Abstract
This study was conducted to determine whether serum adipokine concentrations differed between healthy dogs and dogs with diabetes mellitus (DM). To accomplish this, 19 dogs with newly diagnosed DM were compared to 20 otherwise healthy dogs. The serum concentrations of visfatin, leptin, IL-1β, IL-6, IL-18, and TNF-α were significantly higher in diabetic dogs than in healthy dogs, whereas the serum adiponectin concentrations were lower in diabetic dogs. However, there were no significant differences in the IL-10 and resistin levels between groups. The serum leptin concentrations in diabetic dogs with and without concurrent disorders differed significantly. Treatment with insulin induced a significant decrease in IL-6 in diabetic dogs without concurrent disorders. These results show that the clinical diabetic state of dogs could modulate the circulating visfatin and adiponectin concentrations directly, while upregulation of leptin was probably a result of concurrent disorders rather than an effect of persistent hyperglycemia as a result of DM.
Diabetes mellitus (DM) is a common endocrinopathy in dogs [11] that is characterized by persistent hyperglycemia caused by a relative or absolute insulin deficiency. The most common form in dog resembles type 1 DM in humans [1131]. The mainstay of the clinical treatment of DM in dogs is insulin therapy, and its clinical signs include polydipsia, polyuria, and weight loss, which are associated with hyperglycemia and glucosuria [31]. Multiple factors might contribute to the etiology of canine DM [1114], including obesity, diet, exposure to toxic chemicals or drugs that cause insulin resistance, immune-mediated destruction of islet cells, and the destruction of islet cells secondary to pancreatitis [5111438].
In general, adipokines are defined as biologically active substances produced in adipose tissue that act in an autocrine/paracrine or endocrine manner [2939]. In human medicine, adipokines have attracted a tremendous amount of scientific interest because of the dramatic rise in obesity and its metabolic sequelae during the past few decades [728]. Evidence suggests that adipokines may contribute to the regulation of diverse biological processes, including inflammation and immune functions [33], hemostasis and vascular biology [23], hematopoiesis [10], and cellular proliferation and angiogenesis [2939]. In humans, several studies of metabolic diseases have reported the clinical implications of adipokines such as leptin, adiponectin, resistin, and visfatin, as well as cytokines such as tumor necrosis factor (TNF)-α and interleukin (IL)-6 [728].
It is unclear whether the dysregulation of adipokines is a cause or a result of DM in humans [535], but elevated concentrations of various adipokines and cytokines (TNF-α [35], IL-6 [27], and resistin [25]) have been reported in the circulatory systems of humans and rodents with insulin resistance. In contrast, two other adipokines, leptin and adiponectin, are known to increase insulin sensitivity and improve the effects of insulin in rodents [8]. Several clinical studies have suggested that serum adipokines might be related to the pathophysiology of diabetic humans [59]. However, there is a lack of well-documented studies of the relationship between adipokines and DM in dogs. A recent study proposed that the dysregulation of adipokines might be related to the pathogenesis of diabetic ketoacidosis (DKA) in dogs [26], but there is still a lack of information regarding the roles of adipokines in the pathogenesis of DM in dogs. Therefore, this study investigated whether the concentrations of serum adipokines differed in healthy and diabetic dogs with or without concurrent disorders.
Seventy-five dogs with newly diagnosed, untreated DM were enrolled in this prospective, observational cohort study. Thirty-six dogs were selected based on their body condition score (BCS; nine-point scale), which was 4/9. Nine dogs with complications of diabetes such as DKA or hyperosmolar hyperglycemic syndrome were excluded from this study, as were eight intact female dogs because the production of adipokines may be affected by reproductive status. Thus, only 19 dogs with DM were included in this study. Twenty healthy client-owned dogs with the same BCS scores (4/9) were included as controls. The healthy dogs were recruited from dogs that presented for health examination at the same veterinary medical center. Informed consent was obtained from the owners and the University Ethics Committee approved all of the animal studies.
