Abstract
Increasing evidence suggests that cancer stem cells (CSCs) are responsible for tumor initiation and maintenance. Additionally, it is becoming apparent that cyclooxygenase (COX) signaling is associated with canine mammary tumor development. The goals of the present study were to investigate COX-2 expression patterns and their effect on CSC-mediated tumor initiation in primary canine mammary tissues and tumorsphere models using immunohistochemistry. Patterns of COX-2, CD44, octamer-binding transcription factor (Oct)-3/4, and epidermal growth factor receptor (EGFR) expression were examined in malignant mammary tumor (MMT) samples and analyzed in terms of clinicopathological characteristics. COX-2 and Oct-3/4 expression was higher in MMTs compared to other histological samples with heterogeneous patterns. In MMTs, COX-2 expression correlated with tumor malignancy features. Significant associations between COX-2, CD44, and EGFR were observed in low-differentiated MMTs. Comparative analysis showed that the levels of COX-2, CD44, and Oct-3/4 expression varied significantly among TSs of three histological grades. Enhanced COX-2 staining was consistently observed in TSs. Similar levels of staining intensity were found for CD44 and Oct-3/4, but EGFR expression was weak. Our findings indicate the potential role of COX-2 in CSC-mediated tumor initiation, and suggest that COX-2 inhibition may help treat canine mammary tumors by targeting CSCs.
Mammary carcinoma is one of the most important diseases leading to increased morbidity and mortality in female dogs [23]. Recent stem cell research in veterinary medicine suggests that mammary carcinoma may be driven by cancer stem cells (CSCs) [21926]. This subset of cancer cells has been identified in some types of canine tumors [41528] including mammary tumors. In canine mammary tumors (CMTs), the existence of CSCs has been confirmed by expression of several well-known molecular stem cell markers, CD44, CD24 [13], octamer-binding transcription factor(Oct)-3/4 [27], and aldehyde dehydrogenase (ALDH) 1 [14], that serve as key factors for cell differentiation and maintenance of pluripotency [910]. This subpopulation can also be isolated and enriched in tumorsphere (TS) models for CSC research [422].
To date, a series of cell-based markers and intrinsic signal pathways have been explored to characterize CSCs for the development of innovative cancer treatments by targeting CSCs. In the past few decades, the cyclooxygenase (COX)-prostaglandin E2 (PGE2) pathway has been widely studied and emerged as an important regulator of inflammation-induced or non-inflammatory carcinoma [8]. Aberrant COX-2 expression has also been observed in CMTs and is thought to be a negative prognostic factor [6923]. Recently, it was reported that COX-2-derived PGE2 protects embryonic stem cells from apoptosis [12] and is considered an important mediator in the stem cell network and stem cell-carcinoma niche [11]. However, few studies on the possible role of COX-2 in CSC-mediated tumor initiation for primary CMTs have been performed. Therefore, we conducted the present investigation to clarify the potential role of COX-2 in sustaining CSCs during tumor progression by measuring COX-2 expression and examining its correlation with CSC biomarkers (CD44, Oct-3/4, and epidermal growth factor receptor [EGFR]) in CMTs and CSC-enriched TSs.
CMT tissues were collected from dogs that underwent surgical resection at the Veterinary Teaching Hospital of China Agricultural University (China). Mammary tissues were histologically classified by pathologists according to previously described protocols [36]. The size, histological subtype, histological malignancy, Ki-67 proliferative index, and lymphatic invasion were assessed for each tumor. Cases of malignant mammary tumors (MMTs) were divided according to histological grade. Four normal mammary gland (NMG) tissue samples were included as control samples.
A total of 24 mammary tumor tissue samples from 79 malignant cases were aseptically collected and processed. Briefly, the tissues were minced into small pieces of about 1 mm, then transferred and digested for 4~16 h at 37℃ with 200 U/mL collagenase (Sigma-Aldrich, USA) and 100 U/mL hyaluronidase (Sigma-Aldrich) whilst being stirred. Digestion was terminated and the solution was centrifuged. The resulting cell pellets were suspended and passed through a 40-µm strainer. The single cells were then collected and resuspended for TS culture in serum-free Dulbecco's modified Eagle medium/Ham's F-12 medium (DMEM/F12) supplemented with B27 (Gibco-BRL, USA), epidermal growth factor (EGF; 20 ng/mL; PeproTech, USA), basic fibroblast growth factor (bFGF; 20 ng/mL; PeproTech), and 0.4% bovine serum albumin (BSA; Sigma-Aldrich). After 10~14 days of culturing, the spheres were pelleted and fixed in 10% neutral buffered formalin for 30 min before paraffin embedding.
