Abstract
This study was conducted to investigate the effects of rapamycin treatment during in vitro maturation (IVM) on oocyte maturation and embryonic development after parthenogenetic activation (PA) and somatic cell nuclear transfer (SCNT) in pigs. Morphologically good (MGCOCs) and poor oocytes (MPCOCs) were untreated or treated with 1 nM rapamycin during 0-22 h, 22-42 h, or 0-42 h of IVM. Rapamycin had no significant effects on nuclear maturation and blastocyst formation after PA of MGCOCs. Blastocyst formation after PA was significantly increased by rapamycin treatment during 22-42 h and 0-42 h (46.6% and 46.5%, respectively) relative to the control (33.3%) and 0-22 h groups (38.6%) in MPCOCs. In SCNT, blastocyst formation tended to increase in MPCOCs treated with rapamycin during 0-42 h of IVM relative to untreated oocytes (20.3% vs. 14.3%, 0.05 < p < 0.1), while no improvement was observed in MGCOCs. Gene expression analysis revealed that transcript abundance of Beclin 1 and microtubule-associated protein 1 light chain 3 mRNAs was significantly increased in MPCOCs by rapamycin relative to the control. Our results demonstrated that autophagy induction by rapamycin during IVM improved developmental competence of oocytes derived from MPCOCs.
Pigs are used in a wide variety of biomedical studies as models of human disease, xenotransplantation, and transgenesis for medical application. However, the efficiency of in vitro production of embryos by reproductive biotechnology including in vitro fertilization (IVF), intracytoplasmic sperm injection, and somatic cell nuclear transfer (SCNT) procedures has not been thoroughly investigated in this species. To increase the efficiency of assisted reproductive technology in pigs, it is important to prepare mature oocytes with high developmental competence [14]. The quality of oocytes derived from in vitro maturation (IVM) is a key factor influencing successful embryonic development. Despite many studies to improve IVM systems for mammalian oocytes, relatively little is known about oocyte maturation. Indirect evidence, such as maturation-promoting factor activity, intraoocyte glutathione (GSH) content, and blastocyst formation after IVF and SCNT, are widely used to predict the degree of cytoplasmic maturation of IVM oocytes [891516]. However, morphological features such as thickness of the cumulus cell layer and oocyte diameter are still the most common criteria used for classification of the quality of immature cumulus-oocyte-complexes (COCs). The physiological importance of the role of gap junctions between oocytes and cumulus cells is well known [25]. Cumulus cells play an important role, particularly in normal cytoplasmic maturation of oocytes, regulation of oocyte metabolism, and protection of oocytes from harmful environments such as oxidative stress [452936]. For these reasons, morphologically poor oocytes (MPCOCs) that are smaller in diameter and have less cumulus cells than morphologically good oocytes (MGCOCs) are discarded.
Autophagy or autophagocytosis is a process that removes unnecessary or damaged cellular proteins and components [6]. This process also plays an important role in promoting cellular survival during starvation [21]. Mammalian target of rapamycin (mTOR) is a negative regulator of autophagy [7] that has been reported to be involved in the meiotic maturation of mouse oocytes by regulating the proliferative activity of cumulus cells [13]. As shown in various biological systems, recent evidence indicates that autophagy is involved in embryonic development in mammalian species. Autophagy-deficient mouse embryos die during preimplantation development, [34] and transient induction of autophagy augments the preimplantation development of bovine embryos [26]. Thus, chemical inhibitors of mTOR are frequently used to activate autophagy in mammalian cells [30]. Additionally, expression of several genes including Beclin 1 and microtubule-associated protein 1-light chain 3 (LC3) have been assayed to monitor autophagy in various types of cells [1337]. Beclin 1, which is the mammalian orthologue of the yeast Apg6/Vps30 gene, can complement the defect in autophagy present in apg6 yeast strains and stimulate autophagy when overexpressed in mammalian cells [18]. Expression of LC3, which is essential to the formation of autophagosomes, has also been analyzed to monitor autophagic activity [2737].