Diagnosis of DM was based on the history of polyuria, polydipsia, weight loss despite good appetite, persistent glycosuria, increased serum fructosamine concentration (IDEXX Laboratories, Korea), and a persistent fasting blood glucose concentration > 250 mg/dL [2231]. The clinical signs or presence of concurrent disease did not affect study inclusion or exclusion. Dogs in the healthy group were considered to be healthy based on physical examination, indirect measurement of their systolic blood pressure, examination of fecal specimens to determine the presence of parasites using a flotation technique, heartworm antigen testing, complete blood count analysis, serum biochemical analysis, urinalysis, adrenocorticotropic hormone response testing, and diagnostic imaging, including survey radiography and abdominal ultrasonography. In addition, diabetic dogs were clarified based on a physical examination, indirect measurement of their systolic blood pressure, examination of fecal specimens to determine the presence of parasites using a flotation technique, heartworm antigen testing, complete blood count analysis, serum biochemical analysis, urinalysis, adrenocorticotropic hormone response testing, thyroid function testing, and diagnostic imaging, including survey radiography and abdominal ultrasonography.
All of the dogs were fasted for ≥ 12 h prior to blood collection. Serum was separated from clotted whole blood by centrifugation at 1,200 × g for 10 min within 1 h of blood collection and stored at -80℃ until the assay. The following adipokines were measured: leptin, visfatin, adiponectin, resistin, IL-1β, IL-6, IL-10, IL-18, and TNF-α. Serum leptin was analyzed in duplicate according to the manufacturer's protocol using a canine-specific ELISA kit (Canine Leptin ELISA kit; Millipore, USA), where the intra-assay variability was 4%, the inter-assay variability was 6%, and the leptin assay sensitivity was 0.4 ng/mL. The serum visfatin concentrations were measured using a canine-specific ELISA kit (Canine Visfatin ELISA kit; Tsz Biosciences, USA), where the intra- and inter-assay variabilities were 3% and 9%, respectively, and the assay sensitivity was 0.2 ng/mL. Serum resistin was measured using a canine-specific ELISA kit (Canine Resistin ELISA kit; Tsz Biosciences), where the intra-and inter-assay variabilities were < 5% and < 7%, respectively, according to the manufacturer's instructions. Serum adiponectin was measured using a canine-specific ELISA kit (Canine Adiponectin ELISA kit; Millipore), where the intra- and inter-assay variabilities were < 5% and < 3%, respectively, and the assay sensitivity was 0.03 µg/mL. Serum IL-1β was measured using a canine-specific ELISA kit (Canine ELISA kit; USCN Life Sciences, China), where the intra- and inter-assay variabilities were < 10% and < 12%, respectively, and the assay sensitivity was 6.4 pg/mL. The optical density was determined at 450 nm using an automated microplate reader (ELx 808; BioTek instruments, USA). The serum IL-6, IL-10, IL-18, and TNF-α levels were measured in duplicate using a Milliplex MAP Canine kit (Canine Cytokine/Chemokine MAGNETIC kit; Millipore). The intraand inter-assay variabilities of all the variables were < 5% and < 15%, respectively, and the assay sensitivities of IL-6, IL-10, IL-18, and TNF-α were 3.7, 8.5, 5.8, and 6.1 pg/mL, respectively. The assays were determined using Luminex (Luminex 200; Luminex, USA). All of the samples, standards, and controls were assayed in duplicate.
All of the dogs diagnosed with DM received recombinant human neutral protamine Hagedorn (NPH) insulin (Humulin N; Eli Lilly and Company, Korea). The initial dose of NPH insulin was 0.25 IU/kg, which was administered subcutaneously every 12 h. Subsequently, adjustments in the insulin dose were made by the managing clinician for each dog based on the dog's body weight and response to the previous insulin dose on the glucose curve with the goal of maintaining the blood glucose concentration between 80 and 300 mg/dL, and the lowest blood glucose concentration between 80 and 150 mg/dL [2231].
To evaluate the circulating adipokine concentrations after insulin treatment, a second round of testing was conducted when the dogs were judged to be clinically well controlled based on improvement or disappearance of clinical signs and the blood glucose concentration [2231]. To exclude the effects of other factors such as hypercortisolemia, only eight diabetic dogs without concurrent diseases were selected. The blood glucose concentrations of all eight dogs were within the target ranges after 12 weeks of treatment with NPH insulin, at which time they were reassessed to determine whether their adipokine concentrations had been affected by insulin treatment.
