Abstract
To assess relationships between xanthine oxidase (XOD) and nephropathogenic infectious bronchitis virus (NIBV) infection, 240 growing layers (35 days old) were randomly divided into two groups (infected and control) of 120 chickens each. Each chicken in the control and infected group was intranasally inoculated with 0.2 mL sterile physiological saline and virus, respectively, after which serum antioxidant parameters and renal XOD mRNA expression in growing layers were evaluated at 8, 15 and 22 days post-inoculation (dpi). The results showed that serum glutathione peroxidase and superoxide dismutase activities in the infected group were significantly lower than in the control group at 8 and 15 dpi (p < 0.01), while serum malondialdehyde concentrations were significantly higher (p < 0.01). The serum uric acid was significantly higher than that of the control group at 15 dpi (p < 0.01). In addition, the kidney mRNA transcript level and serum activity of XOD in the infected group was significantly higher than that of the control group at 8, 15 and 22 dpi (p < 0.05). The results indicated that NIBV infection could cause the increases of renal XOD gene transcription and serum XOD activity, leading to hyperuricemia and reduction of antioxidants in the body.
Infectious bronchitis virus (IBV) can cause serious and highly contagious viral disease in chickens and has many serotypes or variants [34]. Nephropathogenic infectious bronchitis virus (NIBV) is one of the most prevalent viruses, exhibiting strong renal tropism and the potential to cause significant mortality to the poultry industry [1235]. Chickens of all ages may be infected, and infected chickens are characterized by coughing, depression, nasal discharge, sneezing, polyuria, and death [425]. Clinicopathological studies reported that infection with NIBV can cause the kidneys of chickens to become swollen and pale, with deposition of monosodium urate crystals in tubules and ureters, resulting in visceral gout [1430]. Exacerbation of visceral gout may be associated with adverse metabolic, nutritional, or infectious conditions, and toxicity. Infections have become the most important cause of gout, and nephropathogenic strain of infectious bronchitis virus has become one of the most prevalent pathogens associated with the outbreak of visceral gout [11].
Gout is a metabolic disorder, and hyperuricemia has been shown to be an independent risk factor for gout [10]. In poultry, avian gout is usually divided into visceral gout and articular gout. Former researchers have defined visceral gout as a disease induced by the accumulation of uric acid crystals in different internal organs that stimulate phagocytosis and initiate the inflammatory cascade [30]. Edwards [9] reported that elevated serum urate levels were closely associated with deposited urate crystals in intrarenal tissues. Uric acid, which is the ultimate decomposition product of endogenous or dietary purines in chickens, is generated by xanthine oxidase (XOD). Previous studies reported that increases in XOD activity were correlated with elevated serum urate levels [13].
XOD is a molybdenum iron-sulfur flavin hydroxylase that is widely distributed in most animal species [828]. Beedkar et al. [5] demonstrated that XOD was a vital mediator in the pathogenesis of human gout. In addition to the generation of uric acid, XOD can catalyze the substrate and concomitant generation of oxygen radicals, which can then induce tissue damage. There is mounting evidence that increased XOD activity is correlated with the development of many diseases due to the concomitantly increased production of uric acid and free radicals [616]. However, to prevent tissue damage caused by oxidative stress, the major antioxidant enzymes such as superoxide dismutases (SOD) and glutathione peroxidase (GSH-PX) played an important role in maintaining the balance between antioxidants and oxidation in vivo [1727]. Tas et al. [31] reported that increased activities of SOD and GSH-PX could reduce the oxidative damage induced by reactive oxygen species (ROS) in rats.
However, few studies have investigated the relationships among XOD, antioxidants and gout induced by NIBV. In the present study, chickens were infected with NIBV and changes in the renal XOD mRNA transcript level, serum XOD, serum uric acid and antioxidant levels were evaluated to clarify the dynamic characteristics of XOD and antioxidants in chickens after infection with NIBV.
A total of 280 1-day-old Hyline brown female chicks were obtained from a local hatchery (Nanchang, Jiangxi, China) and provided with a commercial chick starter diet prepared according to the National Research Council (USA) recommendations (NRC 1994). At 35d of age, 240 apparently healthy Hyline brown hens were randomly allocated to infected and control groups and individually numbered. Each group contained three replicates, and there were 40 birds in each replicate. During the experiment, chicks were reared separately in two animal houses of the Animal Science and Technology School of Jiangxi Agricultural University and received feed and water ad libitum.
