Abstract
In the present study, the use of dogs with experimental autoimmune encephalomyelitis (EAE) as a disease model for necrotizing encephalitis (NE) was assessed. Twelve healthy dogs were included in this study. Canine forebrain tissues (8 g), including white and grey matter, were homogenized with 4 mL of phosphate-buffered saline for 5 min in an ice bath. The suspension was emulsified with the same volume of Freund's complete adjuvant containing 1 mg/mL of killed Mycobacterium tuberculosis H37Ra. Under sedation, each dog was injected subcutaneously with canine brain homogenate at four sites: two in the inguinal and two in the axillary regions. A second injection (booster) was administered to all the dogs using the same procedure 7 days after the first injection. Clinical assessment, magnetic resonance imaging, cerebrospinal fluid analyses, necropsies, and histopathological and immunohistochemical examinations were performed for the dogs with EAE. Out of the 12 animals, seven (58%) developed clinically manifest EAE at various times after immunization. Characteristics of canine EAE models were very similar to canine NE, suggesting that canine EAE can be a disease model for NE in dogs.
Necrotizing encephalitis (NE) is a common inflammatory disorder in veterinary medicine that affects the canine central nervous system (CNS) [30]. The pathogenesis of NE are poorly understood, and limited studies have been performed on dogs with this disease. Autoimmune, infectious, and neoplastic etiologies have been theorized, and an immune-mediated cause is considered most likely. Recent data have indicated that the inflammatory lesions associated with NE contain numerous CD3 antigen-positive T lymphocytes [2228]. These findings are suggestive of an organ-specific autoimmune disease with T cell-mediated delayed-type hypersensitivity [28]. Accordingly, T cell-mediated immune reactions may play a major role in NE development. In recent studies, anti-astrocyte autoantibodies were detected in the cerebrospinal fluid (CSF) of dogs with NE [1932]. However, autoantibodies against glial fibrillary acidic protein (GFAP) are not specific for this disease and are present with other CNS diseases [1932]. Therefore, it is still unclear whether astrocyte autoantibodies cause diseases such as NE.
The antemortem diagnosis of canine meningoencephalitis can be made using various neurodiagnostic approaches, including clinical and neurologic examinations, infectious disease testing, CSF analyses, and cross-sectional imaging analyses. However, histopathological diagnoses should be made based on necropsy findings to differentiate and characterize canine CNS inflammatory diseases. NE is characterized by extensive necrosis and the infiltration of inflammatory cells, including lymphocytes, plasmacytes, neutrophils, and monocytes or histiocytes. Parenchymal necrosis is located mainly in the cerebral cortex or leptomeninges in cases of necrotizing meningoencephalitis (NME) and primarily in the cerebral white matter in cases of necrotizing leukoencephalitis (NLE) [22].
Multiple sclerosis (MS) that affects humans is a disease similar to canine NE [10]. MS is considered a T lymphocyte-mediated autoimmune disease specific for myelin antigens in the CNS, and results in demyelination and axonal injury [27]. It has been hypothesized that MS is a T cell-mediated autoimmune disorder according to studies involving an animal model of the disease, experimental autoimmune encephalomyelitis (EAE) [7121427]. Since the initial experiments, various immunogens, adjuvants, and methods of immunization have been repeatedly evaluated to establish experimental animal models that can be used to study CNS inflammation and demyelination. These studies have shown that EAE can reproduce many of the clinical, neuropathological, and immunological characteristics of MS [711122327]. A previous immunologic study has shown that EAE is a T cell-mediated autoimmune disease that results in myelin and axonal damage through various mechanisms [7]. The major difference between EAE and MS is that the development of EAE requires an external immunization step with brain tissue antigens whereas MS arises due to spontaneous sensitization to an autoantigen. Despite this limitation, most current knowledge about the etiopathogeneses of brain inflammation has been gained from EAE research. Without this information, an understanding of MS pathogenesis and development of new treatments would not be possible.
As described earlier, the etiopathogenesis, therapeutic modalities, and diagnostic tests for NME and NLE can be explored with EAE, which is a single disease model of MS. However, studies have to be conducted on actual patients because there are no pertinent animal disease models. In addition, a recent genetic study suggested that canine NE could be a naturally occurring animal model for fulminant human MS [10]. Because EAE and MS have many similarities, we hypothesized that canine EAE is a disease model of NE. Since previous evaluation of this theory was performed several decades ago in dogs and had many limitations [1531], we conducted the current study to assess canine EAE as a disease model for NE using clinical, diagnostic imaging, and histopathological techniques.