Data were analyzed using a commercially available statistical program (GraphPad Prism 6; GraphPad Software, USA). Normality tests (D'Agostino-Pearson omnibus test) were performed to determine whether the data were normally distributed. Mann-Whitney U tests were used to identify differences between diabetic dogs with or without concurrent diseases and healthy dogs, and the data were expressed as medians (ranges). The p values were calculated for two-tailed tests and the 95% confidence intervals (CIs) of the differences between medians were determined. Wilcoxon matched-pairs signed rank tests were used to compare the results for diabetic dogs before and after insulin treatment, and the data were expressed as medians (ranges) and 95% CIs. Parameters that did not satisfy a normal distribution were transformed mathematically to improve their symmetry before subsequent analyses. The associations among variables were tested by univariable linear regression analysis. For all comparisons, a p < 0.05 was considered significant.
This study included 20 healthy dogs and 19 dogs with DM (Table 1). Overall, 11 of the diabetic dogs had at least one concurrent disease. Eight of the 11 diabetic dogs with concurrent disease(s) had acute pancreatitis (AP) concurrent with chronic kidney disease (CKD) or hyperadrenocorticism (HAC). Specifically, one dog had AP only, four dogs had AP and CKD, and three dogs had AP and HAC. Three of the remaining 11 diabetic dogs had HAC, CKD, or myxomatous mitral valve disease (MMVD), one had CKD, one had HAC, and one had HAC and MMVD. Three of five dogs with HAC were treated for HAC prior to the DM episode. There were no significant differences in body weight or age of healthy and diabetic dogs, regardless of concurrent diseases.
The serum leptin (95% CI = -0.02 to 2.39, p = 0.0498) and visfatin (95% CI = 0.05 to 0.11, p = 0.0006) concentrations were significantly higher in diabetic dogs than healthy dogs, whereas the serum adiponectin (95% CI = -12.2 to -3.58, p < 0.0001) concentration was significantly lower in diabetic dogs than healthy dogs (Fig. 1). However, the serum resistin (95% CI = -2.15 to 22.98, p = 0.1005) concentrations did not differ significantly between diabetic and healthy dogs. Serum IL-1β, IL-6, and TNF-α were not detected in the majority of the healthy dogs, but were detected in several diabetic dogs (Table 2). The serum IL-18 (95% CI = 4.70 to 63.6, p = 0.0070) concentration was significantly higher in dogs with DM than healthy dogs, whereas the serum IL-10 (95% CI = -20.4 to 5.30, p = 0.2060) concentration did not differ significantly between the two groups. Univariate linear regression did not detect significant associations between the blood glucose concentrations and any of the log-transformed hormone concentrations in healthy and diabetic dogs.
The diabetic dogs were divided into two groups to determine whether the adipokine levels might have been affected by concurrent diseases, those with DM alone and diabetic dogs with concurrent disease. The serum leptin concentrations were significantly higher in diabetic dogs with concurrent diseases (95% CI = 0.19 to 4.03, p = 0.0157) than in diabetic dogs without concurrent diseases (Fig. 2). The serum leptin concentration was also significantly higher in diabetic dogs with concurrent diseases (95% CI = 0.86 to 3.81, p = 0.0009) than healthy dogs. However, the leptin (95% CI = -1.07 to 1.66, p = 0.5315) concentration did not differ significantly between diabetic dogs without concurrent diseases and healthy dogs.
The serum visfatin (95% CI = -0.03 to 0.09, p = 0.6415) and adiponectin (95% CI = -3.46 to 2.13, p = 0.2363) concentrations did not differ significantly between diabetic dogs with and without concurrent diseases. However, the visfatin concentrations were significantly increased (95% CI = 0.04 to 0.12, p = 0.0070; 95% CI = 0.02 to 0.12, p = 0.0060, respectively) and the adiponectin concentrations were significantly decreased (95% CI = -13.0 to -2.26, p = 0.0008; 95% CI = -13.3 to -3.52, p < 0.0001, respectively) in diabetic dogs with or without concurrent disorders. The serum resistin concentrations did not differ significantly among the three groups (healthy dogs vs diabetic dogs without concurrent diseases, 95% CI = -18.7 to 28.1, p = 0.8143; healthy dogs vs diabetic dogs with concurrent diseases, 95% CI = -11.5 to 23.6, p = 0.5763; diabetic dogs without concurrent diseases vs diabetic dogs with concurrent diseases, 95% CI = -27.4 to 35.3, p = 0.5278).
The serum IL-1β (95% CI = -25.9 to 62.0, p = 0.6364), IL-10 (95% CI = -19.3 to 25.2, p = 0.9494) and IL-18 (95% CI = -2.20 to 170, p = 0.0588) concentrations did not differ significantly between diabetic dogs with and without concurrent diseases (Table 3). Serum TNF-α was only detected in only one diabetic dog without concurrent diseases, whereas it was detected in nine of the 11 diabetic dogs with concurrent diseases. However, the serum IL-6 (95% CI = 17.6 to 85.6, p = 0.0084) concentration was significantly higher in diabetic dogs with concurrent diseases compared with diabetic dogs without concurrent diseases. In addition, the serum IL-18 (95% CI = 17.3 to 87.5, p = 0.0010) concentration was significantly higher in diabetic dogs with concurrent diseases than in healthy dogs.