De-paraffinized tissue and cell block sections were subjected to heat-induced or pepsin digestion antigen retrieval and incubation in 3% hydrogen peroxide. Next, the sections were incubated overnight with primary antibodies specific for COX-2 (diluted 1 : 100; Vector Laboratories, USA), CD44 (diluted 1 : 100; Santa Cruz Biotechnology, USA), POU5F1B transcription factor (Oct-3/4, diluted 1 : 80; Santa Cruz Biotechnology), EGFR (diluted 1 : 100; Zymed Laboratories, USA), and Ki-67 (diluted 1 : 150; BD Pharmingen, USA) at 4℃ using a two-step Polymer Detection System (GBI Labs, USA). Antibody binding was visualized with 3,3'-diaminobenzidine tetrahydrochloride and the sections were counterstained with Mayer's hematoxylin. For the negative controls, the primary antibody was replaced with phosphate-buffered saline (pH 7.2).
IHS was performed by estimating the percent of positive cells and labeling intensity. The percentages of positive cells were scored as 0 (0% positive cells), 1 (< 10% positive cells), 2 (10~50% positive cells), 3 (51~80% positive cells), or 4 (> 80% positive cells). Staining intensity was scored as 0 (negative), 1 (weak), 2 (moderate), or 3 (strong). Scores for the percentage of positive cells and staining intensity were multiplied to calculate an IHS value from 0 to 12. IHS values ≤ 6 were considered to represent low expression while values 7~12 were considered high. The Ki-67 proliferation index was determined based on the percentage of positive cells: 0 (negative), 1+ (≤ 10% of cells labeled), 2+ (11~50% of cells labeled), or 3+ (51~100% of cells labeled). Each section was examined using a microscope (Nikon, Japan) by two pathologists who were blind to the clinical characteristics and prognosis.
Statistical analyses were performed using SPSS software (ver. 16.0; SPSS, USA) and GraphPad Prism 5.0 (GraphPad Software, USA). Categorical data are expressed as frequencies and percentages. A chi-square test and Fisher's exact test were used to evaluate categorical variables. P values < 0.05 were considered significant.
Histological examination was performed for seven mammary hyperplasia (MH) samples, 79 MMTs including different histological subtypes, and four normal mammary gland (NMG) tissue samples as a control. Histological classification of the MMTs showed that these cases included three carcinoma in situ, 10 complex carcinoma, nine carcinosarcoma, 16 ductal carcinoma, one inflammatory carcinoma, 15 simple carcinoma, and 16 ductal carcinoma. Well-(WD), moderately (MD), and poorly differentiated (PD) tumors accounted for 17 (21.5%), 47 (59.5%), and 15 (20.0 %) of the MMTs, respectively (Table 1).
Among the MMTs, high COX-2 expression was observed in 36 cases (45.6% of the total cases), and low COX-2 expression was found in 25 cases (31.6%). Similarly, the high and low expression rates were 51.9% (41/79) and 35.4% (28/79) for CD44, 22.9% (18/79) and 15.2% (12/79) for Oct-3/4, and 49.4% (39/79) and 40.5% (32/79) for EGFR, respectively. All the unlisted cases were negative for each labeled protein. All the NMGs were negative or had low expression levels for these proteins, and expression varied in the MHs (Table 2).
COX-2 expression was observed in the cytoplasm, cell membrane, and nucleus of the neoplastic epithelial cells with weak to moderate immunoreactivity in some stromal cells. Highly aggressive tumor tissues and neoplastic cells had intense staining in a heterogeneous pattern compared to noninvasive tissues (panel A in Fig. 1). COX-2 expression was significantly higher in the MMTs than other types of specimens (p = 0.001). Oct-3/4 was widely detected in malignant neoplastic cells with strong and diffuse nuclear staining patterns (panel B in Fig. 1). Normal and hyperplastic tissues were mildly positive for Oct-3/4 while strong expression of this factor was observed predominantly at the invasive edge of malignant tissues (p = 0.016). CD44-specific staining was primarily found in the cell membrane of epithelial and myoepithelial cells (panel C in Fig. 1), but was absent from the apical epithelial surface, especially in normal and hyperplasic mammary tissue. CD44 was more extensively expressed in invasive components of the MMTs compared to NMGs although this difference was not statistically significant (p = 0.079). EGFR expression patterns were similar to those of CD44, and its membrane and cytoplasmic localization was consistently observed in epithelial cells of MMTs regardless of histological subtype (panel D in Fig. 1). Malignant tumors also had a higher positive ratio for EGFR than other types of specimens (p = 0.103; Table 2).