The nephropathogenic infectious bronchitis virus was preserved by the clinical veterinary science laboratory of Jiangxi Agricultural University. The virus was propagated in 10-day-old specific pathogen-free (SPF) embryonating chicken eggs. Briefly, serial 10-fold dilutions (10-1 to 10-10) of the virus were prepared in sterile physiological saline and used to infect SPF embryonating chicken eggs. The 10-5 embryo median lethal dose 50 was calculated according to the formula of Reed and Muench [26]. Each chicken in the infected group was intranasally inoculated with 0.2 mL of 10-5 median embryo infectious doses of nephropathogenic infectious bronchitis virus at 35 days of age. Chickens in the control group were intranasally inoculated with 0.2 mL of sterile physiological saline as virus-free controls. Chickens were examined daily for signs of infection after inoculation. Clinical signs were observed at 7 days post-inoculation (dpi) in most infected chickens. We then collected blood and organ samples at 8, 15 and 22 dpi (12 blood samples were collected from each group every time). Sera were separated by centrifugation for 5 min at 2,500 × g, then stored at -20℃ for analysis. Organ samples (kidney) were individually collected into the freezing tubes and snap frozen in liquid nitrogen. Subsequently, snap-frozen organs were stored at -80℃ immediately until RNA extraction. All experiments were conducted after institutional approval of the animal Care and Use Committee of Jiangxi Agricultural University.
The serum activities or concentrations of XOD, SOD, GSH-PX, uric acid (UA) and malondialdehyde (MDA) were measured using commercially available kits (Nanjing Jiancheng Bioengineering Research Institute, China) according to the manufacturer's instructions.
Total RNA was extracted from 12 individual chicken organs (12 kidneys) per group by homogenization in TranZol reagent (TransGen Biotech, China) using a homogenizer under low temperature according to the manufacturer's protocols. RNA was diluted in nuclease-free water, then quantified based on UV spectrophotometry at 260 nm. The integrity of the extracted RNA was confirmed by 0.8% agarose gel electrophoresis.
Before reverse transcription reaction, all RNA samples were individually treated by Genomic DNA elimination reaction (Perfect Real Time; Takara Bio, China) according to the manufacturer's instructions to remove genomic DNA. Briefly, 10 µL of total reaction mixture (1.0 µL gDNA Eraser, 2.0 µL 5× gDNA Eraser Buffer, 0.1 µg total RNA, and RNase free dH2O) was incubated at 42℃ for 2 min, then stored at 4℃. cDNA was synthesized by reverse transcription reaction in a reaction volume of 20 µL containing: reaction solution from Genomic DNA elimination reaction, 10 µL; RT Primer Mix, 4.0 µL; 5 × PrimeScript Buffer 2, 4.0 µL; PrimeScript RT Enzyme Mix 1, 1.0 µL; RNase Free dH2O, 1.0 µL. Reactions were carried out in a thermal cycler (LifePro Thermal Cycler; Hangzhou Bioer Technology, China) by subjecting samples to 37℃ for 15 min followed by 85℃ for 15 sec, then stored at -80℃.
Real-time PCR was conducted using 300 ng of cDNA and a Premix EX Taq Probe qPCR Kit (no. DRR390A; Takara Bio) in 384 well plates in a ABI Prism 7900 Sequence Detection System (Applied Biosystems, USA) according to the manufacturer's instructions. Relative quantification of each gene was normalized to glyceraldehyde-3-phosphate dehydrogenase (GAPDH). The primer and probe sequences are shown in Table 1. The total 10 µL reaction mixture included: 2 × Premix Ex Taq (ProbeqPCR; Takara Bio), 5 µL; PCR forward primer (10 µM), 0.2 µL; PCR reverse primer (10 µM), 0.2 µL; probe (10 µM), 0.2 µL; 50 × ROX Reference Dye (Thermo Fisher Scientific, USA), 0.2 µL; total cDNA, 3 µL; ddH2O, 2.2 µL. PCR was conducted using an Applied Biosystems ABI Prism 7900 Sequence Detection System and consisted of the following conditions: 5 min at 95℃ followed by 40 cycles of 15 sec at 95℃ and 1 min at 60℃. Amplification curves were analyzed using the ABI Prism 7900 Sequence Detection Software to obtain Ct values for each PCR system.
All data were analyzed by SPSS (ver. 17.0; SPSS, USA). Data were determined to be normally distributed by the Shapiro-Wilk test. Differences between the control and the affected group were identified by one-way analysis of variance (ANOVA). The results were expressed as the means ± the standard deviation (SD). Data were considered statistically significant at p < 0.05.
No clinical signs were observed in chickens in the control groups throughout the experimental period. However, various clinical signs were observed in chickens of the infected group at 6 dpi, such as depression, crouching and coughing. At 7 dpi, most of the chickens showed rales, coughing, head shaking and defecation of white excrement. At 8 dpi, severe diarrhea and death were observed in infected chickens. Overall, 28 of the infected chickens died after 8 dpi. Bilateral renal enlargement and deposits of pale urate were observed in the tubules and ureter upon postmortem examination of the dead pullets.