Twelve weeks after the initiation of insulin therapy, the clinical signs, including polyuria, polydipsia, and polyphagia, had resolved in the eight diabetic dogs without concurrent diseases. The body weights and BCS did not differ significantly. There was a significant decrease in the serum IL-6 (95% CI = -64.90 to -4.00, p = 0.0020) concentration after treatment with NPH insulin relative to before treatment, but there were no significant differences in the other analytes.
Previous studies have shown that leptin might be associated with obesity in humans and dogs [162437]. Therefore, diabetic dogs and healthy dogs with the same BCS (4/9) were enrolled in the present study to exclude the effects of adiposity on circulating leptin concentrations. In the present study, the median leptin concentrations between healthy dogs and diabetic dogs were similar, but the serum leptin concentrations were higher in diabetic dogs in the presence of concurrent diseases. There were no differences in serum leptin concentrations of healthy and diabetic dogs without concurrent diseases. In addition, univariate linear regression did not identify any associations between blood glucose concentrations and the log-transformed leptin concentrations, and there was no difference in leptin concentrations before and after treatment with insulin. These results suggest that the increased leptin concentration in diabetic dogs might have been caused by concurrent diseases, instead of being a direct effect of hyperglycemia due to DM. Previous studies have also provided evidence of the dysregulation of serum leptin concentrations by other specific diseases [3617]. A recent study of dogs with pituitary-dependent hyperadrenocorticism suggested that higher circulating leptin concentrations might be associated with hypercortisolemia [6]. In humans, several studies have shown that leptin concentrations were higher in patients with acute pancreatitis [17] and chronic kidney disease [3]. In the present study, AP (8/11, 72.7%), HAC (5/11, 45.5%), and CKD (5/11, 45.5%) were confirmed as concurrent disorders in diabetic dogs. However, further studies will be required to fully understand the regulation of serum leptin concentrations in diabetic dogs.
Previous studies have not investigated serum visfatin concentrations in dogs. There is also little information available regarding the dysregulation of visfatin in human type 1 DM and it remains controversial [121]. In the present study, the circulating visfatin concentrations were upregulated in diabetic dogs, regardless of concurrent diseases. Visfatin, which was first isolated from the visceral fat of humans and mice, exhibits an insulin-like action by binding to the insulin receptor [13]. Because of the blood glucose-lowering actions of visfatin, some studies have suggested that increased plasma visfatin concentrations may be a compensatory mechanism in response to hyperglycemia [91521]. It has also been suggested that visfatin is an extracellular nicotinamide phosphoribosyltransferase enzyme that plays an important role in insulin secretion from pancreatic β-cells via systemic nicotinamide adenine dinucleotide biosynthesis in humans [30]. Therefore, the upregulated visfatin concentrations in diabetic dogs might be associated with a mechanism that compensates for β-cell functioning.
It is well known that hyperglycemia associated with insulin resistance, or a lack of insulin can modulate cytokine production by immune cells and a variety of host immune functions in humans and rodents [218]. The present study analyzed proinflammatory cytokines and revealed that the circulating concentrations of IL-1β and IL-6 were higher in diabetic dogs, despite their lack of concurrent diseases. However, there were no significant differences in the other analytes. These findings suggest that DM in dogs might trigger a proinflammatory state as a consequence of persistent hyperglycemia. However, the higher levels of IL-1β and IL-6 might also be involved in the pathogenesis of naturally occurring canine DM.
The present study also showed that the circulating adiponectin levels were downregulated in non-obese diabetic dogs, regardless of concurrent diseases. In contrast, previous studies showed that the plasma adiponectin levels were higher in humans with type 1 DM or insulin-dependent DM [1232]. The plasma adiponectin levels of humans are known to be negatively correlated with insulin resistance or type 2 DM and metabolic syndrome [20], and the lower adiponectin concentrations in obesity are generally considered to be a key component of insulin resistance [1920]. However, the development of DM in dogs is mainly associated with absolute insulin deficiency rather than insulin resistance [414]. This difference in the adiponectin concentrations of diabetic patients is probably related to differences in the adiponectin physiology of dogs and humans [3638]. A recent study showed that the serum adiponectin concentrations were not lower in dogs with obesity and not associated with obesity-associated changes in insulin sensitivity [37]. Thus, further quantitative assessments will be necessary in dogs with DM because the roles of adiponectin in dogs are still poorly understood.