Twelve healthy dogs (1~4 years old, weighing 4.5~13.1 kg, three females/nine males, nine beagles/one schnauzer/two mongrels) were used in this study. The dogs were monitored for at least 1 month before immunization. A complete blood count, serum biochemistry analyses, physical and neurological examinations, and radiography were performed for every animal. All experimental procedures and protocols were performed in accordance with guidelines that were approved by the Institutional Animal Care and Use Committee (IACUC) of Gyeongsang National University (Korea).
The brain tissues were obtained from six different dogs that were euthanized at Gyeongsang National University Animal Medical Center (Table 1). All donated forebrain tissues, including white and grey matter, were stored fresh in the refrigerator at 4℃ and used within 7 days. The tissues (8 g) were homogenized with 4 mL of phosphate-buffered saline (PBS) for 5 min in an ice bath. The suspension was emulsified with the same volume of Freund's complete adjuvant containing 1 mg/mL of killed Mycobacterium tuberculosis H37Ra (Sigma-Aldrich, USA). While under medetomidine sedation (Domitor, 0.5 mL/kg, intravenous; Orion Corporation, Finland), each dog was injected subcutaneously with canine brain homogenate (0.16~0.20 mL/kg for six animals and 0.21~0.25 mL/kg for six animals) at four sites with two in the inguinal and two in the axillary regions. All animals received a second injection (booster) using the same procedure 7 days after the first injection.
Each dog was evaluated daily for neurological abnormalities and their general condition after immunization. According to a previously described semi-quantitative scale [511], the clinical course during immunization was recorded daily by two trained and experienced observers. Successful EAE induction was based on the following criteria: 1) clear appearance of neurological signs after immunization, 2) positive lesions identified during brain magnetic resonance imaging (MRI) after immunization, and 3) nucleated cell pleocytosis identified by CSF analysis after immunization.
Following induction with propofol (Anepol, 6 mg/kg, intravenous; Hana Pharm, Korea), general anesthesia was maintained with 3% isoflurane inhalation (Ipran; Hana Pharm). The location, extent, and progress of brain inflammation were assessed with 0.4-Tesla MRI systems (APERTO; Hitachi Medical Corporation, Japan). MRI was performed according to the study schedule (Table 2). For each animal, T1-weighted (T1W), T2-weighted (T2W), and fluid-attenuated inversion recovery (FLAIR) MRI data sets were recorded in the sagittal and transverse planes. Contrast studies were performed for animals nos. 10 and 11 with intravenous injections of 0.3 mL gadodiamide/kg body weight (Omniscan; GE Healthcare, UK). Post-contrast T1W images were acquired 1 min after the injection.
CSF was collected by cerebellomedullary cistern puncture with a 22-gauge spinal needle under general anesthesia. CSF analyses were performed according to the study schedule (Table 2) including cytological, total nucleated cell count (cells/µL), total protein (mg/dL), and CSF albumin (mg/dL) evaluation for all samples. In addition, protein electrophoresis was performed for the first CSF sample collected for each dog.
Postmortem examination was conducted for dogs that died after successful induction of EAE. Gross findings were recorded, and the brain was then sectioned into 3-mm-thick slices that were compared to the MRI findings. Tissues containing the lesions were fixed in 10% neutral-buffered formalin, embedded in paraffin, and sectioned serially into 4-µm-thick slices. Hematoxylin and eosin (H&E) staining was performed to assess inflammation and other histological changes observed during microscopic examination.
To evaluate the infiltration and distribution of inflammatory cells, monoclonal mouse antibodies against macrophages (1 : 100; Santa Cruz Biotechnology, USA) and CD 79a (1 : 100; Dako, Japan) along with polyclonal rabbit antibodies against CD3 (1 : 250; Dako, Japan) and GFAP (1 : 800; Dako, Japan) were used. All slides were washed four times in PBS and then incubated with horseradish peroxidase-conjugated secondary antibodies (Dako REAL EnVision kit; Dako, Denmark) for 40 min. Antibody binding was visualized with 3,3'-diaminobenzidine tetrahydrochloride (Wako Pure Chemical Industries, Japan), and the sections were counterstained with hematoxylin.
To identify astrocytes as a possible target of the autoimmune reaction, double-labeling immunofluorescence was performed. A fluorescein isothiocyanate-conjugated sheep polyclonal antibody against canine immunoglobulin G (IgG, 1 : 250; Bethyl Laboratories, USA) and a rabbit polyclonal antibody against human GFAP (1 : 1000; Abcam, USA) were used as primary antibodies for staining the antigen-retrieved slides. After three washes with PBS, the sections were incubated with secondary antibody (Alexa Fluor 594-labeled goat anti-rabbit IgG; Life Technologies, USA) at room temperature for 1 h. The slides were then mounted with Vectashield medium containing 4',6-diamidino-2-phenylindole (DAPI; Vector Labs, USA). Colocalization studies were performed with a fluorescence microscope (DM6000B; Leica Microsystems, Germany).