Elevated COX-2 expression was significantly associated with tumor size (p = 0.006), histological subtype (p = 0.017), histological grade (p = 0.032), lymphatic invasion (p = 0.033), and Ki-67 index (p = 0.022). Oct-3/4 expression strongly correlated with the Ki-67 index (p = 0.018). CD44 expression was statistically associated with lymphatic invasion (p = 0.005). EGFR expression was significantly related to histological subtype (p = 0.019) and Ki-67 index (p = 0.020). Significant associations between protein expression and selected variables are summarized in Table 1.
In moderately and poorly differentiated MMTs, COX-2 expression was strongly associated with CD44 (r = 0.402, p = 0.000; r = 0.810, p = 0.005) and EGFR (r = 0.367, p = 0.04; r = 0.700, p = 0.02) expression. In contrast, no statistical correlation was found between COX-2 and Oct-3/4 expression (Table 3).
Cell blocks from 24 CMTs with different histological grades (seven WD tumors including one simple carcinoma and six simple carcinomas, nine MD tumors including three ductal carcinomas and six solid carcinomas, and eight PD tumors consisting of four solid carcinomas, three complex carcinomas, and one carcinosarcomas) were analyzed to evaluate COX-2, CD44, Oct-3/4, and EGFR immunolabeling. Representative TSs derived from tubular carcinomas (WDs), tubulopapillary carcinomas (MDs), and solid carcinomas (PDs) were assessed. The TSs had inter-sphere heterogeneity and a compact morphology. Enhanced COX-2 expression was consistently observed in the cytoplasm with sporadic nuclear staining in three TSs. COX-2 expression was stronger in TSs derived from more malignant (more poorly differentiated) tumors. CD44 staining was enriched in cellular membranes. Nuclear localization of Oct-3/4 was extensive in solid carcinomagenerated spheres compared to the other two samples. EGFR was located in the cytoplasm with negative or weak immunoreactivity. Comparative analysis showed that there was a significant difference in COX-2 (PD 10.0 ± 2.1, MD 7.6 ± 1.9, WD 10.0 ± 2.1; p = 0.006), CD44 (PD 10.9 ± 1.9, MD 8.9 ± 2.5, WD 6.5 ± 1.4; p = 0.002), and Oct-3/4 (PD 9.4 ± 2.5, MD 6.0 ± 1.4, WD 5.5 ± 1.4; p = 0.001) expression among the three histological grades (p < 0.01). This was not observed for EGFR expression (PD 1.7 ± 1.4, MD 2.2 ± 2.1, WD 2.0 ± 1.4; p = 0.856). Staining specific for CD44 and Oct-3/4 had a similar level of intensity along with elevated COX-2 expression in the TSs (Fig. 2).
CSCs have the ability to self-renew and generate the diverse types of cells that form tumors. This makes CSCs a potential therapeutic target in human and veterinary oncology. Oncoprotein COX-2 is generally characterized as a pivotal regulator of growth and anti-apoptosis signals used by malignant tumor cells [724], and may also have an impact on CSCs [161826]. In the current study, the possible role of COX-2 in tumor initiation was explored by assessing the expression patterns of COX-2 and CSC-related markers.
COX-2 plays different roles in tumor initiation. According to our data, enhanced COX-2 expression and a heterogeneous pattern were commonly observed in pre-malignant and malignant tumor tissues, and were strongly associated with malignant features such as large tumor size, complex histological type, poor differentiation, and an aggressive phenotype as previously reported in the literature [5821]. Absent or low levels of COX-2 expression were found in normal and hyperplastic mammary tissues while expression was dramatically increased in tumor tissues. These results also imply that inducible COX-2 expression may promote canine mammary tumorigenesis. Moreover, differential stromal COX-2 staining in fibroblasts, endothelial, and infiltrating inflammatory cells may also suggest that stroma-derived COX-2 contributes to the acquired proliferative and invasive properties of tumor cells through tumor-stromal interaction during mammary tumorigenesis [1]. For cases of human breast cancer, it has been reported that proliferative tumors (COX-2+ or Ki-67+) possess more unfavorable characteristics and have a poorer prognosis than non-proliferative tumors (COX-2+ or Ki-67+) [20]. In our study, we found that MTs with rapid growth (Ki-67+) as well as moderate and poorly differentiated ones showed elevated COX-2 expression, which may suggest a dynamic switch of functional COX-2 during tumor proliferation and differentiation. It has been reported that down-regulated COX-2 expression suppresses the malignant phenotype, delays the onset of tumors formed by poorly differentiated cancer cells [24], and reverses the epithelial-to-mesenchymal transition [18].