It is well known that resistin expression can be upregulated in humans with type 1 DM, type 2 DM, obesity, and inflammatory diseases [34], but there is only one report of elevated serum resistin concentrations in dogs with diabetic ketoacidosis, although the subject did not have uncomplicated DM [26]. However, in the present study, there was no difference in the resistin concentrations of healthy dogs and diabetic dogs, regardless of the presence of concurrent diseases.
This study was originally designed as a pilot study and therefore had several limitations. One was the small number of dogs with DM, which limited the reliability of the negative findings, such as the nonsignificant difference in the resistin and IL-10 concentrations; however, this limitation does not reduce the validity of the positive findings. Another limitation was that the dogs in this study had concurrent diseases which could have affected the concentrations of adipokines. Therefore, the possibility that concurrent diseases might have affected both the positive and negative findings cannot be excluded completely. Furthermore, the lack of differences between diabetic dogs with concurrent diseases and healthy dogs or diabetic dogs without concurrent diseases might have been due to biological variations caused by the various concurrent diseases. The duration of the study was also too short to evaluate the effects of insulin administration on diabetic dogs. In the present study, only the serum IL-6 concentrations were lower after insulin treatment, which might have been due to persistent hyperglycemia mediating chronic inflammation during parts of each day, even after insulin treatment.
Furthermore, dogs with DKA and hyperosmolar hyperglycemic syndrome were excluded from our study. A recent study compared the adipokine concentrations of healthy dogs, dogs with DM, and dogs with DKA, regardless of concurrent diseases [26], and the results differed from those obtained in the present study, i.e., the IL-6 and leptin levels were below the detection limit in the majority of dogs, and the TNF-α and adiponectin concentrations did not differ significantly between the DKA, DM, and healthy dogs. The different results in these two studies might be explained by the exclusion of diabetic dogs with specific concurrent diseases and/or the methodology. Further studies will be required to elucidate the roles of adipokines during the pathogenesis of DM in dogs.
Overall, our results suggest that the circulating concentrations of visfatin and adiponectin might be dysregulated directly in diabetic dogs. However, the upregulation of leptin was probably a consequence of concurrent disorders, rather than being an effect of persistent hyperglycemia as a result of DM. Further studies are required to elucidate the association between hyperglycemia and adipokine dysregulation in diabetic dogs.
Figures and Tables
Fig. 1
Scatter plots of the serum leptin, visfatin, adiponectin, and resistin concentrations in healthy dogs (n = 20) and dogs with diabetes mellitus (n = 19). The horizontal bars indicate the median and range. *The median values were significantly (p < 0.05) different in healthy dogs and diabetic dogs (Mann-Whitney U test). DM: diabetes mellitus.
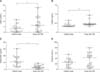
Fig. 2
Scatter plots of the serum leptin, visfatin, adiponectin, and resistin concentrations in healthy dogs (n = 20), diabetic dogs without concurrent diseases (n = 8), and diabetic dogs with concurrent diseases (n = 11). The horizontal bars indicate the median and range. *The median values were significantly (p < 0.05) different in healthy dogs and diabetic dogs (Mann-Whitney U test).
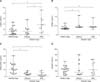
Acknowledgments
The authors thank all of the owners of the dogs included in the study. This research was supported by the Basic Science Research Program through the National Research Foundation of Korea (NRF), which was funded by the Ministry of Science, ICT, and Future Planning (2013R1A1A1011113).
References
1. Alexiadou K, Kokkinos A, Liatis S, Perrea D, Katsilambros N, Tentolouris N. Differences in plasma apelin and visfatin levels between patients with type 1 diabetes mellitus and healthy subjects and response after acute hyperglycemia and insulin administration. Hormones (Athens). 2012; 11:444–450.