Out of the 12 dogs studied, seven (58%) developed clinically manifest neurological disease at various times after immunization. The first clinical signs of EAE were observed within an average of 14.8 days (range, 10~24 days) after the first injection. These signs included changes in general condition such as decreased activity, hypothermia, and anorexia as well as neurological symptoms including paresis, mental changes, nystagmus, facial palsy, or seizure. Among the seven dogs with neurological symptoms, four (nos. 2, 3, 4, and 9) underwent an acute clinical course whereas three other dogs had chronic-progressive (no. 10), recovery (no. 5), or relapsing-remission (no. 11) courses. Among the seven dogs with induced EAE, survival times after immunization was 12~63 days (average, 25.6 days) except for nos. 5 and 11. Two dogs (nos. 5 and 11) survived after experiment periods without neurological signs.
The first MR images for the seven dogs with neurological symptoms were obtained within a few days after initial clinical symptom onset. All animals had asymmetrically distributed lesions in the prosencephalon. The frontal (4/7), parietal (4/7), and temporal (4/7) lobes were most commonly affected. All lesions were hyperintense on T2W and FLAIR images, but isointense on T1W images. The brain lesions were mainly located in the white and gray matter. A mass effect, such as ventricular compression, was observed in three dogs (nos. 3, 10, and 11) while falx cerebri shift was not present in any animal. For four dogs (nos. 4, 5, 10, and 11), periventricular hyperintensities were observed on FLAIR images.
Follow-up MRI examinations were performed for three dogs (nos. 5, 10, and 11). In dog no. 5, a diffuse lesion in the white matter of the cerebral hemisphere was not detectable 15 days after the original lesion was observed. In dog no. 10, diffuse white matter lesions were observed 17 days after EAE induction on T2W and FLAIR images that persisted progressively for 6 weeks (Fig. 1). The wider the distribution of the lesion, the more focal the cystic changes. Furthermore, mass effects were observed in the ipsilateral hemisphere with lesions. Peripheral contrast enhancement of the cerebral parenchyma was seen at 52 and 59 days after EAE induction on gadodiamide-enhanced T1W images. In addition, extra-transverse enhancement was observed beside the parenchymal cystic lesions. In dog no. 11, a diffuse lesion in the white matter progressively disappeared from 24 to 39 days. The lesion recurred more widely in a similar position at 94 days and then disappeared again after 22 days. In this dog, contrast enhancement was seen on gadodiamide-enhanced T1W images at 24 and 116 days.
The first CSF analysis was performed for four (nos. 2, 3, 5, and 10) of the seven dogs with EAE within a few days of the appearance of clinical signs after immunization (Table 3). Serial CSF analyses were completed for three dogs (no. 2, 5, and 10) except for one dog that died suddenly. In dog no. 2, the nucleated cell number decreased to 160 cells/µL 4 days after the first analysis, and pleocytosis only involved mononuclear cells. In dog no. 10, the nucleated cell number increased to 40 cells/µL 32 days after the first analysis, and pleocytosis involved only mononuclear cells. In dog no. 11, the nucleated cell number decreased to the normal range (2 cells/µL) and only consisted of mononuclear cells.
Necropsies and neuropathological examinations were performed for five dogs (nos. 2, 3, 4, 9, and 10) after death. Postmortem gross findings included predominant left hemisphere atrophy in dog no. 10 with chronic clinical progression. Cross-sectional gross findings included discoloration, cavitation, atrophy, and ventricular enlargement were similar to areas shown to be affected by MRI findings (Fig. 2). The selected lesions diagnosed by MRI or gross findings were examined histopathologically with H&E staining. In all five dogs, the brain lesions were commonly distributed in the cerebral white matter; however, the cerebral cortex was also affected in two dogs (nos. 3 and 9). In these animals, the inflammatory lesions, which were composed of numerous neutrophils, mainly existed in the leptomeningeal and perivascular regions, and mononuclear cells were found in the meningitis lesions. Four dogs (nos. 2, 3, 4, and 9) had moderate to severe inflammation with mild to moderate malacic changes (Fig. 3). In addition, neutrophilic infiltration was present in all of the canines. The clinical courses between initial disease onset and death of these dogs ranged from 10 to 21 days. The other dog (no. 10) exhibited concurrent severe malacic changes or cavitation and severe inflammation. This animal had perivascular cuffs and parenchymal infiltrations of mononuclear cells whereas neutrophils were not observed at all. Furthermore, edema and mononuclear cell infiltrations were found throughout the meninges in dog no. 10. The length of the clinical course between initial disease onset and death in this dog (no. 10) last for 49 days (from 14 to 63 days after EAE induction). Perivascular cuffs and parenchymal infiltrations of inflammatory cells were observed in all five dogs.
Immunohistochemical staining (Fig. 4) was used to determine the distribution of the inflammatory cells and identify the presence of astrocytosis. In all five dogs with EAE (nos. 2, 3, 4, 9, and 10), CD3-positive T lymphocytes were found predominantly in the malacic neuroparenchyma, perivascular cuffs, and meningeal lesions. Macrophages were densely located in the active inflammatory lesions, such as the perivascular cuffs and meningeal lesions, whereas few were present in the malacic regions or neuroparenchyma. CD79a-positive B lymphocytes were dense in the malacic neuroparenchyma of three dogs (nos. 3, 4, and 10) while the population of CD79a-positive B lymphocytes in the malacic neuroparenchyma of two dogs (nos. 2 and 9) were relatively sparse. In cerebral sections labeled for GFAP, numerous sites of hypertrophic fibrillosis and astrocytosis were observed within and around the inflammatory lesions in all five dogs.