Rapamycin is an autophagy inducer that inhibits Ser/Thr protein kinase, which regulates cellular metabolism and growth in response to environmental stimuli and has shown both pro- and anti-apoptotic effects. Pan et al. [22] reported that rapamycin protected neuronal cells from apoptosis induced by rotenone treatment by inducing autophagy. In contrast, Tirado et al. [32] observed that rapamycin stimulated apoptosis of human desmoplastic small round cell tumor (JN-DSRCT-1) cells by increasing the Bax : Bcl-xl ratio. Although Song et al. [27] examined the effects of rapamycin in a maturation medium on subsequent development of in vitro-fertilized pig embryos, limited information is available regarding whether autophagy induction (in both MGCOCs and MPCOCs) by rapamycin treatment will show beneficial effects on oocyte maturation and preimplantation development in vitro following parthenogenetic activation (PA) and SCNT. Therefore, this study was conducted to examine the effects of rapamycin, an autophagy inducer, on oocyte maturation and embryonic development after PA and SCNT in pigs. Our results demonstrate that treatment with the autophagy inducing agent, rapamycin, during IVM improves developmental competence after PA and SCNT of MPCOCs in pigs, probably via stimulation of expression of autophagy-related genes.
All chemicals used in this study were obtained from Sigma-Aldrich Chemical Company (USA), unless otherwise noted. The base medium for IVM was medium-199 (M-199; Invitrogen, USA), which consisted of 0.6 mM cysteine, 0.91 mM pyruvate, 10 ng/mL epidermal growth factor, 75 µg/mL kanamycin, 1 µg/mL insulin, and 10% (v/v) pig follicular fluid. IVM medium was supplemented with 80 µg/mL FSH (Antrin R-10; Kyoritsu Seiyaku, Japan) and 10 IU/mL hCG (Intervet International BV, the Netherland) for the first 22 h of IVM. Porcine zygote medium (PZM)-3 containing 0.3% (w/v) bovine serum albumin (BSA) was used as the in vitro culture (IVC) medium for embryonic development, which was modified by adding 2.77 mM myo-inositol, 0.34 mM trisodium citrate, and 10 µM β-mercaptoetanol as previously described [39].
Pig ovaries of 6-7-month-old prepubertal gilts weighing 110 to 120 kg were obtained from a local abattoir and transported to the laboratory at 37℃ in saline. Follicular contents containing oocytes were aspirated from follicles 3 to 8 mm in diameter using an 18-gauge needle attached to a plastic syringe. Oocytes were classified into two groups of MGCOCs and MPCOCs according to the thickness of the cumulus cell layer of the oocytes (Fig. 1). Immature COCs were placed into each well of a four-well culture dish (Nunc 4-well Dishes for IVF; Thermo Scientific, USA) that contained 500 µL of maturation medium with hormones. The COCs were cultured at 39℃ with 5% CO2 under maximum humidity. After 22 h of maturation, oocytes were washed several times in fresh hormone-free IVM medium and then further cultured in hormone-free IVM medium for an additional 20 h. During IVM, the maturation medium was supplemented with 1 nM rapamycin according to the experimental design. We used a ready-made rapamycin solution (R-8781) in which rapamycin was dissolved in dimethyl sulfoxide (DMSO) (2.5 mg/mL). The final concentration of DMSO in the maturation medium was less than 0.0001% (v/v) when the medium was supplemented with 1 nM rapamycin. Thus, we disregarded the effects of DMSO included in the IVM medium. Following IVM, denuded oocytes were examined for nuclear maturation. Oocytes having their first polar bodies in the perivitelline space were considered meiotically matured.
MGCOCs and MPCOCs in Experiments 1 and 2, respectively, were untreated or treated with 1 nM rapamycin during 0-22, 22-42, and 0-42 h of IVM, and the effects of rapamycin treatment on nuclear maturation and embryonic development after PA were examined to determine the optimal timing of treatment. In Experiment 3, the effects of rapamycin treatment on intraoocyte content of GSH were examined. Finally, embryonic development after SCNT of MGCOCs and MPCOCs was compared following treatment of recipient cytoplasts with rapamycin during 0-42 h of IVM in Experiment 4. The proportions of oocytes that reached metaphase II (MII) stage and developed to the blastocyst stage were calculated based on the number of COCs cultured for maturation and the number of PA or SCNT oocytes cultured, respectively. Finally, the relative transcript abundance of Beclin 1 and LC3 mRNA was analyzed in Experiment 5 to determine whether rapamycin treatment stimulated expression of autophagy related genes.