Figs. 1,2,3 show the serum XOD, GSH-PX and SOD activity in the infected group and control group at 8, 15 and 22 dpi, individually. At 8 and 15 dpi, the serum activity of XOD in the infected group had increased significantly when compared with the control group (p < 0.01), and a significant increase was observed at 22 dpi (p < 0.05; Fig. 1). GSH-PX and SOD activities were statistically lower than in the control group at 8 dpi and 15 dpi (p < 0.01; GSH-PX, Fig. 2; SOD, Fig. 3). At 22 dpi, SOD and GSH-PX did not differ significantly, but a steadily decreasing trend between infected and control groups was observed.
The serum concentrations of UA and MDA are shown in Figs. 4,5. MDA was found to be significantly higher in the infected group than in the control group at 8 dpi (p < 0.01; Fig. 4), while no significant difference was observed in UA (p > 0.05; Fig. 5). At 15 dpi, the serum concentration of UA and MDA in the infected group was statistically higher than that of the control group (p < 0.01). At 22 dpi, no significant difference was observed in UA and MDA between the infected and control group.
The levels of renal XOD mRNA transcription are shown in Fig. 6. At 8, 15 and 22 dpi, the transcript levels of XOD in the infected group had increased significantly relative to the control group (p < 0.01).
XOD can oxidize a variety of purines, pyrimidines, pteridines, and other heterocyclic nitrogenous compounds while concomitantly generating UA, superoxide anion (O2 -) and hydrogen peroxide (H2O2). It is well known that appropriate concentrations of UA and reactive oxygen species (ROS) are necessary for maintenance of homeostasis. Previous studies have shown that hyperactivity of XOD occasionally occurs in the body, and that by-products of both UA and ROS play important roles in various pathophysiological conditions [2]. Peralta et al. [22] demonstrated that XOD activity induced by hepatic ischemia-reperfusion was associated with liver and lung damage. Among human diseases, gout or hyperuricemia was also associated with the activity of XOD, and this enzyme has become a target of drugs used for therapy [20]. Therefore, its activity plays an important role in the development of several diseases. Former studies have shown that infection with NIBV could induce elevation of serum UA in chickens [1]. However, few studies have been conducted to explain the relationships between XOD and hyperuricemia induced by NIBV in the poultry industry to date.
In the present study, chickens in challenged groups sustained the highest levels of XOD mRNA transcript in kidneys at 8, 15 and 22 dpi (p < 0.01). These results were correlated with the serum activity of XOD between infected and control groups. Previous studies demonstrated that XOD activity or gene transcription could be reinforced by hypoxia and pro-inflammatory cytokines in vitro. Poss et al. [24] showed that hypoxia exposure alone increased XOD activity, but that mRNA transcript levels remained normal in cultured bovine aortic endothelial cells. These results may have occurred because the bovine XOD gene lacks a hypoxia response element for hypoxia-inducible factor-1 [24]. However, Pfeffer et al. [23] demonstrated that cytokines including TNF, IFN-γ, IL-6, and IL-1 could induce increases in both XOD activity and mRNA transcript levels in bovine renal epithelial cells. Asasi et al. [4] demonstrated that the serum concentration of IFN-γ increased significantly after IBV infection. Furthermore, Okino et al. [21] showed that the highest relative expression of IL-6, IL-1, and IFN-γ was correlated with the degree of IBV infection. A previous study also revealed that NIBV infection could induce renal inflammatory responses [33]. Therefore, it is likely that the increase of XOD renal transcription and its serum activity was due to NIBV infection induced by the increase of pro-inflammatory cytokines. However, further study is needed to elucidate the specific mechanism responsible for the increase of XOD renal transcription and its serum activity induced by NBIV infection.
Uric acid is as an end product of endogenous or dietary purines generated by XOD in chickens. In the present study, a significant increase in the serum concentration of UA was observed in the infected group relative to the control group at 15 dpi (p < 0.01). These findings were positively correlated with the increase of serum XOD activity at 15 dpi. Ejaz et al. [10] revealed that long-standing hyperuricemia could result in UA crystal deposition in intrarenal tissues and tubulointerstitial injury. A previous study showed that NIBV triggers UA salt precipitation by invading the tubule cell to initiate kidney tissue damage, and that the accumulation of UA salt could block the ureter [33]. Therefore, the increase in UA generation induced by the hyperactivity of XOD and damage of the kidney caused by NIBV might be the major reason for chicken hyperuricemia.
