Abstract
Chronic inflammation has been considered an important risk factor for development of prostate cancer. Toll-like receptors (TLRs) recognize microbial moieties or endogenous molecules and play an important role in the triggering and promotion of inflammation. In this study, we examined whether expression of TLR4 and TLR5 was associated with progression of prostate transformation in the transgenic adenocarcinoma of mouse prostate (TRAMP) model. The expression of TLR4 and TLR5 was evaluated by immunohistochemisty in formalin-fixed paraffin-embedded prostate tissue from wild-type (WT) and TRAMP mice. Normal prostate tissue from WT mice showed strong expression of TLR4 and TLR5. However, TLR4 expression in the prostate tissue from TRAMP mice gradually decreased as pathologic grade became more aggressive. TLR5 expression in the prostate tissue from TRAMP mice also decreased in low-grade prostate intraepithelial neoplasia (PIN), high-grade PIN and poorly differentiated adenocarcinoma. Overall, our results suggest that decreased expression of TLR4 and TLR5 may contribute to prostate tumorigenesis.
Prostate cancer is the most common malignancy and the second cause of cancer-related death among men in the United States. A wide range of genetic and environmental factors influence pathogenesis of prostate cancer [33]. Among the environmental factors, chronic inflammation has long been considered an important risk factor for the development of prostate cancer [25]. Chronic prostate inflammation can be induced by various sources including infection, urine reflux, dietary factors, or a combination of two or more of these factors [6]. Sexually transmitted agents such as Neisseria gonorrhea, Chlamydia trachomatitis, Trichomonas vaginalis and Escherichia coli have been shown to cause acute and chronic bacterial prostatitis [332]. In addition, uric acid, a chemical compound in the urine [29], and dietary 2-amino-1-methyl-6-phenylimidazo [4,5-b]pyridine (PhIP) treatment has been shown to induce chronic prostatitis.
Toll-like receptors (TLRs), a family of transmembrane proteins that recognize microbial moieties or endogenous molecules, play an important role in triggering and promoting inflammation. Stimulation of innate immune cells (e.g., dendritic cells and macrophages) by TLRs induces the release of inflammatory cytokines and chemokines and development of adaptive immune response manifested by T and B cell activation [1821]. To date, ten functional TLRs have been identified in humans. The ligands of these TLRs are bacterial lipoprotein (recognized by TLR1, 2, and 6), lipopolysaccharide (TLR4), flagellin (TLR5), viral double-stranded RNA (TLR3), single-stranded RNA (TLR7) and unmethylated CpG DNA (TLR9) of bacterial or viral genome [35]. After recognition of specific microbial moieties, TLRs initiate inflammatory signaling through intracellular pathways that lead to activation of nuclear factor-κB (NF-κB), mitogen-activated protein kinases (MAPKs), and interferon regulatory factors (IRFs) [35]. Activated inflammatory signaling results in host defense response to eliminate microbial invasion through secretion of cytokines, chemokines, or interferones [35]. Accordingly, TLRs play an important role in host defense against prostate infection. TLR2 mediated Mycoplasma hominis-induced IL-8 production in prostate epithelial cells [36], TLR5 induced early expression of proinflammatory genes against Escherichia coli infection, and deficiency in TLR5 was found to lead to overwhelming bacterial growth [1].
Although TLRs play a central role in host defense response against microbial infection, recent reports confirm these receptors as crucial mediators involved in tumor growth and progression [722]. Several epidemiological studies demonstrated a significant decrease in expression of TLR4, 5, 7 and 9 in prostate carcinoma compared to benign prostate hyperplasia [19]. However, Väisänen et al. [37] showed increased expression of TLR9 in prostatic carcinoma relative to benign prostate hyperplasia and high expression of TLR3, 4, and 9 in prostate carcinoma recurrence [12]. These controversial data may come from individual differences in genetic and environmental background, even within the same group. For example, microorganisms colonized in tissue, which may possibly lead to up-regulation of TLR expression, were not considered.