Porcine fetal fibroblasts were cultured in four-well culture dishes in Dulbecco's modified Eagle medium using nutrient mixture F-12 (Invitrogen) amended with 10% (v/v) fetal bovine serum until a complete monolayer of cells formed. Synchronization of donor cells at the G0/G1 stage of the cell cycle was induced by contact inhibition for 72 to 96 h. Cultured cells were trypsinized, washed, and resuspended in HEPES-buffered Tyrode's medium containing 0.4% (w/v) BSA (TLH-BSA) [1] to prepare a suspension of single cells for nuclear transfer.
For SCNT, oocytes at the MII stage were placed in a manipulation medium (calcium-free TLH-BSA) containing 5 µg/mL Hoechst 33342 for 15 min. After incubation, oocytes were washed in a fresh manipulation medium and transferred into a droplet of manipulation medium containing 5 µg/mL cytochalasin B (CB) that was covered with warm mineral oil. The oocytes were enucleated by aspirating the polar body and MII chromosomes using a 17-µm beveled glass pipette (Humagen Micropipets; Origio, USA). Presence or absence of MII chromosomes was observed under an epifluorescence microscope (TE300; Nikon, Japan). A single cell was injected into the perivitelline space of each enucleated oocyte, after which cell-oocyte couplets were placed on a fusion chamber overlaid with 1 mL of 260 mM mannitol containing 0.001 mM CaCl2 and 0.05 mM MgCl2, as previously described [2835]. Membrane fusion of cell-oocyte couplets was induced by applying electric stimuli using a cell fusion generator (LF101; Nepa Gene, Japan) [17]. Oocytes were then incubated for 1 h in TLH-BSA, after which they were examined for fusion under a stereomicroscope. The SCNT oocytes were activated with two pulses of 120 V/mm direct current for 60 µs in a 280 mM mannitol solution containing 0.1 mM CaCl2 and 0.05 mM MgCl2. To induce parthenogenesis, MII oocytes were activated using the same pulse sequence that was used to activate SCNT oocytes [17].
After electrical activation, the PA and SCNT embryos were treated with 5 µg/mL CB and 0.4 µg/mL demecolcine, respectively, combined with 1.9 mM 6-dimethylaminopurine in IVC medium for 4 h [17]. The SCNT and PA embryos were washed three times in fresh IVC medium, transferred into 30-µL droplets of IVC medium under mineral oil, and then cultured at 39℃ in a humidified atmosphere of 5% CO2, 5% O2, and 90% N2 for 7 days. Cleavage and blastocyst formation were evaluated on days 2 and 7, respectively, with the day of SCNT or PA designated as day 0 [17]. The number of cells in blastocysts was examined by Hoechst 33342 staining under an epifluorescence microscope.
The intraoocyte GSH contents of IVM oocytes were measured using previously described methods [23]. Briefly, CellTracker Blue 4-chloromethyl-6,8-difluoro-7-hydroxycoumarin (Invitrogen) was used to detect intracellular GSH with blue fluorescence, respectively. A group of 10 to 15 denuded oocytes at the MII stage from each treatment group was incubated for 30 min in TLH containing 0.05% (w/v) polyvinyl alcohol (PVA) supplemented with 10 µM CellTracker in the dark. Oocytes were then washed three times, after which they were incubated for an additional 30 min in IVC medium in the dark. Following incubation, the oocytes were washed with D-PBS (Invitrogen) containing 0.1% (w/v) PVA, then placed into 2-µL droplets, and the fluorescence was observed under an epifluorescence microscope (TE300; Nikon) with ultraviolet ray filters (370 nm). The fluorescence intensities of oocytes were subsequently analyzed by the ImageJ software (ver. 1.46r; National Institutes of Health, USA) and normalized against untreated MPCOCs oocytes.
The extraction of total mRNA from denuded MII oocytes was conducted using the Dynabeads mRNA DIRECT Kit (Ambion, USA) according to the manufacturer's instructions. Reverse transcription of the extracted mRNA was performed using ReverTra Ace qPCR RT Master Mix with a gDNA Remover kit (Toyobo, Japan). Subsequently, the 7500 Real-Time PCR System (Applied Biosystems, USA) was used for PCR amplification with Real-time PCR Master Mix (Toyobo) to quantify the expression of specific genes. PCR specificity was identified by analyzing melting curve data and the specific gene expression was normalized by comparison to the GAPDH transcriptional level. Relative mRNA level was calculated as 2-ΔCt, where Ct = the threshold cycle for target amplification and ΔCt = Cttarget gene (specific genes for each sample) - Ctinternal reference (GAPDH for each sample). General information and sequences of primers designed with cDNA sequences derived from GenBank for pig and by the Primer3 software (Whitehead Institute/MIT Center for Genome Research, USA) are shown in Table 1.
