Abstract
Canine mesenchymal cells (MSCs) derived from Wharton's jelly were co-cultured, then supplemented or not supplemented with platelet rich plasma (PRP) and demineralized bone matrix (DBM) to verify osteogenic differentiation. Osteoblastic differentiation followed by mineralized bone matrix production was found to be significantly higher (p < 0.05) when MSCs were associated with PRP/DBM in culture after 14-21-days of induction. Osteopontin and osteocalcin gene expression were significantly superior (p < 0.05) under the same culture conditions after 21 days of observation. In conclusion, addition of PRP to DBM co-cultured with MSCs successfully induced osteogenesis in vitro.
Wharton's jelly-derived mesenchymal stem cells (MSCs) are characterized by their ability for self-renewal and capacity to differentiate into a variety of mesenchymal tissues such as bone, cartilage, fat, and muscle [2467101214]. For clinical scale application, MSCs are usually amplified in vitro, which currently occurs through medium containing fetal bovine serum (FBS) characterized as non-defined medium [26]. Platelet-rich plasma (PRP) represents an alternative safe growth medium to FBS [2]. PRP is defined as the portion of the plasma fraction of autologous blood having a platelet concentration above baseline [15]. PRP is a storage vehicle of growth factors such as platelets-derived growth factor (PDGF), transforming growth factor (TGF), platelet-derived angiogenesis factor (PDAF), insulin growth factor-1 (IGF-1), and platelet factor-4 (PF-4), which are known to influence bone regeneration [58101315]. Although it is widely accept that PRP promotes bone regeneration by facilitating osteogenic progenitor cells proliferation, the detailed mechanism remains unclear.
Reconstruction of bone defects is based on the use of autogenous bone grafts, either free or vascularized. Demineralized bone matrix (DBM) contains a great amount of bone morphogenetic proteins responsible for osteo-inductive potential in vivo and potential bone regeneration [911]. However, the ability of DBM to provoke bone mineralization is still unknown. Therefore, this study was conducted to obtain canine umbilical MSCs from Wharton's jelly and then co-culture MSCs with demineralized bone matrix and/or platelet-rich plasma to evaluate bone mineralization.
All experiments were performed under the guidelines of Colégio Brasileiro Experimentação Animal (COBEA; proc. 5671/13) of the University of São Paulo State. Five neonatal umbilical cords from were collected healthy female dogs with prior authorization from the owners. Wharton's jelly portion was obtained as previously described [26]. MSCs culture, phenotyping and multi-differentiation properties were evaluated, while PRP was obtained as previously described [246121415].
DBM was prepared after collected from a dead dog at College of Veterinary Medicine, Small Animal Surgery Center, UNESP, Araçatuba, São Paulo, Brazil. Demineralization was performed by immersion in 0.6 N hydrochloric acid at room temperature for 20 days. Demineralized bones were transversely sectioned (4.0 mm) and stored in 70% ethanol at room temperature for 30 days until use.
Four experiments were established in which 1 × 106 MSCs/mL were used: MSCs alone; MSCs + PRP, MSCs + DBM and MSCs + PRP + DBM. Each evaluation was conducted in six well culture plates, all of which contained DMEM-F12 medium supplemented with B-27 and XenoFree (50×; Gibco, USA). MSCs monolayers were observed at zero (0), seven (7), 14 and 21-days after initial culture. To determine extracellular mineralization, cells were fixed with 4% paraformaldehyde for 15 min, washed five times with ultra-pure water and allowed to dry at room temperature, after which they were stained with Alizarin red. All images were obtained using a digital camera DP73 attached to an IX 70 inverted microscope (Olympus, Japan). Images were analyzed using the cellSens software (Olympus). Alkaline phosphatase (ALP) activity was measured from culture supernatants in triplicate as previously described [9]. The results were calculated after determining the cutoff point, which was established by the arithmetic mean of the OD values of the negative controls (cells without induction).
Total cellular RNA was extracted from cells with the indicated treatments using Trizol reagent (Invitrogen, USA). cDNA synthesis was conducted using an Enhanced Avian RT First Strand Synthesis Kit (Sigma-Aldrich, USA). Primers for target genes and the internal control were as follows: osteopontin (forward)-5'-CATATGATGGCCGAGGTGATAG-3', (reverse)-5'CAAGTGATGTGAAGTCCTCCTC-3'; osteocalcin (forward)-5'GAGGGCAGCGAGGTGGTGAG-3', (reverse)-5'-TCAGCCAGCTCGTCACAGTTGG-3'; Runx2 ( forward)-5'TGGGAGAGGTACCAGATGGG-3', (reverse)-5'-TCTTGCCTCGTCCACTCCGG-3'; β-actin (forward)-5'GGGCCAGAAGGACTCCTACGT-3', (reverse)-5'-TGCTGTCCCTGTACGCCTCT-3'. cDNA from the RT reaction was used as the template in quantitative polymerase chain reaction (PCR) with the StepOne real time PCR system (Applied Biosystems, USA). Power SYBR Green PCR master mix was applied to amplify the cDNA (100 ng) according to the manufacturer's protocols. β-actin was used as a housekeeping gene to normalize the amount of mRNA. Gene expression was measured relative to that of the control. Fold change in gene expression relative to the control was calculated by the 2ΔΔCt method. All statistical analyses were performed using the SAS 9.1.2 software package (SAS Institute, USA.) and data are presented as the means ± standard deviation based on three replicates for each experiment. One-way analysis of variance (ANOVA) for multiple comparisons or a two tailed student t-test was used to identify significant differences between groups. A p < 0.05 was considered to indicate significance.