Immunofluorescence labeling was used to determine the distribution of plasma cells and assess the possibility of astrocytes as targets for autoimmune reactions. In the lesions of three dogs (nos. 2, 3, and 10), the IgG-positive plasma cells and GFAP-positive astrocytes tended to co-localize (Fig. 5) whereas IgG-positive plasma cells were not found in the other dogs. The cytoplasm of some astrocytes was positive for IgG and GFAP (yellow) in one dog (no. 10) with chronic-progressive EAE. These double-positive astrocytes were observed in the inflammatory lesions with necrosis and astrogliosis in this dog.
EAE is an inflammatory demyelinating disease of the CNS that is induced in laboratory animals by an autoimmune response against the myelin sheath. Researchers have used various immunogens including tissue homogenates of spinal cord or brain, myelin extracts, and proteins such as myelin basic protein, myelin proteolipid protein, and myelin oligodendrocyte glycoprotein [16182124]. However, encephalitogenic T-cell responses have been induced by myelin protein and other antigens, including astrocyte and neuron antigens [14], GFAP, transaldolase, Ma [23], and amyloid precursor protein [9]. The fact that idiopathic CNS inflammatory diseases, such as NE, are astrocyte-targeting autoimmune disorders has been supported by CSF, serologic, and histopathological analyses [1932]. In order to investigate the potential of an animal model for studying these diseases, we therefore inoculated dogs with brain tissue homogenates as immunogens that included various proteins.
The total incidence of EAE under the experimental conditions of this study was 58.0% (7 of 12 dogs), which was slightly lower than the rate (63.6%, 35 of 55 dogs) that has been reported for the same species [31]. However, the dogs were given repeated subcutaneous injections for 12 months at intervals of 2 to 5 weeks until neurological symptoms occurred in the previous study while neurologic signs were observed after only two injections at a set interval in the present investigation.
In the first CSF analysis, total protein and albumin levels were increased in three dogs (nos. 2, 3, and 10). Because albumin is solely of serum origin, increased CSF albumin concentration is considered an indicator of blood-brain barrier (BBB) damage [6]. Quantitative analyses of CSF proteins performed by agarose gel electrophoresis enable calculations of the albumin quotient (AQ), which has been proposed as a more significant measure than albumin alone [325]. The AQ, which was measured for only two out of four dogs in this study, was increased for both animals.
Protein distribution can be characterized using electrophoresis, and abnormal electrophoresis patterns of CSF proteins have been reported to be useful for confirming the presence of inflammatory, neoplastic, and degenerative disorders [2526]. In this study, an increased gamma globulin ratio and elevated albumin levels were observed in the CSF of all dogs with EAE. In addition, patterns of the CSF albumin and gamma globulin ratio increases were similar to that observed in cases of canine autoimmune encephalitis [2].
It is unclear whether pleocytosis associated with meningoencephalitis is due to an influx of systemic inflammatory cells or regional production by the CNS macrophage phagocytic system [13]. In previous reports, mononuclear-cell pleocytosis was most commonly found in dogs with NE although mixed-cell pleocytosis was also observed [16]. Mild to moderate mixed-cell pleocytosis may result from necrosis or inflammation due to various diseases such as spinal cord and cerebral trauma, disc disease, hemorrhagic myelomalacia, ischemia, or infarction. In the present study, the number of neutrophils and mononucleated cells was increased in the first CSF analysis of the four dogs with EAE. Interestingly, the pattern of pleocytosis changed from mixed-cell to mononuclear pleocytosis at the time of the second CSF analysis. This difference suggested that neutrophils play an important role in the acute phase of EAE in dogs. The absence of neutrophils in the CSF during diagnosis of diseases, such as MS or NE, may indicate that the acute phase of autoimmune inflammation has passed. In addition, this finding was reflected in the histological test results. In dogs with EAE, the perivascular or parenchymal infiltration of neutrophils was predominantly observed in the four animals with the acute form of the disease rather than in the dog with chronic progression. Therefore, neutrophils might play a role in the early or acute stage of the autoimmune process for canine EAE. However, further studies are warranted since a serial analysis of CSF was performed for only three dogs in this investigation.
