younger dogs compared to older dogs. These findings are in contrast to data from López-Alonso et al. [18,19] demonstrating that renal storage of Pb in dogs is unaffected by the age or gender. The authors [19] also failed to detect gender- or age-related differences for hepatic Pb accumulation. In another study [18], high hepatic Pb concentrations in very young dogs up to 1 year, lower Pb concentrations in middle-aged dogs, and a subsequent increase in hepatic Pb concentrations in older animals were found. López-Alonso et al. [19] observed higher hepatic Pb concentrations when the dogs received commercial diets compared to homemade diets or a mixture of commercial and homemade diets, and the measured hepatic and renal Pb concentrations were generally lower than the levels found in the present study. Variations in Pb uptake of the dogs may therefore be one explanation for the observed differences between the present results and the findings of López-Alonso et al. [18,19].
Ba concentrations in the tissues of the dogs did not differ depending on gender. Only minor age-associated effects were observed with lower concentrations in the kidneys of dogs 7.5~ 10 years old compared to dogs 4.5~7 years old (renal cortex) or dogs 0~1 and 4.5~7 years old (renal medulla). These data are similar to findings in humans in which higher Ba concentrations were observed in the liver and kidney of children compared to older persons [31].
Sb concentrations in the tissues of the dogs did not vary according to the age or gender of the animals. Up to now, only few data have been available and the present results are therefore of high importance for increasing the knowledge about Sb storage in canines. In humans [33], Sb concentrations in the liver (20 µg/kg) and kidneys (10~20 µg/kg) were found to be lower compared to those observed in the present study. The underlying causes of these species-dependent differences are unclear.
The occurrence of CKD only marginally affected levels of the measured elements in the tissues of the dogs. Higher Cd and Cr concentrations were found in the liver of animals with CKD. In humans, lower concentrations of Mn were observed in the liver, kidneys, cardiac muscle, cerebrum, ribs, testes, lung, and whole blood of individuals with CKD [32]. These observations conflicted with data from the current study.
In conclusion, results from the present investigation enhance the knowledge about Sr, Ba, Cd, Cu, Zn, Mn, Cr, Sb, Se, and Pb storage in the liver and kidneys of dogs. The measured concentrations along with observed gender- and age-related variations differed somewhat compared to data from humans or other animals. Aside from species-dependent characteristics, exogenous factors, especially living conditions and diet, may help explain the observed variations.
Figures and Tables
Table 1
Results (expressed in mg/kg) of the analyzed Certified Reference Material NBS SRM biological standard 1577 (bovine liver)
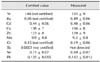
Table 3
Concentrations (µg/kg) of Sr, Ba, Cd, Cu, Zn, Mn, Cr, Sb, Se, and Pb in the liver and kidneys of dogs (n = 50)
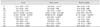
Table 4
Concentrations (µg/kg) of Sr, Ba, Cd, Cu, Zn, Mn, Cr, Sb, Se, and Pb in the liver and kidneys of dogs that varied according to gender
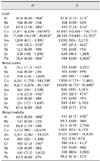
Table 5
Concentrations (µg/kg) of Sr, Ba, Cd, Cu, Zn, Mn, Cr, Sb, Se, and Pb in the liver and kidneys of dogs that varied depending on age1
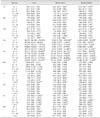
1n = 10 (0~1 year), n = 10 (1.5~4 years), n = 10 (4.5~7 years), n = 13 (7.5~10 years), and n = 7 (> 10 years). Group comparisons were made separately for each element and each tissue. Significant differences (p < 0.05) within a column are marked with different superscript letters. Median (minimum - maximum).
References
1. Anke M, Schneider HJ. Zinc, cadmium and copper metabolism in man. Arch Exp Veterinarmed. 1971; 25:805–809.
2. Anke M, Schneider HJ, Grün M, Groppel B, Hennig A. The diagnosis of manganese, zinc and copper deficiency and cadmium exposure. Zentralblatt für Pharmazie, Pharmakotherapie und Laboratoriumsdiagnostik. 1978; 117:688–707.
3. Baldini M, Stacchini P, Cubadda F, Miniero R, Parodi P, Facelli P. Cadmium in organs and tissues of horses slaughtered in Italy. Food Addit Contam. 2000; 17:679–687.


4. Drinker KR, Thompson PK, Marsh M. An investigation of the effect of long-continued ingestion of zinc, in the form of zinc oxide, by cats and dogs, together with observations upon the excretion and the storage of zinc. Am J Physiol. 1927; 80:31–64.


5. EFSA Scientific Panel on Contaminants in the Food Chain (CONTAM). Opinion of the scientific panel on contaminants in the food chain on a request form the commission related to lead as undesirable substance in animal feed. EFSA J. 2004; 71:1–20.
6. EFSA Panel on Contaminants in the Food Chain (CONTAM). Scientific opinion on lead in food. EFSA J. 2010; 8:1570.
7. Ellsasser JC, Farnham JE, Marshall JH. Comparative kinetics and autoradiography of 45Ca and 133Ba in ten-year-old beagle dogs: the diffuse component distribution throughout the skeleton. J Bone Joint Surg Am. 1969; 51:1397–1412.


8. Froidevaux P, Bochud F, Haldimann M. Retention half times in the skeleton of plutonium and 90Sr from above-ground nuclear tests: a retrospective study of the Swiss population. Chemosphere. 2010; 80:519–524.