Data from each experiment were analyzed using the general linear model procedure followed by the least square method when treatments differed at a p < 0.05 in the Statistical Analysis System (ver. 9.3; SAS Institute, USA). The results are expressed as the mean ± standard error of the mean (SEM).
When MGCOCs were treated with rapamycin during 0-22, 22-42 or 0-42 h of IVM, no significant difference in nuclear maturation was observed among groups examined (92.4, 90.1, 94.4 and, 89.2% for untreated oocytes and those treated for 0-22, 22-42, and 0-42 h of IVM, respectively). Rapamycin treatment during IVM did not alter PA embryonic development to the cleavage (89.4-94.5%) and blastocyst stages (48.5, 54.9, 51.1, and 48.8% for untreated oocytes and those treated for 0-22, 22-42, and 0-42 h of IVM, respectively) (Table 2). In addition, the number of cells in the blastocyst (37.1-38.7 cells per blastocyst) was not influenced by rapamycin treatment (Table 2).
Regardless of time of treatment, rapamycin did not improve nuclear maturation (82.4-86.6%) of MPCOCs, which was significantly lower than that (92.8%) of MGCOCs. In contrast to nuclear maturation of oocytes, significant effects of rapamycin were observed on embryonic development after PA. Blastocyst formation of oocytes treated with rapamycin during 22-42 h (46.6%) and 0-42 h of IVM (46.5%) was not significantly different from that of untreated MGCOCs (50.8%), but was higher than that of untreated (33.3%) oocytes and those treated for 0-22 h of IVM (38.6%). The number of cells in the blastocyst (36.2-38.7 cells per blastocyst) was not influenced by rapamycin treatment (Table 3).
Treatment of oocytes with rapamycin during IVM did not influence intraoocyte GSH contents in MII oocytes derived from MGCOCs (1.08 ± 0.04 and 0.97 ± 0.05 pixels/oocyte for untreated and treated oocytes, respectively) and MPCOCs (1.00 ± 0.07 and 1.03 ± 0.06 pixels/oocyte for untreated and treated oocytes, respectively) (Table 4).
When MII oocytes that were treated with rapamycin during IVM were used as recipient cytoplasts for SCNT, embryonic development to the blastocyst stage was not influenced by rapamycin treatment in MGCOCs (27.7% and 26.0% for untreated and treated oocytes, respectively). However, blastocyst formation of SCNT embryos derived from MPCOCs tended to increase in response to rapamycin treatment compared to non-treatment (20.3% vs. 14.3%, p < 0.1). There were no significant effects of rapamycin on embryo cleavage (89.4-94.5%) or number of cells in the blastocyst (37.7-41.6 cells) observed (Table 5).
Treatment of MPCOCs with rapamycin during IVM stimulated the expression of Beclin 1 and LC3 mRNAs in MII oocytes relative to the control (p < 0.05), while no significant effect was observed in MGCOCs after treatment with rapamycin (Fig. 2).
Oocyte quality is an important factor influencing early embryonic viability, establishment of pregnancy, fetal development, and even diseases in adulthood [14]. Thus, many studies have been conducted to ensure matured oocytes have good quality [1117]. Particularly, the normal nuclear and cytoplasmic maturation of oocytes is critically dependent on interactions with their cumulus cells [12]. Cumulus cells and oocytes are associated with the gap junction system. The gap junctions between the oocyte and cumulus cells enable transport of small molecules such as nutrients and messengers, which may be involved in regulating meiotic resumption of oocytes enclosed with cumulus cells [19]. In this study, MPCOCs showed lower competence of nuclear maturation than MGCOCs, which was consistent with the results of previous studies that showed maturation of denuded porcine oocytes was lower than that seen in intact oocytes [220] and that co-culture of denuded oocytes improved maturation status [10].
All cells have nutrient and energy substrate reserves to enable their survival during unfavorable situations such as starvation, hypoxia, and nutrient depletion. Autophagy plays an essential role in providing access to such nutrient stores and is known as a prosurvival pathway activated by certain stressors [33]. Upon activation of autophagy under stressful conditions, cells reuse organelles and aging proteins to produce energy or essential nutrients through sequestration and degradation within autophagosomes and autolysosomes [33]. In this study, rapamycin treatment significantly increased the relative transcript abundance of Beclin 1 and LC3 mRNAs in MPCOCs. However, the