There have been reports that small numbers of neutrophils can be observed during histopathological examination of dogs with NE with a predominant infiltration of mononuclear cells [29]. Although mononuclear cells predominated in most cases of the EAE models, neutrophils have been shown to infiltrate the brain in cases of hyperacute EAE [17]. In the current study, neutrophil infiltration was evident in the four dogs with acute EAE while the dog with chronic progression had only mononuclear cell infiltration. These histopathological findings agreed with the CSF test results and indicated the significant role of neutrophils in the acute or early stage of canine EAE. In dogs with suspected cases of NE, the presence of neutrophils in CSF and brain tissue may be a useful indicator of the acute disease stage. Nevertheless, a pathological examination of chronic progressive EAE was performed for only one dog in this investigation, and further studies are needed.
Conventional MRI techniques, such as T2W and gadolinium-enhanced T1W sequences, are commonly used for the diagnosis and treatment evaluation of MS lesions [20]. Furthermore, recent study has demonstrated the clinical usefulness of MRI and FLAIR for patients with MS [20]. MRI is also a powerful diagnostic tool for treating NE in veterinary medicine [30]. However, studies on lesion assessment with MRI are limited in rodents and marmoset monkeys, which are commonly used as disease models [8], and FLAIR imaging data are scarce. Moreover, obtaining serial clinical data with CSF or imaging analyses is difficult in these models and has thus been rarely reported [4]. In the present study, EAE was induced in dogs and various imaging techniques were used to collect information including T2W, gadolinium-enhanced T1W, and FLAIR data, notably for the serial assessment of disease progression. Therefore, results of the present study suggested that serial clinical assessment in therapeutic trials and MRI, including FLAIR studies, is possible using our canine EAE model.
Based on the MRI and histopathological examination findings, the brain lesions of dogs with EAE were mainly characterized by apparent parenchymal necrosis, perivascular cuffs, and parenchymal infiltration of inflammatory cells including lymphocytes, macrophages, neutrophils, and plasma cells. In addition, the lesions were predominately observed in the cerebral white matter and/or cortex. These features were very similar to those of NE [2229]. Immunohistochemical staining to observed the distribution of inflammatory cells revealed that CD3-positive T cells and macrophages were the most prominent inflammatory cells in dogs with EAE, which was comparable to NE. These findings suggested an etiopathogenesis of T cell-mediated delayed-type hypersensitivity reaction in dogs with EAE similar to the etiopathogenesis of NE [29]. Thus, the present study suggested that the canine EAE model had similar pathophysiological mechanisms as NE. Consequently, the canine EAE model might be valuable for studying NE in dogs.
An autoimmune pathogenesis has been suggested for NE based on the presence of anti-astrocyte autoantibodies in the CSF and IgG-positive astrocytes in the brain [192232]. In the current study, IgG in GFAP-positive astrocytes was observed in brain lesions of the dog with chronic EAE (no. 10); this was not seen in the four dogs with an acute fulminant clinical course. In addition, some astrocytes have been reported positive for both IgG and GFAP in dogs with NME and NLE but not with GME [22], suggesting that astrocytes are the target of the autoimmune response. However, double-labeling immunofluorescence did not reveal whether these autoreactive activities against GFAP were a primary cause or a secondary outcome of the immunization process. Only one dog (no. 10) obviously exhibited pathological and immunohistochemical features similar to NE which was reported previously [22]. Further study should be included a large cohort of chronic progression EAE models such as dog no. 10 for proving EAE models in this study is considerably similar to canine NE.
This was a pilot study for establishing canine EAE models. Thus, there were some limitations that are worth noting. First, whole brain tissues containing various types of proteins were used to induce EAE. Moreover, we used brain samples from six different donors for immunization. To establish immunization protocols for further study, the donor sample should come from one source. In addition, other antigens, including neurons, axons, myelin proteins, transaldolase, and amyloid precursor protein, should be considered as possible targets of autoimmunity in addition to astrocytes. Moreover, further study on EAE that is induced by a specific protein is needed to establish additional disease models for both NE and MS.
In conclusion, EAE was induced in dogs. The clinical features, CSF, MRI data, and neuropathological characteristics of the animals were evaluated. Based on these examinations, canine EAE appeared to be very similar to NE. Therefore, canine EAE appears to be a viable disease model for NE in dogs.
Acknowledgments
This research was supported by the Basic Science Research Program through the National Research Foundation of Korea (NRF) funded by the Ministry of Education, Science and Technology (2011-0008358).
References
1. Adamo PF, Adams WM, Steinberg H. Granulomatous meningoencephalomyelitis in dogs. Compend Contin Educ Vet. 2007; 29:678–690. PMID: 18210978.
2. Behr S, Trumel C, Cauzinille L, Palenché F, Braun JP. High resolution protein electrophoresis of 100 paired canine cerebrospinal fluid and serum. J Vet Intern Med. 2006; 20:657–662. PMID: 16734104.