In addition to the generation of UA, reactive oxygen species (ROS) were also generated by XOD. Chambers et al. [7] reported that XOD was a major source of ROS in reperfused myocardium. In disease promotion and prevention, ROS act as a double-edged sword. On one hand, ROS prevent initiation of disease and play a key role in the immune system and apoptosis to eliminate damaged cells [29]. Vorbach et al. [32] reported that ROS could also function as a microbicide by generating an entire cascade of microbicidal reactions and products. Conversely, the aberrant production of ROS could randomly attack lipids, proteins, nucleic acids, and carbohydrates, causing cell and tissue damage [19]. Former studies have 19reported that overproduction of free radicals could induce excessive degeneration of the polyunsaturated lipids, leading to elevated MDA levels [317]. Previous studies have also shown that MDA was a significant indicator to check lipid peroxidation and cellular oxidative damage [15], and its concentration was positively correlated with the degree of free radical-induced damage. In the present study, serum MDA values were statistically higher in the infected group than the control group at 8 and 15 dpi (p < 0.01). These results indicated that the chicken suffered oxidative damage induced by the aberrant production of ROS in the infected group.
SOD and GSH-PX are free radical scavengers that play a key role in maintenance of the balance between antioxidants and oxidation. SOD is a major defense enzyme for free radical metabolism that clears superoxide anion (O2 -), thus preventing cell damage caused by toxic oxygen radicals. GSH-PX eliminates intracellular H2O2 and lipid radicals. The activities of both SOD and GSH-PX reflect the capability of elimination of free radicals in the body. In our study, the activities of serum GSH-PX and SOD decreased significantly in the infected group relative to the control group at 8 and 15 dpi (p < 0.01), while no significant difference but a steadily decreasing trend was observed at 22 dpi. The SOD and GSH-PX levels indicated that chicken's infected with NIBV would induce an increase in ROS, leading to decreased antioxidants in the body. Nath et al. [18] reported that the kidney was vulnerable to attack by ROS. Therefore, the aberrant generation of ROS might be an another pathogenesis of kidney damage in chickens caused by NIBV infection.
In conclusion, our results demonstrated that NIBV infection could elevate XOD gene transcription and its serum activity. The elevation of XOD activity could concomitantly lead to increased UA and ROS contents. On one hand, the increase of serum UA could lead to hyperuricemia, which is the main risk factor for UA crystal deposition. On the other hand, the increase of ROS could not only induce decreased antioxidants, but also cause tissue oxidative damage.
Figures and Tables
Fig. 1
Serum xanthine oxidase (XOD) activities in chickens in the control and infected groups. N = 12 per group at each time. Significant differences are indicated by *p < 0.05 and **p < 0.01 in comparison with control group.
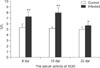
Fig. 2
Serum glutathione peroxidase (GSH-PX) activities in chickens in the control and infected groups. N = 12 per group at each time. Significant differences are indicated by **p < 0.01 in comparison with control group.
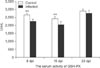
Fig. 3
Serum superoxide dismutases (SOD) activities in chickens in the control and infected groups. N = 12 per group at each time. Significant differences are indicated by **p < 0.01 in comparison with control group.
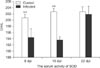
Fig. 4
Serum uric acid (UA) contents in chickens in the control and infected groups. N = 12 per group at each time. Significant differences are indicated by **p < 0.01 in comparison with control group.
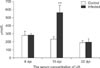
Fig. 5
Serum malondialdehyde (MDA) contents in chickens in the control and infected groups. N = 12 per group at each time. Significant differences are indicated by **p < 0.01 in comparison with control group.
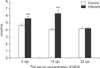
Acknowledgments
This project was supported by a National Natural Science Foundation of China grant awarded to Xiaoquan Guo (nos. 31260627 and 30860212), a Jiangxi Young Scientists Target Training Program grant to Xiaoquan Guo (no. 20122BCB23 022) and the Technology R&D Program of Jiangxi Province awarded to Xiaoquan Guo (no. 2010BNB00501).
References
1. Afanador G, Roberts JR. Effect of nephropathogenic infectious bronchitis viruses on renal function in young male broiler chickens. Br Poult Sci. 1994; 35:445–456.