stimulating effect on gene expression was not observed in MGCOCs. These findings coincided well with the effects of rapamycin on embryonic development. We used 1 nM rapamycin to induce autophagy based on the results reported by Song et al. [27], who found that 1 nM rapamycin sufficiently induced autophagy in pig COCs. When autophagy was induced in MGCOCs by rapamycin treatment during IVM, no improvement in oocyte maturation and embryonic development was observed. However, our results were inconsistent with the finding that treatment of pig COCs with rapamycin for 0-22 h of IVM improved nuclear maturation and embryonic development after IVF [27]. Although direct comparison is not possible due to use of different IVM systems, including the source of immature oocytes and type of maturation medium, nuclear maturation of untreated MGCOCs was 92.4% in this study, while it was only 75.8% in a previous study by Song et al. [27]. Thus, it was likely that the quality of immature oocytes used in this study was too high to be improved by autophagy induction. Another probable reason for the difference between previous and present studies was that the 1 nM rapamycin might not be the optimal concentration in our IVM system.
Interestingly, in contrast to the lack of a beneficial effect of rapamycin in MGCOCs, beneficial effects of autophagy induction by rapamycin treatment were shown in MPCOCs. Neither nuclear nor cytoplasmic maturation improved, but embryonic development to the blastocyst stage after PA and SCNT was increased by rapamycin treatment during IVM. Although it was not clear how or why autophagy was stimulated only in MPCOCs in this study (based on the expression of autophagy-related genes), it is likely that MPCOCs were exposed to poorer conditions during oocyte maturation, such as insufficient nutrients transportation to oocytes due to less cumulus cell attachment. As a result, the effects of autophagy induction in oocytes were more prominent than in MGCOCs. In pigs and cattle, intraoocyte GSH content increases while oocytes mature [338]. It is well known that intraoocyte GSH improves development of IVF or SCNT embryos by neutralizing reactive oxygen species, thereby protecting oocytes or embryos from oxidative stress [2431]. Therefore, intraoocyte GSH contents were measured in MII oocytes to determine whether the increased developmental competence of MPCOCs was due to improved cytoplasmic maturation after rapamycin treatment. GSH contents did not differ between untreated and treated oocytes. However, the results of this study did not clarify whether the improved developmental competence of MPCOCs was attributed to the induction of autophagy or other unknown effects of rapamycin on cytoplasmic maturation. Further studies are needed to determine the effects of autophagy induction on the expression of cytoplasmic factor(s) beneficial for embryonic development. In summary, our results demonstrate that rapamycin-assisted IVM leads to efficient production of MII oocytes and increased blastocyst formation. These findings will be useful for large-scale production of MII oocytes from MPCOCs and embryos having high developmental competence in vitro.
Figures and Tables
Fig. 1
(A) Morphologically good (MGCOCs) and (B) poor pig oocytes (MPCOCs) used in this study. MGCOCs were surrounded by a thick cumulus cell layer, whereas MPCOCs were partially denuded or had a thin cumulus cell layer compared to MGCOCs. Scale bars = 100 µm.
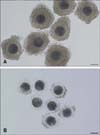
Fig. 2
Relative transcript abundance (mean ± SEM) of Beclin 1 and LC3 mRNAs in metaphase II oocytes derived from morphologically good oocytes (MGCOCs) and morphologically poor oocytes (MPCOCs) treated with 1 nM rapamycin during in vitro maturation. Bars with different letters (a, b) in the same mRNA are significantly different (p < 0.05).
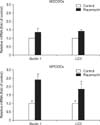
Table 1
Primers and real-time polymerase chain reaction conditions used for gene expression analysis
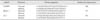
Table 2
Effects of rapamycin in maturation medium on oocyte maturation and development after parthenogenetic activation (PA) of morphologically good oocytes (MGCOCs)
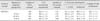
Table 3
Effects of rapamycin in maturation medium on oocyte maturation and development after PA of morphologically poor oocytes (MPCOCs)
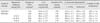
Table 4
Effects of rapamycin in maturation medium on oocyte maturation and intraoocyte glutathione (GSH) contents of MGCOCs and MPCOCs