3. Bichsel P, Vandevelde M, Vandevelde E, Affolter U, Pfister H. Immunoelectrophoretic determination of albumin and IgG in serum and cerebrospinal fluid in dogs with neurological diseases. Res Vet Sci. 1984; 37:101–107. PMID: 6473908.


4. Boretius S, Schmelting B, Watanabe T, Merkler D, Tammer R, Czéh B, Michaelis T, Frahm J, Fuchs E. Monitoring of EAE onset and progression in the common marmoset monkey by sequential high-resolution 3D MRI. NMR Biomed. 2006; 19:41–49. PMID: 16408325.


5. Brok HP, Uccelli A, Kerlero de Rosbo N, Bontrop RE, Roccatagliata L, de Groot NG, Capello E, Laman JD, Nicolay K, Mancardi GL, Ben-Nun A, 't Hart BA. Myelin/oligodendrocyte glycoprotein-induced autoimmune encephalomyelitis in common marmosets: the encephalitogenic T cell epitope pMOG24-36 is presented by a monomorphic MHC class II molecule. J Immunol. 2000; 165:1093–1101. PMID: 10878388.


6. Chrisman CL. Cerebrospinal fluid analysis. Vet Clin North Am Small Anim Pract. 1992; 22:781–810. PMID: 1641918.


7. Constantinescu CS, Farooqi N, O'Brien K, Gran B. Experimental autoimmune encephalomyelitis (EAE) as a model for multiple sclerosis (MS). Br J Pharmacol. 2011; 164:1079–1106. PMID: 21371012.