There are several mouse models to study carcinogenesis of prostate cancer. The transgenic adenocarcinoma mouse prostate (TRAMP) model is a widely used in vivo system [14]. In this model, the SV40 large and small T antigens are expressed under the androgen-dependent control of the rat probasin promoter in the prostatic epithelium [814]. This provides a convincing animal model system to study the progression of prostate cancer. TRAMP mice progressively develop intraepithelial neoplasia (PIN), adenocarcinoma and subsequent metastasis with similar pathology of human prostate cancer [20]. In this study, we evaluated the expression of TLR4 and TLR5 during prostate cancer progression in a TRAMP model with the same genetic and environmental background.
TRAMP mice, a well characterized transgenic mouse model of prostate cancer, are well described in the literature [14]. TRAMP mice with a C57BL/6 background and wild-type (WT) C57BL/6 mice were purchased from Jackson Laboratory (USA) and Koatech (Korea), respectively. Mice were housed in a temperature-controlled room with a 12-h light:dark cycle and maintained at a constant temperature of 24 ± 1℃ and humidity of 55 ± 10%. All animal studies were approved by the Institutional Animal Care and Use Committee in Seoul National University, and all associated regulations were strictly followed.
Age-matched WT C57BL/6 and TRAMP mice were sacrificed at 28 to 32 weeks of age. Urogenital tissues including the urinary bladder, seminal vesicle, and prostate were obtained, fixed in 10% formalin for 24 h, and processed in a standard alcoholxylene series. The tissues were then embedded in paraffin, after which 3 µm sections were prepared and stained with hematoxylin and eosin (H&E; Sigma, USA). Prostate samples were classified as normal, low-grade prostate intraepithelial neoplasia (PIN), high-grade PIN, adenocarcinoma, and phylloides-like cancer according to Gleason grades [12].
Paraffin sections were blocked with 3% hydrogen peroxide (H2O2) in phosphate-buffered saline (PBS) for 10 min to remove endogenous peroxidase, then blocked with 10% normal goat serum in PBS for 30 min. Next, the sections were incubated overnight in a solution containing diluted rabbit or mouse IgG isotype, anti-cytokeratin 8 (1:200 dilution; Covance, USA), anti-synaptophysin (1:200 dilution; Abcam, UK), anti-TLR4 (1:200 dilution; Imgenex, USA) or anti-TLR5 antibody (1:200 dilution; Imgenex) at room temperature and subsequently exposed to biotinylated goat anti-rabbit IgG or anti-mouse IgG and streptavidin peroxidase complex (Vector Laboratories, USA). These sections were then visualized with 3,3'-diaminobenzidine in 0.1 M Tris-HCl buffer and counterstained with methyl green (Sigma). Three fields of epithelial area from each view image at magnifications of 100 and 400× were selected at random, and the intensity was determined using the ImageJ software (ver. 1.45p; NIH, USA).
The differences among the mean values of the different groups were assessed, and all data are expressed as the mean ± SD. Statistical calculations consisted of Pearson's Chi-squared test and one-way ANOVA followed by Tukey's multiple comparison test for multi-group comparisons using GraphPad Prism (ver. 5.01; GraphPad Software, USA). P values < 0.05 were considered statistically significant.