Acknowledgments
This study was supported by the Bio-industry Technology Development Program (IPET 112015-4), Ministry of Agriculture, Food and Rural Affairs and the Basic Science Research Program through the National Research Foundation of Korea (NRF) funded by the Ministry of Education (grant no. NRF-2012R1A1A4A01002564).
References
1. Bavister BD, Leibfried ML, Lieberman G. Development of preimplantation embryos of the golden hamster in a defined culture medium. Biol Reprod. 1983; 28:235–247.


2. Bing YZ, Hirao Y, Iga K, Che LM, Takenouchi N, Kuwayama M, Fuchimoto D, Rodriguez-Martinez H, Nagai T. In vitro maturation and glutathione synthesis of porcine oocytes in the presence or absence of cysteamine under different oxygen tensions: role of cumulus cells. Reprod Fertil Dev. 2002; 14:125–131.


3. de Matos DG, Furnus CC, Moses DF. Glutathione synthesis during in vitro maturation of bovine oocytes: role of cumulus cells. Biol Reprod. 1997; 57:1420–1425.


4. Eppig JJ, Pendola FL, Wigglesworth K, Pendola JK. Mouse oocytes regulate metabolic cooperativity between granulosa cells and oocytes: amino acid transport. Biol Reprod. 2005; 73:351–357.