2. Araya AV, Pavez V, Perez C, Gonzalez F, Columbo A, Aguirre A, Schiattino I, Aguillón JC. Ex vivo lipopolysaccharide (LPS)-induced TNF-α, IL-1β, IL-6 and PGE2 secretion in whole blood from Type 1 diabetes mellitus patients with or without aggressive periodontitis. Eur Cytokine Netw. 2003; 14:128–133.
3. Carrero JJ, Nakashima A, Qureshi AR, Lindholm B, Heimbürger O, Bárány P, Stenvinkel P. Protein-energy wasting modifies the association of ghrelin with inflammation, leptin, and mortality in hemodialysis patients. Kidney Int. 2011; 79:749–756.


4. Catchpole B, Ristic JM, Fleeman LM, Davison LJ. Canine diabetes mellitus: can old dogs teach us new tricks? Diabetologia. 2005; 48:1948–1956.


5. Chen MP, Chung FM, Chang DM, Tsai JC, Huang HF, Shin SJ, Lee YJ. Elevated plasma level of visfatin/pre-B cell colony-enhancing factor in patients with type 2 diabetes mellitus. J Clin Endocrinol Metab. 2006; 91:295–299.


6. Cho KD, Paek J, Kang JH, Chang D, Na KJ, Yang MP. Serum adipokine concentrations in dogs with naturally occurring pituitary-dependent hyperadrenocorticism. J Vet Intern Med. 2014; 28:429–436.