The results indicated that PRP and DBM both have strong effects on the differentiation of Wharton's jelly canine MSCs, which occurs at least partially through induction of ALP and osteocalcin/osteopontin gene expression. We also found that association of co-culture MSCs, PRP and DBM, played a much better role in promotion of osteogenic progenitor proliferation due to its ability to restrain the differentiation at 21 days after induction.
MSCs isolation and expansion showed typical morphology of undifferentiated mesenchymal cells with fusiform fibroblast-like and elongated appearance during all passages (panel A in Fig. 1). Mesenchymal cell phenotyping revealed CD44+, CD105+ and CD71+ (panels B-D in Fig. 1) cells, while they were negative for the hematopoietic cell phenotypic markers CD34- and CD45- (panels B and D in Fig. 1). These findings are similar to those of previous studies [2461214]. The optimum conditions were found to be co-culture of MSCs were with PRP and DBM, which produced the optimum ALP levels (panel A in Fig. 2). Several studies have described mitogenic effects of PRP in stem cells; however, this study was the first to demonstrate these effects in veterinary science [18]. In contrast, PRP has been reported to promote a decrease in ALP activity, despite increasing calcium levels [5]. ALP activity is not always directly correlated with the mineralization process [9]; however, in this study, a correlation between these two processes was confirmed (panel A in Fig. 2). No significant differences in Runx2 gene expression were observed during analysis (panel B in Fig. 2). The main role of Runx2 transcription factor is to promote chondrocytes and osteoblast differentiation, which is associated with bone formation [25]. Interestingly, when canine Whaton's jelly MSCs were co-cultured with PRP and DBM, osteocalcin and osteopontin genes were expressed in higher levels when compared to other combinations (panel B in Fig. 2). Osteocalcin is considered a late marker of osteogenesis, while osteopontin has been implicated as an important factor in bone remodeling [311]. These findings suggest that an association of PRP and DBM could improve MSCs differentiation into osteoblast cells and facilitate bone formation, as partially demonstrated here.
To expand the population of osteogenic progenitors would be advantageous for clinical application of MSCs to the reconstruction of massive bone defects. Mesenchymal cell expansion is currently conducted by adding fetal bovine serum or growth factors, which could lead to sanitary problems, especially infectious pathogen transmission [2]. Moreover, the PRP applied in this study are safer to use than homologous recombinant growth factors and is free from concerns regarding disease transmission [2].
Overall, the results of this study strongly suggest that PRP and DBM co-cultured with MSCs have the potential for use in an osteo-induction in vitro model with future clinical applications to avoid the risk of graft versus host disease, leading to better acceptance by patients. Further studies are ongoing to explore use of this in vitro model during orthopedic surgeries.
Figures and Tables
Fig. 1
(A) Fibroblast-like morphology of canine MSCs isolated Wharton's jelly at the 10th passage. Micrographs were taken under phase contrast microscopy. 40× magnification. MSCs phenotyping by flow cytometer analysis: (B) CD34-/CD44+, (C) CD45-/CD271+, (D) CD45-/CD105+. Data from surface markers were obtained by histograms generated using an Attune acoustic focusing cytometer, and auto-fluorescence was excluded as a global compensation tool (cutoff > 104). Y-axis corresponds to the number of positive labeled cells detected by BL1A filter (488 nm).
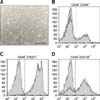
Fig. 2
(A) Alizarin Red staining showing extracellular matrix deposition and mineralization. 40× magnification. Alkaline phosphatase levels (405 nm; Y-axis) from control (MSCs)β, MSCs + PRP, MSCs + DBM, and MSCs + PRP + DBM groups at 0, 7, 14 and 21 days after culture (*p < 0.05). (B) Osteopontin, osteocalcin and Runx2 transcription in all studied groups at 0, 7, 14 and 21 days after culture.
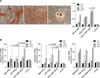
Acknowledgments
The authors thank the FAPESP (Fundação Amparo à Pesquisa do Estado de São Paulo; grant nos. 2010/52465-9 and 2011/50845-1) for their support. TC Cardoso is a recipient of CNPq (Brazilian Council for Research) funding.
References
1. Anitua E, Andia I, Ardanza B, Nurden P, Nurden AT. Autologous platelets as a source of proteins for healing and tissue regeneration. Thromb Haemost. 2004; 91:4–15.