5. Fatehi AN, Roelen BA, Colenbrander B, Schoevers EJ, Gadella BM, Beverst MM, van den Hurk R. Presence of cumulus cells during in vitro fertilization protects the bovine oocyte against oxidative stress and improves first cleavage but does not affect further development. Zygote. 2005; 13:177–185.


6. Ferraro E, Cecconi F. Autophagic and apoptotic response to stress signals in mammalian cells. Arch Biochem Biophys. 2007; 462:210–219.


7. Foster KG, Fingar DC. Mammalian target of rapamycin (mTOR): conducting the cellular signaling symphony. J Biol Chem. 2010; 285:14071–14077.


8. Funahashi H, Kim NH, Stumpf TT, Cantley TC, Day BN. Presence of organic osmolytes in maturation medium enhances cytoplasmic maturation of porcine oocytes. Biol Reprod. 1996; 54:1412–1419.


9. Hashimoto N, Kishimoto T. Regulation of meiotic metaphase by a cytoplasmic maturation-promoting factor during mouse oocyte maturation. Dev Biol. 1998; 126:242–252.


10. Ju S, Rui R. Effects of cumulus cells on in vitro maturation of oocytes and development of cloned embryos in the pig. Reprod Domest Anim. 2012; 47:521–529.


11. Kim J, You J, Hyun SH, Lee G, Lim J, Lee E. Developmental competence of morphologically poor oocytes in relation to follicular size and oocyte diameter in the pig. Mol Reprod Dev. 2010; 77:330–339.


12. Kim KS, Minami N, Yamada M, Utsumi K. Follicular cells affect the fertilizability and developmental competency of bovine oocytes in vitro. Reprod Fertil Dev. 1997; 9:763–766.


13. Kogasaka Y, Hoshino Y, Hiradate Y, Tanemura K, Sato E. Distribution and association of mTOR with its cofactors, raptor and rictor, in cumulus cells and oocytes during meiotic maturation in mice. Mol Reprod Dev. 2013; 80:334–348.


14. Krisher RL. The effect of oocyte quality on development. J Anim Sci. 2004; 82:Suppl. E14–E23.
15. Kubelka M, Anger M, Kalous J, Schultz RM, Motlík J. Chromosome condensation in pig oocytes: lack of a requirement for either cdc2 kinase or MAP kinase activity. Mol Reprod Dev. 2002; 63:110–118.


16. Kubelka M, Rimkeviĉová Z, Guerrier P, Motlík J. Inhibition of protein synthesis affects histone H1 kinase, but not chromosome condensation activity, during the first meiotic division of pig oocytes. Mol Reprod Dev. 1995; 41:63–69.


17. Lee J, You J, Lee GS, Hyun SH, Lee E. Pig oocytes with a large perivitelline space matured in vitro show greater developmental competence after parthenogenesis and somatic cell nuclear transfer. Mol Reprod Dev. 2013; 80:753–762.


18. Liang XH, Jackson S, Seaman M, Brown K, Kempkes B, Hibshoosh H, Levine B. Induction of autophagy and inhibition of tumorigenesis by beclin 1. Nature. 1999; 402:672–676.


19. Luciano AM, Lodde V, Beretta MS, Colleoni S, Lauria A, Modina S. Developmental capability of denuded bovine oocyte in a co-culture system with intact cumulus-oocyte complexes: role of cumulus cells, cyclic adenosine 3',5' -monophosphate, and glutathione. Mol Reprod Dev. 2005; 71:389–397.


20. Maedomari N, Kikuchi K, Ozawa M, Noguchi J, Kaneko H, Ohnuma K, Nakai M, Shino M, Nagai T, Kashiwazaki N. Cytoplasmic glutathione regulated by cumulus cells during porcine oocyte maturation affects fertilization and embryonic development in vitro. Theriogenology. 2007; 67:983–993.


21. Othman EQG, Kaur G, Mutee AF, Muhammad TST, Tan ML. Immunohistochemical expression of MAP1LC3A and MAP1LC3B protein in breast carcinoma tissues. J Clin Lab Anal. 2009; 23:249–258.


22. Pan T, Rawal P, Wu Y, Xie W, Jankovic J, Le W. Rapamycin protects against rotenone-induced apoptosis through autophagy induction. Neuroscience. 2009; 164:541–551.


23. Sakatani M, Suda I, Oki T, Kobayashi S, Kobayashi S, Takahashi M. Effects of purple sweet potato anthocyanins on development and intracellular redox status of bovine preimplantation embryos exposed to heat shock. J Reprod Dev. 2007; 53:605–614.