In order to better understand the function of COX-2 in CSC-mediated tumor initiation, we evaluated the distribution of CSCs in mammary tissues by examining CD44, Oct-3/4, and EGFR expression patterns, and their respective associations with clinicopathological parameters. Aberrant expression of these factors was frequently observed in aggressive components of malignant tumors characterized by poor differentiation and rapid proliferation. Nevertheless, normal and hyperplastic tissues also had lower levels of expression. These findings indicate that CSCs maintain their malignant properties and can form a recurrent tumor that is more malignant. In contrast to COX-2, no significant correlation between CSC marker expression and poor prognostic variables was found. We therefore propose that these markers are not suitable independent prognostic factors. However, we further examined the co-expression of COX-2, CD44, Oct-3/4, and EGFR in MMTs and found significant associations among COX-2, CD44, and EGFR. These results show that COX-2 activation in CSCs may be CD44- or EGFR-dependent [1729]. Although we did not observe a relationship between COX-2 and Oct-3/4 in primary CMTs, it has been previously hypothesized that Oct-3/4 expression is associated with nuclear COX-2 activation [25] and COX-2high or Oct-3/4+ cells may acquire stem cell-like features in cases of human cancer [22].
The present study has provided new insight into the potential role of COX-2 in CSC-mediated tumor initiation by evaluating the expression patterns of COX-2 and CSC markers in TSs, which consist primarily of partially differentiated progenitor cells with few CSCs. The robust expression of COX-2 in heterogeneous patterns and at higher levels in more aggressive tumor-derived TSs suggests that COX-2 may contribute to the self-renewing ability and maintenance of CSCs. We compared COX-2, CD44, Oct-3/4, and EGFR expression in TSs according to tumor differentiation. The results confirmed that COX-2 expression accompanied enhanced CD44 and Oct-3/4 expression to a similar degree, and COX-2 up-regulation was associated with CSC enrichment in in vitro models. Therefore, it is reasonable to believe that COX-2 expression is necessary for CSC-mediated tumor initiation.
Taken together, our data highlight the potential role of COX-2 in CSC-mediated tumor initiation. These findings suggest that CSCs may be more sensitive to direct COX-2 inhibition than the general cell population. Further studies should be conducted to explore COX-2-dependant elimination of CSCs as well as the development of novel therapeutic approaches for CMTs.
Figures and Tables
Fig. 1
Immunostaining for COX-2, Oct-3/4, CD44, and EGFR in canine mammary carcinoma. (A) Strong cytoplasmic COX-2 expression in the invasive components of ductal carcinoma with sporadic nucleic labeling. (B) Homogeneous nucleic expression of Oct-3/4 in well-differentiated simple carcinoma. (C) Predominant membrane expression of CD44 in ductal carcinoma. (D) Strong membrane and cytoplasmic expression of EGFR in tubulopapillary carcinoma with apical labeling in luminal cells. Scale bar = 50 µm.
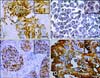
Fig. 2
Immunolabeling of COX-2, CD44, Oct-3/4, and EGFR in tumorspheres (TSs). TSs generated from primary tumor tissues were compactly aggregated with a regular or irregular appearance. Heterogeneous patterns of COX-2 expression in the cytoplasm and nucleus were observed in all three TSs. Intensity of COX-2 expression in the TSs was elevated in a manner corresponding to primary tumor malignancy (histological grade) along with similar intensities of membrane CD44 and nucleic Oct-3/4 staining. Additionally, EGFR was absent or weakly expressed in all samples. Asterisks indicate a significant difference in IHC scores between PD (n = 8) vs. MD (n = 9) vs. WD (n = 7) primary tumor-derived TSs (p < 0.01). Scale bar = 50 µm (TS) or 20 µm (EGFR).
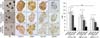
Table 1
Correlation of COX-2, CD44, Oct-4, and EGFR with selected clinical and pathological variables in MMTs (n = 79)*
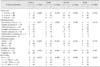
Table 2
COX-2, CD44, Oct-3/4, and EGFR expression correlated with different histological types*
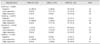
*p values indicating statistical differences between NMGs vs. MHs. vs. MMTs. p values < 0.05 were considered significant and were calculated using a chi-square test. COX: cyclooxygenase, EGFR: epidermal growth factor receptor, NMG: normal mammary gland, MH: mammary hyperplasia, MMT: malignant mammary tumor.
Acknowledgments
The authors thank Dr. Dong for technical assistance. This work was supported by research grant no.31372489 from the National Natural Science Foundation of China.
References
1. Arnoletti JP, Upson J, Babb JS, Bellacosa A, Watson JC. Differential stromal and epithelial localization of cyclooxygenase-2 (COX-2) during colorectal tumorigenesis. J Exp Clin Cancer Res. 2005; 24:279–287.
2. Borena BM, Bussche L, Burvenich C, Duchateau L, Van de Walle GR. Mammary stem cell research in veterinary science: an update. Stem Cells Dev. 2013; 22:1743–1751.