9. Gerhardsson L, Brune D, Nordberg GF, Wester PO. Antimony in lung, liver and kidney tissue from deceased smelter workers. Scand J Work Environ Health. 1982; 8:201–208.


10. Greim H. The MAK-Collection for Occupational Health and Safety. Part I: MAK Value Documentations. Weinheim: WILEY-VCH;2007. Vol. 23.
11. Groppel B, Hennig A. Zinc deficiency in ruminants. Arch Exp Veterinarmed. 1971; 25:817–821.
12. Grün M, Kronemann H. Relevance of the trace element zinc in human nutrition. Zentralblatt für Pharmazie. 1978; 117:711–719.
13. Hecht H. Carry-over-processes when producing food of animal origin. Fleischwirtschaft. 1984; 64:12041206–1209.
14. Johnston AN, Center SA, McDonough SP, Wakshlag JJ, Warner KL. Hepatic copper concentrations in Labrador Retrievers with and without chronic hepatitis: 72 cases (1980-2010). J Am Vet Med Assoc. 2013; 242:372–380.


15. Kane E, Morris G, Rogers QR, Ihrke PJ, Cupps PT. Zinc deficiency in the cat. J Nutr. 1981; 111:488–495.


16. Keen CL, Lönnerdal B, Fisher GL. Age-related variations in hepatic iron, copper, zinc and selenium concentrations in beagles. Am J Vet Res. 1981; 42:1884–1887.
17. Kjellström T. Exposure and accumulation of cadmium in populations from Japan, the United States, and Sweden. Environ Health Perspect. 1979; 28:169–197.


18. Löpez-Alonso M, Miranda M, García-Partida P, Mendez A, Castillo C, Benedito JL. Toxic and trace metal concentrations in liver and kidney of dogs: influence of diet, sex, age, and pathological lesions. Biol Trace Elem Res. 2007; 116:185–202.
19. Löpez-Alonso M, Miranda M, García-Partida P, Cantero F, Hernández J, Benedito JL. Use of dogs as indicators of metal exposure in rural and urban habitats in NW Spain. Sci Total Environ. 2007; 372:668–675.


20. Mahoney JP, Small WJ. Studies on manganese: III. The biological half-life of radiomanganese in man and factors which affect this half-life. J Clin Invest. 1968; 47:643–653.
21. Mehdi Y, Hornick JL, Istasse L, Dufrasne I. Selenium in the environment, metabolism and involvement in body functions. Molecules. 2013; 18:3292–3311.


22. Mena I. Metals in The Environment. In : Waldron HA, editor. Manganese. London: Academic Press;1980. p. 199–200.
23. Mertz W. Trace Elements in Human and Animal Nutrition. Orlando: Academic Press;1988.
24. Petrides PE, Löffler G. Biochemistry and pathobiochemistry. Berlin: Springer;1998. p. 624–626.
25. Plumlee KH, Johnson B, Gardner IA. Heavy metal concentrations in injured racehorses. Vet Hum Toxicol. 1996; 38:204–206.
26. Rhian M, Moxon AL. Chronic selenium poisoning in dogs and its prevention by arsenic. J Pharmacol Exp Ther. 1943; 78:249–264.
27. Salmi A, Hirn J. Cadmium content of muscle, liver and kidney from Finnish horse and reindeer. Fleischwirtschaft. 1981; 61:1199–1201.
28. Schneider HJ, Anke M. Calcium, phosphorus and manganese content of various organs in man. Arch Exp Veterinarmed. 1971; 25:787–792.
29. Schroeder HA, Balassa JJ, Tipton IH. Abnormal trace metals in man: chromium. J Chronic Dis. 1962; 15:941–964.
30. Schroeder HA, Balassa JJ, Tipton IH. Essential trace metals in man: manganese. A study in homeostasis. J Chronic Dis. 1966; 19:545–571.
31. Schroeder HA, Tipton IH, Nason AP. Trace metals in man: strontium and barium. J Chronic Dis. 1972; 25:491–517.


32. Stein G, Anke M, Fünfstück R, Schneider HJ. The effect of chronic renal insufficiency with and without dialysis therapy on the manganese content of various human organs. Z Gesamte Inn Med. 1979; 34:648–651.
33. Sumino K, Hayakawa K, Shibata T, Kitamura S. Heavy metals in normal Japanese tissues. Amounts of 15 heavy metals in 30 subjects. Arch Environ Health. 1975; 30:487–494.
34. Taylor DM, Bligh PH, Duggan MH. The absorption of calcium, strontium, barium and radium from the gastrointestinal tract of the rat. Biochem J. 1962; 83:25–29.


35. Thind GS, Fischer GM. Cadmium and zinc distribution in cardiovascular and other tissues of normal and cadmiumtreated dogs. Exp Mol Pathol. 1975; 22:326–334.


36. Todd SE, Thomas DG, Bosch G, Hendriks WH. Selenium status in adult cats and dogs fed high levels of dietary inorganic and organic selenium. J Anim Sci. 2012; 90:2549–2555.


37. Van Vleet JF. Experimentally induced vitamin E-selenium deficiency in the growing dog. J Am Vet Med Assoc. 1975; 166:769–774.
38. Wedekind KJ, Kirk C, Yu S, Nachreiner R. Defining the safe lower and upper limits for selenium in adult dogs. Fed Proc. 2002; 16:A992–A993.