24. Salmen JJ, Skufca F, Matt A, Gushansky G, Mason A, Gardiner CS. Role of glutathione in reproductive tract secretions on mouse preimplantation embryo development. Biol Reprod. 2005; 73:308–314.


25. Santiquet NW, Develle Y, Laroche A, Robert C, Richard FJ. Regulation of gap-junctional communication between cumulus cells during in vitro maturation in swine, a gap-FRAP study. Biol Reprod. 2012; 87:46.
26. Song BS, Yoon SB, Kim JS, Sim BW, Kim YH, Cha JJ, Choi SA, Min HK, Lee Y, Huh JW, Lee SR, Kim SH, Koo DB, Choo YK, Kim HM, Kim SU, Chang KT. Induction of autophagy promotes preattachment development of bovine embryos by reducing endoplasmic reticulum stress. Biol Reprod. 2012; 87:8.
27. Song BS, Kim JS, Kim YH, Sim BW, Yoon SB, Cha JJ, Choi SA, Yang HJ, Mun SE, Park YH, Jeong KJ, Huh JW, Lee SR, Kim SH, Kim SU, Chang KT. Induction of autophagy during in vitro maturation improves the nuclear and cytoplasmic maturation of porcine oocytes. Reprod Fertil Dev. 2014; 26:974–981.


28. Song K, Hyun SH, Shin T, Lee E. Post-activation treatment with demecolcine improves development of somatic cell nuclear transfer embryos in pigs by modifying the remodeling of donor nuclei. Mol Reprod Dev. 2009; 76:611–619.


29. Su J, Wang Y, Li R, Peng H, Hua S, Li Q, Quan F, Guo Z, Zhang Y. Oocytes selected using BCB staining enhance nuclear reprogramming and the in vivo development of SCNT embryos in cattle. PLoS One. 2012; 7:e36181.
30. Sully K, Akinduro O, Philpott MP, Naeem AS, Harwood CA, Reeve VE, O'Shaughnessy RF, Byrne C. The mTOR inhibitor rapamycin opposes carcinogenic changes to epidermal Akt1/PKBXMLLink_XYZ isoform signaling. Oncogene. 2013; 32:3254–3262.


31. Tatemoto H, Sakurai N, Muto N. Protection of porcine oocytes against apoptotic cell death caused by oxidative stress during in vitro maturation: role of cumulus cells. Biol Reprod. 2000; 63:805–810.


32. Tirado OM, Mateo-Lozano S, Notario V. Rapamycin induces apoptosis of JN-DSRCT-1 cells by increasing the Bax : Bcl-xL ratio through concurrent mechanisms dependent and independent of its mTOR inhibitory activity. Oncogene. 2005; 24:3348–3357.


33. Tsukada M, Ohsumi Y. Isolation and characterization of autophagy-defective mutants of Saccharomyces cerevisiae. FEBS Lett. 1993; 333:169–174.


34. Tsukamoto S, Kuma A, Mizushima N. The role of autophagy during the oocyte-to-embryo transition. Autophagy. 2008; 4:1076–1078.


35. Walker SC, Shin T, Zaunbrecher GM, Romano JE, Johnson GA, Bazer FW, Piedrahita JA. A highly efficient method for porcine cloning by nuclear transfer using in vitro-matured oocytes. Cloning Stem Cells. 2002; 4:105–112.


36. Wongsrikeao P, Kaneshige Y, Ooki R, Taniguchi M, Agung B, Nii M, Otoi T. Effect of the removal of cumulus cells on the nuclear maturation, fertilization and development of porcine oocytes. Reprod Domest Anim. 2005; 40:166–170.


37. Xu YN, Shen XH, Lee SE, Kwon JS, Kim DJ, Heo YT, Cui XS, Kim NH. Autophagy influences maternal mRNA degradation and apoptosis in porcine parthenotes developing in vitro. J Reprod Dev. 2012; 58:576–584.