2. Agarwal A, Banerjee A, Banerjee UC. Xanthine oxidoreductase: a journey from purine metabolism to cardiovascular excitation-contraction coupling. Crit Rev Biotechnol. 2011; 31:264–280.


3. Al-Hakeim HK, Auda FM, Ali BM. Lack of correlation between non-labile iron parameters, total carbonyl and malondialdehyde in major thalassemia. J Clin Biochem Nutr. 2014; 55:203–206.


4. Asasi K, Mohammadi A, Boroomand Z, Hosseinian SA, Nazifi S. Changes of several acute phase factors in broiler chickens in response to infectious bronchitis virus infection. Poult Sci. 2013; 92:1989–1996.


5. Beedkar SD, Khobragade CN, Chobe SS, Dawane BS, Yemul OS. Novel thiazolo-pyrazolyl derivatives as xanthine oxidase inhibitors and free radical scavengers. Int J Biol Macromol. 2012; 50:947–956.


6. Celik VK, Sari I, Engin A, Gürsel Y, Aydin H, Bakir S. Determination of serum adenosine deaminase and xanthine oxidase levels in patients with crimean-congo hemorrhagic fever. Clinics (Sao Paulo). 2010; 65:697–702.


7. Chambers DE, Parks DA, Patterson G, Roy R, McCord JM, Yoshida S, Parmley LF, Downey JM. Xanthine oxidase as a source of free radical damage in myocardial ischemia. J Mol Cell Cardiol. 1985; 17:145–152.


8. Dupont GP, Huecksteadt TP, Marshall BC, Ryan US, Michael JR, Hoidal JR. Regulation of xanthine dehydrogenase and xanthine oxidase activity and gene expression in cultured rat pulmonary endothelial cells. J Clin Invest. 1992; 89:197–202.


9. Edwards NL. The role of hyperuricemia and gout in kidney and cardiovascular disease. Cleve Clin J Med. 2008; 75:Suppl 5. S13–S16.


10. Ejaz S, Kim BS, Lim CW. Gout Induced by intoxication of sodium bicarbonate in Korean native broilers. Drug Chem Toxicol. 2005; 28:245–261.


11. Gaba A, Dave H, Pal JK, Prajapati KS. Isolation, identification and molecular characterization of IBV variant from out break of visceral gout in commercial broilers. Vet World. 2010; 3:375–377.
12. Ji J, Xie J, Chen F, Shu D, Zuo K, Xue C, Qin J, Li H, Bi Y, Ma J, Xie Q. Phylogenetic distribution and predominant genotype of the avian infectious bronchitis virus in China during 2008-2009. Virol J. 2011; 8:184.


13. Kang DH, Nakagawa T. Uric acid and chronic renal disease: Possible implication of hyperuricemia on progression of renal disease. Semin Nephrol. 2005; 25:43–49.


14. Lee CW, Brown C, Hilt DA, Jackwood MW. Nephropathogenesis of chickens experimentally infected with various strains of infectious bronchitis virus. J Vet Med Sci. 2004; 66:835–840.