We first determined the pathologic grade of prostate from WT and TRAMP mice as previously described [2026]. Normal prostate glands of WT C57BL/6 mice were lined by a monolayer of cuboidal to columnar epithelial cells with basally oriented nuclei (panel A in Fig. 1). However, the prostate glands of TRAMP mice consisted of foci with multilayers of atypical cells in the epithelium or the lumen of the duct (panels B-E in Fig. 1). Prostate glands showing large foci with two or three layers of atypical cells without luminal involvement were classified as low-grade intraepithelial neoplasia (PIN) and those showing foci with multi layers of atypical cells without luminal involvement were classified as high-grade PIN. In high-grade PIN, the prostate gland showed epithelial crowing and stratification, including variation of nuclear shape, chromatin condensation, and an increase of nuclear-to-cytoplasmic ratio (panel C in Fig. 1). Poorly differentiated adenocarcinoma (AC) was characterized by anaplastic sheets of cells containing pleomorphic cells with irregular nuclei (panel D in Fig. 1). In addition to pathologic grade of prostate, TRAMP mice developed phylloides-like cancer (PLC) in seminal vesicles. The neoplastic stromal cells of PLC emerged multicentrically immediately beneath the cuboidal to columnar epithelium (panel E in Fig. 1).
A recent consensus report on mouse models of human prostate cancer demonstrated that TRAMP mice have a high incidence of neuroendocrine phenotype arising in the prostate [17]; therefore, we measured the expression of cytokeratin 8 (marker of epithelial differentiation) and synaptophysin (marker of neuroendocrine differentiation) in the TRAMP prostate. Normal and TRAMP prostate epithelium from all pathologic grades showed strong expression of cytokeratin 8 (panels A-D in Fig. 2). However, synaptophysin was occasionally detected only in single cells located on the prostate epithelium of high-grade PIN (panel C in Fig. 3) and on normal prostate epithelium in poorly differentiated adenocarcinoma tissue (panel D in Fig. 3). These IHC data showed that prostate tissues from all pathologic grades examined showed epithelial phenotype.
We next evaluated the expression of TLR4 and TLR5 in prostate tissues from WT and TRAMP mice with various pathologic grades. TLR4 was strongly expressed in the normal prostate epithelia of WT mice (panels A and E in Fig. 4). However, the intensity of TLR4 immunostaining in the prostate tissue from TRAMP mice gradually decreased as pathologic grade became more aggressive (p < 0.001) (panels B-E in Fig. 4). Both lowand high-grade PIN tissues showed reduced the expression of TLR4, and TLR4 expression of AC was very weak (panels B-E in Fig. 4).
TLR5 was moderately expressed in normal prostate (panel A in Fig. 5), but decreased during progression of prostate transformation (p < 0.001). Low-grade PIN, high-grade PIN and adenocarcinoma from TRAMP mice showed reduced expression of TLR5 compared with normal prostate tissue, although there was no significant difference between low-grade PIN, high-grade PIN and AC (panels B-E in Fig. 5). A comparison of TLR4 and TLR5 expression by measuring the intensity of immunostaining using an image analyzer is shown in panel E in Fig. 4 and 5. Staining with IgG antibody was not detected in prostate epithelial cells (data not shown).
Recent reports showed that the stimulation of TLRs in cancer cells can inhibit or promote tumorigenesis dependently on receptors or tumor cell type. Triggering of TLR3 induced apoptosis in human pharynx carcinoma cells and head and neck cancer [2324], while TLR9 agonist inhibited tumor growth by sensitizing lung cancer cells to apoptosis [38]. In the case of prostate cancer, TLR7 agonist inhibited the growth and colony formation of TRAMP-C2 cells [15]. Conversely, TLR4 stimulation promoted the survival and proliferation of hepatocarcinoma cells [39], while TLR5 stimulation promoted the migration and invasion of salivary gland adenocarcinoma [27] and the proliferation of gastric cancer cells [34]. In addition, activation of TLRs in cancer cells induces production of variable molecules that have pro- or antitumor activity. TLR4 stimulation of lung cancer cells promoted immune escape by inducing secretion of immunosuppressive cytokines and resistance to TNF-related apoptosis [16], while high levels of TLR3, TLR4, and TLR9 in breast cancer were associated with higher probability of metastasis [13]. Conversely, LPS treatment induced production of chemokines such as CCL2, CCL5, CXCL8, and CXCL10 in prostate cancer cells [11] and elicited T cell-mediated antitumor immune response, resulting in tumor growth inhibition [2]. Moreover, TLR3 or TLR5 agonist-treated prostate cells induced NF-κB-dependent upregulation of inflammatory molecules with recruitment of inflammatory cells [9].