2. Cardoso TC, Ferrari HF, Garcia AF, Novais JB, Silva-Frade C, Ferrarezi MC, Andrade AL, Gameiro R. Isolation and characterization of Wharton's jelly-derived multipotent mesenchymal stromal cells obtained from bovine umbilical cord and maintained in a defined serum-free three-dimensional system. BMC Biotechnol. 2012; 12:18.


3. Chaudhary LR, Hofmeister AM, Hruska KA. Differential growth factor control of bone formation through osteoprogenitor differentiation. Bone. 2004; 34:402–411.


4. Chung DJ, Hayashi K, Toupadakis CA, Wong A, Yellowley CE. Osteogenic proliferation and differentiation of canine bone marrow and adipose tissue derived mesenchymal stromal cells and the influence of hypoxia. Res Vet Sci. 2012; 92:66–75.


5. Devescovi V, Leonardi E, Ciapetti G, Cenni E. Growth factors in bone repair. Chir Organi Mov. 2008; 92:161–168.


6. Filioli Uranio MF, Valentini L, Lange-Consiglio A, Caira M, Guaricci AC, L'Abbate A, Catacchio CR, Ventura M, Cremonesi F, Dell'Aquila ME. Isolation, proliferation, cytogenetic, and molecular characterization and in vitro differentiation potency of canine stem cells from foetal adnexa: a comparative study by amniotic fluid, amnion; and umbilical cord matrix. Mol Reprod Dev. 2011; 78:361–373.


7. Handschel J, Naujoks C, Langenbach F, Berr K, Depprich RA, Ommerborn MA, Kübler NR, Brinkmann M, Kögler G, Meyer U. Comparison of ectopic bone formation of embryonic stem cells and cord blood stem cells in vivo. Tissue Eng Part A. 2010; 16:2475–2483.


8. Henderson JL, Cupp CL, Ross EV, Shick PC, Keefe MA, Wester DC, Hannon T, McConnell D. The effects of autologous platelet gel on wound healing. Ear Nose Throat J. 2003; 82:598–602.


9. Hoemann CD, El-Gabalawy H, McKee MD. In vitro osteogenesis assays: influence of the primary cell source on alkaline phosphatase activity and mineralization. Pathol Biol (Paris). 2009; 57:318–323.


10. Ishida K, Acharya C, Christiansen BA, Yik JHN, DiCesare PE, Haudenschild DR. Cartilage oligomeric matrix protein enhances osteogenesis by directly binding and activating bone morphogenetic protein-2. Bone. 2013; 55:23–35.


11. Kärner E, Unger C, Sloan AJ, Ährlund-Richter L, Sugars RV, Wendel M. Bone matrix formation in osteogenic cultures derived from human embryonic stem cells in vitro. Stem Cells Dev. 2007; 16:39–52.


12. Park SB, Seo MS, Kim HS, Kang KS. Isolation and characterization of canine amniotic membrane-derived multipotent stem cells. PLoS One. 2012; 7:e44693.


13. Pradeep AR, Shetty SK, Garg G, Pai S. Clinical effectiveness of autologous platelet-rich plasma and peptide-enhanced bone graft in the treatment of intrabony defects. J Periodontol. 2009; 80:62–71.