15. Liu MD, Luo P, Wang ZJ, Fei Z. Changes of serum Tau, GFAP, TNF-α and malonaldehyde after blast-related traumatic brain injury. Chin J Traumatol. 2014; 17:317–322.
16. Many A, Hubel CA, Roberts JM. Hyperuricemia and xanthine oxidase in preeclampsia, revisited. Am J Obstet Gynecol. 1996; 174:288–291.


17. Naguib YM, Azmy RM, Samaka RM, Salem MF. Pleurotus ostreatus opposes mitochondrial dysfunction and oxidative stress in acetaminophen-induced hepato-renal injury. BMC Complement Altern Med. 2014; 14:494.


18. Nath KA, Norby SM. Reactive oxygen species and acute renal failure. Am J Med. 2000; 109:665–678.


19. Nemzer B, Chang T, Xie Z, Pietrzkowski Z, Reyes T, Ou B. Decrease of free radical concentrations in humans following consumption of a high antioxidant capacity natural product. Food Sci Nutr. 2014; 2:647–654.


20. Okamoto K, Kusano T, Nishino T. Chemical nature and reaction mechanisms of the molybdenum cofactor of xanthine oxidoreductase. Curr Pharm Des. 2013; 19:2606–2614.


21. Okino CH, dos Santos IL, Fernando FS, Alessi AC, Wang X, Montassier HJ. Inflammatory and cell-mediated immune responses in the respiratory tract of chickens to infection with avian infectious bronchitis virus. Viral Immunol. 2014; 27:383–391.


22. Peralta C, Bulbena O, Xaus C, Prats N, Cutrin JC, Poli G, Gelpi E, Roselló-Catafau J. Ischemic preconditioning: a defense mechanism against the reactive oxygen species generated after hepatic ischemia reperfusion. Transplantation. 2002; 73:1203–1211.


23. Pfeffer KD, Huecksteadt TP, Hoidal JR. Xanthine dehydrogenase and xanthine oxidase activity and gene expression in renal epithelial cells. Cytokine and steroid regulation. J Immunol. 1994; 153:1789–1797.
24. Poss WB, Huecksteadt TP, Panus PC, Freeman BA, Hoidal JR. Regulation of xanthine dehydrogenase and xanthine oxidase activity by hypoxia. Am J Physiol. 1996; 270:L941–L946.


25. Qian DH, Zhu GJ, Wu LZ, Hua GX. Isolation and characterization of a coronavirus from pigeons with pancreatitis. Am J Vet Res. 2006; 67:1575–1579.


26. Reed LJ, Muench H. A simple method of estimating fifty per cent endpoints. Am J Epidemiol. 1938; 27:493–497.
27. Reyazuddin M, Azmi SA, Islam N, Rizvi A. Oxidative stress and level of antioxidant enzymes in drug-naive schizophrenics. Indian J Psychiatry. 2014; 56:344–349.


28. Sato A, Nishino T, Noda K, Amaya Y, Nishino T. The structure of chicken liver xanthine dehydrogenase. cDNA cloning and the domain structure. J Biol Chem. 1995; 270:2818–2826.
29. Seifried HE, Anderson DE, Fisher EI, Milner JA. A review of the interaction among dietary antioxidants and reactive oxygen species. J Nutr Biochem. 2007; 18:567–579.


30. Singh N, Ghosh RC, Singh A. Prevalence and haemato-biochemical studies on naturally occurring gout in Chhattisgarh. Adv Anim Vet Sci. 2013; 1:3S. 9–11.
31. Taş S, Sarandöl E, Dirican M. Vitamin B6 supplementation improves oxidative stress and enhances serum paraoxonase/arylesterase activities in streptozotocin-induced diabetic rats. ScientificWorldJournal. 2014; 2014:351598.


32. Vorbach C, Harrison R, Capecchi MR. Xanthine oxidoreductase is central to the evolution and function of the innate immune system. Trends Immunol. 2003; 24:512–517.


33. Wideman RF Jr, Cowen BS. Effect of dietary acidification on kidney damage induced in immature chickens by excess calcium and infectious bronchitis virus. Poult Sci. 1987; 66:626–633.