Our IHC results showed that the expression of TLR4 and TLR5 decreased as the tumor progressed in TRAMP prostate. This reduced expression of TLR4 and TLR5 in transformed prostate may provide tumor cells with one strategy to escape host immune attack because TLRs on tumor cells can induce antitumor immune response as mentioned above. Conversely, the loss of differentiation in aggressive cells during tumorigenesis might lead to reduced expression of TLR4 and TLR5.
In human prostate cancer cells, TLR4 promotes an immune escape, survival and progression of malignancy. LPS treatment enhances metastasis of the human prostate cancer cell line PC3 by promoting VEGF and TGFβ1 expression [28], while tumorderived peroxiredoxin 1 mediates tumor metastasis via TLR4, providing a mechanistic link between inflammation and TLR4 in prostate carcinogenesis [31]. However, in accordance with our results, two independent studies showed that the percentage of epithelial cells expressing TLR4 is gradually reduced as pathologic grade increases in human prostate tissues [10] and that stimulation of prostate cancer cell MAT-LU with LPS in vitro before inoculation produces significant inhibition of tumor growth in Copenhagen rats [2]. Although these discrepancies among studies could not be explained clearly, diversity of in vivo microenvironments, including stroma-derived factors and immune cells, might be an important factor to be explored.
The effects of TLR5 stimulation on prostate tumorigenesis have not been well studied. However, Galli et al. [9] showed that TLR5 stimulation of human prostate cancer cells triggered the production of inflammatory cytokines and chemokines, which mediate recruitment of inflammatory cells, while König et al. [19] showed decreased levels of TLR5 mRNA in human prostate cancer tissue compared with that of benign prostate hyperplasia. Although the prostate epithelial expression of TLR5 was not defined in the latter because they showed TLR5 mRNA expression in crude prostate tissues, this result is in the line with our IHC results. Regarding the possible inhibitory function of TLR5 in cancer cells, Rhee et al. [30] showed that blocking TLR5-mediated signaling in human colon cancer xenografts was associated with enhanced tumor growth, while activation of TLR5 in breast cancer cells inhibited cell proliferation [4].
A recent consensus report on mouse models of human prostate cancer demonstrated that TRAMP mice have a high incidence of neuroendocrine tumors arising in the prostate that are highly metastatic to lung, liver and other tissues [17]. However, the origin of neuroendocrine malignancy remains controversial. Kaplan-Lefko et al. [20] concluded that the poorly differentiated adenocarcinoma expressed neuroendocrine features as a consequence of a phenotypic switch as a function of cancer progression. In addition, Yuan et al. [40] suggested that prostate cancer cells undergo a transdifferentiation process to become neuroendocrine-like cells, which acquire the neuroendocrine phenotype and express neuroendocrine markers. However, Chiaverotti et al. [5] suggested that neuroendocrine carcinomas arise independently from atypical hyperplasias and may develop from bipotential progenitor cells at an early stage of prostate tumorigenesis by transplantation studies using TRAMP prostatic ducts. Our IHC data showed that normal prostate, low-grad PIN, high-grad PIN and poorly differentiated adenocarcinoma tissue expressed cytokeratin 8, but not synaptophysin, demonstrating epithelial origin of poorly differentiated adenocarcinoma cells. Because epithelial to neuroendocrine transdifferentiation is thought to be a stochastic event related to prostate cancer progression in TRAMP, the absence of synaptophysin expression on poorly differentiated adenocarcinoma cells may be explained by insufficient progression of prostate epithelial malignancy to show neuroendocrine phenotype. Indeed, we found that expression of another epithelial marker, E-cadherin, was very weak in poorly differentiated adenocarcinoma tissue (data not shown).