3. Clemente M, Pérez-Alenza MD, Illera JC, Peña L. Histological, immunohistological, and ultrastructural description of vasculogenic mimicry in canine mammary cancer. Vet Pathol. 2010; 47:265–274.


4. Cocola C, Anastasi P, Astigiano S, Piscitelli E, Pelucchi P, Vilardo L, Bertoli G, Beccaglia M, Veronesi MC, Sanzone S, Barbieri O, Reinbold RA, Luvoni GC, Zucchi I. Isolation of canine mammary cells with stem cell properties and tumour-initiating potential. Reprod Domest Anim. 2009; 44:Suppl 2. 214–217.


5. Doré M, Lanthier I, Sirois J. Cyclooxygenase-2 expression in canine mammary tumors. Vet Pathol. 2003; 40:207–212.


6. Goldschmidt M, Peña L, Rasotto R, Zappulli V. Classification and grading of canine mammary tumors. Vet Pathol. 2011; 48:117–131.


7. Greenhough A, Smartt HJ, Moore AE, Roberts HR, Williams AC, Paraskeva C, Kaidi A. The COX-2/PGE2 pathway: key roles in the hallmarks of cancer and adaptation to the tumour microenvironment. Carcinogenesis. 2009; 30:377–386.


8. Heller DA, Clifford CA, Goldschmidt MH, Holt DE, Shofer FS, Smith A, Sorenmo KU. Cyclooxygenase-2 expression is associated with histologic tumor type in canine mammary carcinoma. Vet Pathol. 2005; 42:776–780.


9. Hiraga T, Ito S, Nakamura H. Cancer stem-like cell marker CD44 promotes bone metastases by enhancing tumorigenicity, cell motility, and hyaluronan production. Cancer Res. 2013; 73:4112–4122.


10. Kumar SM, Liu S, Lu H, Zhang H, Zhang PJ, Gimotty PA, Guerra M, Guo W, Xu X. Acquired cancer stem cell phenotypes through Oct4-mediated dedifferentiation. Oncogene. 2012; 31:4898–4911.


11. Li HJ, Reinhardt F, Herschman HR, Weinberg RA. Cancer-stimulated mesenchymal stem cells create a carcinoma stem cell niche via prostaglandin E2 signaling. Cancer Discov. 2012; 2:840–855.