7. Choi SH, Hong ES, Lim S. Clinical implications of adipocytokines and newly emerging metabolic factors with relation to insulin resistance and cardiovascular health. Front Endocrinol (Lausanne). 2013; 4:97.


8. Dyck DJ, Heigenhauser GJ, Bruce CR. The role of adipokines as regulators of skeletal muscle fatty acid metabolism and insulin sensitivity. Acta Physiol (Oxf). 2006; 186:5–16.


9. El-Mesallamy HO, Kassem DH, El-Demerdash E, Amin AI. Vaspin and visfatin/Nampt are interesting interrelated adipokines playing a role in the pathogenesis of type 2 diabetes mellitus. Metabolism. 2011; 60:63–70.


10. DiMascio L, Voermans C, Uqoezwa M, Duncan A, Lu D, Wu J, Sankar U, Reya T. Identification of adiponectin as a novel hemopoietic stem cell growth factor. J Immunol. 2007; 178:3511–3520.


11. Fall T, Hamlin HH, Hedhammar A, Kämpe O, Egenvall A. Diabetes mellitus in a population of 180,000 insured dogs: incidence, survival, and breed distribution. J Vet Intern Med. 2007; 21:1209–1216.


12. Forsblom C, Thomas MC, Moran J, Saraheimo M, Thorn L, Wadén J, Gordin D, Frystyk J, Flyvbjerg A, Groop PH. FinnDiane Study Group. Serum adiponectin concentration is a positive predictor of all-cause and cardiovascular mortality in type 1 diabetes. J Intern Med. 2011; 270:346–355.


13. Fukuhara A, Matsuda M, Nishizawa M, Segawa K, Tanaka M, Kishimoto K, Matsuki Y, Murakami M, Ichisaka T, Murakami H, Watanabe E, Takagi T, Akiyoshi M, Ohtsubo T, Kihara S, Yamashita S, Makishima M, Funahashi T, Yamanaka S, Hiramatsu R, Matsuzawa Y, Shimomura I. Visfatin: a protein secreted by visceral fat that mimics the effects of insulin. Science. 2005; 307:426–430.


14. Guptill L, Glickman L, Glickman N. Time trends and risk factors for diabetes mellitus in dogs: analysis of veterinary medical data base records (1970-1999). Vet J. 2003; 165:240–247.


15. Haider DG, Pleiner J, Francesconi M, Wiesinger GF, Müller M, Wolzt M. Exercise training lowers plasma visfatin concentrations in patients with type 1 diabetes. J Clin Endocrinol Metab. 2006; 91:4702–4704.


16. Ishioka K, Hosoya K, Kitagawa H, Shibata H, Honjoh T, Kimura K, Saito M. Plasma leptin concentration in dogs: effects of body condition score, age, gender and breeds. Res Vet Sci. 2007; 82:11–15.


17. Karpavicius A, Dambrauskas Z, Sileikis A, Vitkus D, Strupas K. Value of adipokines in predicting the severity of acute pancreatitis: comprehensive review. World J Gastroenterol. 2012; 18:6620–6627.


18. Kitabchi AE, Stentz FB, Umpierrez GE. Diabetic ketoacidosis induces in vivo activation of human T-lymphocytes. Biochem Biophys Res Commun. 2004; 315:404–407.


19. Lee JM, Kim SR, Yoo SJ, Hong OK, Son HS, Chang SA. The relationship between adipokines, metabolic parameters and insulin resistance in patients with metabolic syndrome and type 2 diabetes. J Int Med Res. 2009; 37:1803–1812.


20. Li S, Shin HJ, Ding EL, van Dam RM. Adiponectin levels and risk of type 2 diabetes: a systematic review and meta-analysis. JAMA. 2009; 302:179–188.


21. López-Bermejo A, Chico-Juliá B, Fernández-Balsells M, Recasens M, Esteve E, Casamitjana R, Ricart W, Fernández-Real JM. Serum visfatin increases with progressive β-cell deterioration. Diabetes. 2006; 55:2871–2875.