In our study, the expression of TLR4 and TLR5 in TRAMP prostate decreased as pathologic grade became more aggressive, which could have been due to loss of differentiation during tumor progression and may provide tumor cells with a strategy to escape host immune surveillance. These findings suggest that TLR4 and TLR5 may contribute to antitumor immune response.
Figures and Tables
Fig. 1
Pathologic grade of transgenic adenocarcinoma of mouse prostate (TRAMP). Prostate tissues from wild-type (WT) and TRAMP mice were fixed in 10% formalin for 24 h, then processed in a standard alcohol-xylene series. The tissues were subsequently embedded in paraffin, after which 3 µm sections were prepared and stained with hematoxylin and eosin. Images of normal prostate tissue from WT mice (A) and low-grade prostate intraepithelial neoplasia (PIN) (B), high-grade PIN (C), poorly differentiated adenocarcinoma (D) and phylloides-like cancer (E) from TRAMP mice. Magnification: 400× (A-E), 100× (inset).
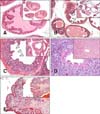
Fig. 2
Cytokeratin 8 expression in pathologic grades of TRAMP. Sections from Fig. 1 were incubated with anti-cytokeratin 8 antibody. Images of normal prostate tissue from WT mice (A) and low-grade PIN (B), high-grade PIN (C), poorly differentiated adenocarcinoma (D). Magnification: 400× (A-E), 100× (inset).
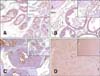
Fig. 3
Synaptophysin expression in pathologic grades of TRAMP prostate. Sections from Figure 1 were incubated with antisynaptophysin antibody. Images of normal prostate tissue from WT mice (A) and low-grade PIN (B), high-grade PIN (C), poorly differentiated adenocarcinoma (D). Magnification: 400× (A-D), 100× (inset).
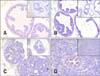
Fig. 4
TLR4 expression in pathologic grades of TRAMP prostate. Sections from Figure 1 were incubated with anti-TLR4 antibody. Images of normal prostate tissue from WT mice (A) and low-grade PIN (B), high-grade PIN (C), poorly differentiated adenocarcinoma (D). The intensity of TLR4 immunostaining in each pathologic grade is shown (E). Intensity of TLR4 immunostaining was measured in an average of three fields and is presented as the mean ± standard deviation (SD; *p < 0.05, **p < 0.01, and ***p < 0.001). Normal, normal prostate; Low PIN, low-grade PIN; High PIN, high-grade PIN; AC, poorly differentiated adenocarcinoma. Magnification: 400× (A-D), 100× (inset).
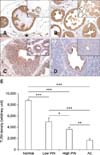
Fig. 5
TLR5 expression in pathologic grades of TRAMP prostate. Sections from Figure 1 were incubated with anti-TLR5 antibody. Images of normal prostate tissue from WT mice (A) and low-grade PIN (B), high-grade PIN (C), poorly differentiated adenocarcinoma (D). The intensity of TLR5 immunostaining in each pathologic grade is shown (E). Intensity of TLR5 immunostaining was measured in an average of three fields and is presented as mean ± SD (*p < 0.05 and **p < 0.01). (A-D) 400× magnification, (inset) 100× magnification.
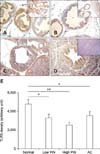
Acknowledgments
This work was supported by a program (grant no. 2010- 0002626) for Basic Research in Science and Engineering and by the World Class Institute (WCI) program (grant no. 2009- 002) of the National Research Foundation of Korea (NRF) funded by the Ministry of Education, Science and Technology of Korea (MEST). This study was also supported by the BK21 PLUS Program for Creative Veterinary Science Research and the Research Institute for Veterinary Science, Seoul National University, Korea.
References
1. Andersen-Nissen E, Hawn TR, Smith KD, Nachman A, Lampano AE, Uematsu S, Akira S, Aderem A. Cutting edge: Tlr5-/- mice are more susceptible to Escherichia coli urinary tract infection. J Immunol. 2007; 178:4717–4720.