12. Liou JY, Ellent DP, Lee S, Goldsby J, Ko BS, Matijevic N, Huang JC, Wu KK. Cyclooxygenase-2-derived prostaglandin E2 protects mouse embryonic stem cells from apoptosis. Stem Cells. 2007; 25:1096–1103.


13. Magalhães GM, Terra EM, de Oliveira Vasconcelos R, de Barros Bandarra M, Moreira PRR, Rosolem MC, Alessi AC. Immunodetection of cells with a CD44+/CD24- phenotype in canine mammary neoplasms. BMC Vet Res. 2013; 9:205.


14. Michishita M, Akiyoshi R, Suemizu H, Nakagawa T, Sasaki N, Takemitsu H, Arai T, Takahashi K. Aldehyde dehydrogenase activity in cancer stem cells from canine mammary carcinoma cell lines. Vet J. 2012; 193:508–513.


15. Michishita M, Ezaki S, Ogihara K, Naya Y, Azakami D, Nakagawa T, Sasaki N, Arai T, Shida T, Takahashi K. Identification of tumor-initiating cells in a canine hepatocellular carcinoma cell line. Res Vet Sci. 2014; 96:315–322.


16. Moon CM, Kwon JH, Kim JS, Oh SH, Lee KJ, Park JJ, Hong SP, Cheon JH, Kim TI, Kim WH. Nonsteroidal anti-inflammatory drugs suppress cancer stem cells via inhibiting PTGS2 (cyclooxygenase 2) and NOTCH/HES1 and activating PPARG in colorectal cancer. Int J Cancer. 2014; 134:519–529.


17. Murphy JF, Lennon F, Steele C, Kelleher D, Fitzgerald D, Long AC. Engagement of CD44 modulates cyclooxygenase induction, VEGF generation, and proliferation in human vascular endothelial cells. FASEB J. 2005; 19:446–448.
18. Neil JR, Johnson KM, Nemenoff RA, Schiemann WP. Cox-2 inactivates Smad signaling and enhances EMT stimulated by TGF-β through a PGE2-dependent mechanisms. Carcinogenesis. 2008; 29:2227–2235.


19. Pang LY, Argyle D. Cancer stem cells and telomerase as potential biomarkers in veterinary oncology. Vet J. 2010; 185:15–22.


20. Park BW, Park S, Park HS, Koo JS, Yang WI, Lee JS, Hwang H, Kim SI, Lee KS. Cyclooxygenase-2 expression in proliferative Ki-67-positive breast cancers is associated with poor outcomes. Breast Cancer Res Treat. 2012; 133:741–751.


21. Queiroga FL, Pires I, Lobo L, Lopes CS. The role of Cox-2 expression in the prognosis of dogs with malignant mammary tumours. Res Vet Sci. 2010; 88:441–445.


22. Singh B, Cook KR, Vincent L, Hall CS, Martin C, Lucci A. Role of COX-2 in tumorospheres derived from a breast cancer cell line. J Surg Res. 2011; 168:e39–e49.


23. Sleeckx N, de Rooster H, Veldhuis Kroeze EJB, Van Ginneken C, Van Brantegem L. Canine mammary tumours, an overview. Reprod Domest Anim. 2011; 46:1112–1131.


24. Stasinopoulos I, Mori N, Bhujwalla ZM. The malignant phenotype of breast cancer cells is reduced by COX-2 silencing. Neoplasia. 2008; 10:1163–1169.


25. Thanan R, Murata M, Ma N, Hammam O, Wishahi M, El Leithy T, Hiraku Y, Oikawa S, Kawanishi S. Nuclear localization of COX-2 in relation to the expression of stemness markers in urinary bladder cancer. Mediators Inflamm. 2012; 2012:165879.


26. Trosko JE. Cancer stem cells and cancer nonstem cells: from adult stem cells or from reprogramming of differentiated somatic cells. Vet Pathol. 2009; 46:176–193.


27. Webster JD, Yuzbasiyan-Gurkan V, Trosko JE, Chang CC, Kiupel M. Expression of the embryonic transcription factor Oct4 in canine neoplasms: a potential marker for stem cell subpopulations in neoplasia. Vet Pathol. 2007; 44:893–900.