22. Maggiore AD, Nelson RW, Dennis J, Johnson E, Kass PH. Efficacy of protamine zinc recombinant human insulin for controlling hyperglycemia in dogs with diabetes mellitus. J Vet Intern Med. 2012; 26:109–115.


23. Malyszko J, Malyszko JS, Kozminski P, Pawlak K, Mysliwiec M. Adipokines, linking adipocytes and vascular function in hemodialyzed patients, may also be possibly related to CD146, a novel adhesion molecule. Clin Appl Thromb Hemost. 2008; 14:338–345.


24. Mantzoros CS, Magkos F, Brinkoetter M, Sienkiewicz E, Dardeno TA, Kim SY, Hamnvik OPR, Koniaris A. Leptin in human physiology and pathophysiology. Am J Physiol Endocrinol Metab. 2011; 301:E567–E584.


25. Muse ED, Obici S, Bhanot S, Monia BP, McKay RA, Rajala MW, Scherer PE, Rossetti L. Role of resistin in diet-induced hepatic insulin resistance. J Clin Invest. 2004; 114:232–239.


26. O'Neill S, Drobatz K, Satyaraj E, Hess R. Evaluation of cytokines and hormones in dogs before and after treatment of diabetic ketoacidosis and in uncomplicated diabetes mellitus. Vet Immunol Immunopathol. 2012; 148:276–283.
27. Pickup JC, Mattock MB, Chusney GD, Burt D. NIDDM as a disease of the innate immune system: association of acute-phase reactants and interleukin-6 with metabolic syndrome X. Diabetologia. 1997; 40:1286–1292.


28. Rabe K, Lehrke M, Parhofer KG, Broedl UC. Adipokines and insulin resistance. Mol Med. 2008; 14:741–751.


29. Radin MJ, Sharkey LC, Holycross BJ. Adipokines: a review of biological and analytical principles and an update in dogs, cats, and horses. Vet Clin Pathol. 2009; 38:136–156.


30. Revollo JR, Körner A, Mills KF, Satoh A, Wang T, Garten A, Dasgupta B, Sasaki Y, Wolberger C, Townsend RR, Milbrandt J, Kiess W, Imai S. Nampt/PBEF/Visfatin regulates insulin secretion in β cells as a systemic NAD biosynthetic enzyme. Cell Metab. 2007; 6:363–375.


31. Rucinsky R, Cook A, Haley S, Nelson R, Zoran DL, Poundstone M;. AAHA diabetes management guidelines. J Am Anim Hosp Assoc. 2010; 46:215–224.
32. Saraheimo M, Forsblom C, Thorn L, Wadén J, Rosengård-Bärlund M, Heikkilä O, Hietala K, Gordin D, Frystyk J, Flyvbjerg A, Groop PH. FinnDiane Study Group. Serum adiponectin and progression of diabetic nephropathy in patients with type 1 diabetes. Diabetes Care. 2008; 31:1165–1169.


33. Schäffler A, Schölmerich J, Salzberger B. Adipose tissue as an immunological organ: Toll-like receptors, C1q/TNFs and CTRPs. Trends Immunol. 2007; 28:393–399.


34. Schwartz DR, Lazar MA. Human resistin: found in translation from mouse to man. Trends Endocrinol Metab. 2011; 22:259–265.


35. Uysal KT, Wiesbrock SM, Marino MW, Hotamisligil GS. Protection from obesity-induced insulin resistance in mice lacking TNF-α function. Nature. 1997; 389:610–614.


36. Verkest KR, Bjornvad CR. Understanding adiponectin in dogs and cats: a work in progress. Vet J. 2012; 193:4–5.


37. Verkest KR, Fleeman LM, Morton JM, Ishioka K, Rand JS. Compensation for obesity-induced insulin resistance in dogs: assessment of the effects of leptin, adiponectin, and glucagon-like peptide-1 using path analysis. Domest Anim Endocrinol. 2011; 41:24–34.