2. Andreani V, Gatti G, Simonella L, Rivero V, Maccioni M. Activation of Toll-like receptor 4 on tumor cells in vitro inhibits subsequent tumor growth in vivo. Cancer Res. 2007; 67:10519–10527.


3. Cai T, Mazzoli S, Meacci F, Boddi V, Mondaini N, Malossini G, Bartoletti R. Epidemiological features and resistance pattern in uropathogens isolated from chronic bacterial prostatitis. J Microbiol. 2011; 49:448–454.


4. Cai Z, Sanchez A, Shi Z, Zhang T, Liu M, Zhang D. Activation of Toll-like receptor 5 on breast cancer cells by flagellin suppresses cell proliferation and tumor growth. Cancer Res. 2011; 71:2466–2475.


5. Chiaverotti T, Couto SS, Donjacour A, Mao JH, Nagase H, Cardiff RD, Cunha GR, Balmain A. Dissociation of epithelial and neuroendocrine carcinoma lineages in the transgenic adenocarcinoma of mouse prostate model of prostate cancer. Am J Pathol. 2008; 172:236–246.


6. De Marzo AM, Platz EA, Sutcliffe S, Xu J, Grönberg H, Drake CG, Nakai Y, Isaacs WB, Nelson WG. Inflammation in prostate carcinogenesis. Nat Rev Cancer. 2007; 7:256–269.


7. El-Omar EM, Ng MT, Hold GL. Polymorphisms in Toll-like receptor genes and risk of cancer. Oncogene. 2008; 27:244–252.


8. Foster BA, Gingrich JR, Kwon ED, Madias C, Greenberg NM. Characterization of prostatic epithelial cell lines derived from transgenic adenocarcinoma of the mouse prostate (TRAMP) model. Cancer Res. 1997; 57:3325–3330.
9. Galli R, Starace D, Busà R, Angelini DF, Paone A, De Cesaris P, Filippini A, Sette C, Battistini L, Ziparo E, Riccioli A. TLR stimulation of prostate tumor cells induces chemokine-mediated recruitment of specific immune cell types. J Immunol. 2010; 184:6658–6669.


10. Gatti G, Quintar AA, Andreani V, Nicola JP, Maldonado CA, Masini-Repiso AM, Rivero VE, Maccioni M. Expression of Toll-like receptor 4 in the prostate gland and its association with the severity of prostate cancer. Prostate. 2009; 69:1387–1397.


11. Gatti G, Rivero V, Motrich RD, Maccioni M. Prostate epithelial cells can act as early sensors of infection by upregulating TLR4 expression and proinflammatory mediators upon LPS stimulation. J Leukoc Biol. 2006; 79:989–998.


12. González-Reyes S, Fernández JM, González LO, Aguirre A, Suárez A, González JM, Escaff S, Vizoso FJ. Study of TLR3, TLR4, and TLR9 in prostate carcinomas and their association with biochemical recurrence. Cancer Immunol Immunother. 2011; 60:217–226.


13. González-Reyes S, Marín L, González L, González LO, del Casar JM, Lamelas ML, González-Quintana JM, Vizoso FJ. Study of TLR3, TLR4 and TLR9 in breast carcinomas and their association with metastasis. BMC Cancer. 2010; 10:665.


14. Greenberg NM, DeMayo F, Finegold MJ, Medina D, Tilley WD, Aspinall JO, Cunha GR, Donjacour AA, Matusik RJ, Rosen JM. Prostate cancer in a transgenic mouse. Proc Natl Acad Sci U S A. 1995; 92:3439–3443.


15. Han JH, Park SY, Kim JB, Cho SD, Kim B, Kim BY, Kang MJ, Kim DJ, Park JH, Park JH. TLR7 expression is decreased during tumour progression in transgenic adenocarcinoma of mouse prostate mice and its activation inhibits growth of prostate cancer cells. Am J Reprod Immunol. 2013; 70:317–326.


16. He W, Liu Q, Wang L, Chen W, Li N, Cao X. TLR4 signaling promotes immune escape of human lung cancer cells by inducing immunosuppressive cytokines and apoptosis resistance. Mol Immunol. 2007; 44:2850–2859.


17. Ittmann M, Huang J, Radaelli E, Martin P, Signoretti S, Sullivan R, Simons BW, Ward JM, Robinson BD, Chu GC, Loda M, Thomas G, Borowsky A, Cardiff RD. Animal models of human prostate cancer: the consensus report of the New York meeting of the mouse models of human cancers consortium prostate pathology committee. Cancer Res. 2013; 73:2718–2736.


18. Iwasaki A, Medzhitov R. Toll-like receptor control of the adaptive immune responses. Nat Immunol. 2004; 5:987–995.


19. König JE, Senge T, Allhoff EP, König W. Analysis of the inflammatory network in benign prostate hyperplasia and prostate cancer. Prostate. 2004; 58:121–129.


20. Kaplan-Lefko PJ, Chen TM, Ittmann MM, Barrios RJ, Ayala GE, Huss WJ, Maddison LA, Foster BA, Greenberg NM. Pathobiology of autochthonous prostate cancer in a preclinical transgenic mouse model. Prostate. 2003; 55:219–237.


21. Kawai T, Akira S. Toll-like receptors and their crosstalk with other innate receptors in infection and immunity. Immunity. 2011; 34:637–650.


22. Kim WY, Lee JW, Choi JJ, Choi CH, Kim TJ, Kim BG, Song SY, Bae DS. Increased expression of Toll-like receptor 5 during progression of cervical neoplasia. Int J Gynecol Cancer. 2008; 18:300–305.


23. Matijevic T, Marjanovic M, Pavelic J. Functionally active Toll-like receptor 3 on human primary and metastatic cancer cells. Scand J Immunol. 2009; 70:18–24.


24. Nomi N, Kodama S, Suzuki M. Toll-like receptor 3 signaling induces apoptosis in human head and neck cancer via survivin associated pathway. Oncol Rep. 2010; 24:225–231.


25. Omabe M, Ezeani M. Infection, inflammation and prostate carcinogenesis. Infect Genet Evol. 2011; 11:1195–1198.


26. Park JH, Walls JE, Galvez JJ, Kim M, Abate-Shen C, Shen MM, Cardiff RD. Prostatic intraepithelial neoplasia in genetically engineered mice. Am J Pathol. 2002; 161:727–735.


27. Park JH, Yoon HE, Kim DJ, Kim SA, Ahn SG, Yoon JH. Toll-like receptor 5 activation promotes migration and invasion of salivary gland adenocarcinoma. J Oral Pathol Med. 2011; 40:187–193.


28. Pei Z, Lin D, Song X, Li H, Yao H. TLR4 signaling promotes the expression of VEGF and TGFβ1 in human prostate epithelial PC3 cells induced by lipopolysaccharide. Cell Immunol. 2008; 254:20–27.


29. Persson BE, Ronquist G. Evidence for a mechanistic association between nonbacterial prostatitis and levels of urate and creatinine in expressed prostatic secretion. J Urol. 1996; 155:958–960.


30. Rhee SH, Im E, Pothoulakis C. Toll-like receptor 5 engagement modulates tumor development and growth in a mouse xenograft model of human colon cancer. Gastroenterology. 2008; 135:518–528.


31. Riddell JR, Bshara W, Moser MT, Spernyak JA, Foster BA, Gollnick SO. Peroxiredoxin 1 controls prostate cancer growth through Toll-like receptor 4-dependent regulation of tumor vasculature. Cancer Res. 2011; 71:1637–1646.


32. Sarma AV, McLaughlin JC, Wallner LP, Dunn RL, Cooney KA, Schottenfeld D, Montie JE, Wei JT. Sexual behavior, sexually transmitted diseases and prostatitis: the risk of prostate cancer in black men. J Urol. 2006; 176:1108–1113.


34. Song EJ, Kang MJ, Kim YS, Kim SM, Lee SE, Kim CH, Kim DJ, Park JH. Flagellin promotes the proliferation of gastric cancer cells via the Toll-like receptor 5. Int J Mol Med. 2011; 28:115–119.


36. Takeyama K, Mitsuzawa H, Shimizu T, Konishi M, Nishitani C, Sano H, Kunishima Y, Matsukawa M, Takahashi S, Shibata K, Tsukamoto T, Kuroki Y. Prostate cell lines secrete IL-8 in response to Mycoplasma hominis through Toll-like receptor 2-mediated mechanism. Prostate. 2006; 66:386–391.


37. Väisänen MR, Väisänen T, Jukkola-Vuorinen A, Vuopala KS, Desmond R, Selander KS, Vaarala MH. Expression of Toll-like receptor-9 is increased in poorly differentiated prostate tumors. Prostate. 2010; 70:817–824.


38. Wang H, Rayburn ER, Wang W, Kandimalla ER, Agrawal S, Zhang R. Chemotherapy and chemosensitization of nonsmall cell lung cancer with a novel immunomodulatory oligonucleotide targeting Toll-like receptor 9. Mol Cancer Ther. 2006; 5:1585–1592.