8. Dousset V, Ballarino L, Delalande C, Coussemacq M, Canioni P, Petry KG, Caillé JM. Comparison of ultrasmall particles of iron oxide (USPIO)-enhanced T2-weighted, conventional T2-weighted, and gadolinium-enhanced T1-weighted MR images in rats with experimental autoimmune encephalomyelitis. AJNR Am J Neuroradiol. 1999; 20:223–227. PMID: 10094342.
9. Furlan R, Brambilla E, Sanvito F, Roccatagliata L, Olivieri S, Bergami A, Pluchino S, Uccelli A, Comi G, Martino G. Vaccination with amyloid-β peptide induces autoimmune encephalomyelitis in C57/BL6 mice. Brain. 2003; 126:285–291. PMID: 12538398.
10. Greer KA, Wong AK, Liu H, Famula TR, Pedersen NC, Ruhe A, Wallace M, Neff MW. Necrotizing meningoencephalitis of Pug dogs associates with dog leukocyte antigen class II and resembles acute variant forms of multiple sclerosis. Tissue Antigens. 2010; 76:110–118. PMID: 20403140.


11. Hart BA, Bauer J, Muller HJ, Melchers B, Nicolay K, Brok H, Bontrop RE, Lassmann H, Massacesi L. Histopathological characterization of magnetic resonance imaging-detectable brain white matter lesions in a primate model of multiple sclerosis: a correlative study in the experimental autoimmune encephalomyelitis model in common marmosets (Callithrix jacchus). Am J Pathol. 1998; 153:649–663. PMID: 9708823.
12. Hohlfeld R, Wekerle H. Immunological update on multiple sclerosis. Curr Opin Neurol. 2001; 14:299–304. PMID: 11371751.


13. Kipar A, Baumgärtner W, Vogl C, Gaedke K, Wellman M. Immunohistochemical characterization of inflammatory cells in brains of dogs with granulomatous meningoencephalitis. Vet Pathol. 1998; 35:43–52. PMID: 9545134.


14. Kojima K, Berger T, Lassmann H, Hinze-Selch D, Zhang Y, Gehrmann J, Reske K, Wekerle H, Linington C. Experimental autoimmune panencephalitis and uveoretinitis transferred to the Lewis rat by T lymphocytes specific for the S100β molecule, a calcium binding protein of astroglia. J Exp Med. 1994; 180:817–829. PMID: 7520474.
15. Kuharik MA, Edwards MK, Farlow MR, Becker GJ, Azzarelli B, Klatte EC, Augustyn GT, Dreesen RG. Gd-enhanced MR imaging of acute and chronic experimental demyelinating lesions. AJNR Am J Neuroradiol. 1988; 9:643–648. PMID: 3135711.
16. Lassmann H, Kitz K, Wisniewski HM. Structural variability of demyelinating lesions in different models of subacute and chronic experimental allergic encephalomyelitis. Acta Neuropathol. 1980; 51:191–201. PMID: 7445973.


17. Levine S. Hyperacute, neutrophilic, and localized forms of experimental allergic encephalomyelitis: a review. Acta Neuropathol. 1974; 28:179–189. PMID: 4139872.


18. Levine S, Wenk EJ. Studies on the mechanism of altered susceptibility to experimental allergic encephalomyelitis. Am J Pathol. 1961; 39:419–441. PMID: 13761512.
19. Matsuki N, Fujiwara K, Tamahara S, Uchida K, Matsunaga S, Nakayama H, Doi K, Ogawa H, Ono K. Prevalence of autoantibody in cerebrospinal fluids from dogs with various CNS diseases. J Vet Med Sci. 2004; 66:295–297. PMID: 15107560.


20. McDonald WI, Compston A, Edan G, Goodkin D, Hartung HP, Lublin FD, McFarland HF, Paty DW, Polman CH, Reingold SC, Sandberg-Wollheim M, Sibley W, Thompson A, van den Noort S, Weinshenker BY, Wolinsky JS. Recommended diagnostic criteria for multiple sclerosis: guidelines from the International Panel on the diagnosis of multiple sclerosis. Ann Neurol. 2001; 50:121–127. PMID: 11456302.


21. McRae BL, Miller SD. Fine specificity of CD4+ T cell responses to the dominant encephalitogenic PLP 139-151 peptide in SJL/J mice. Neurochem Res. 1994; 19:997–1004. PMID: 7528357.
22. Park ES, Uchida K, Nakayama H. Comprehensive immunohistochemical studies on canine necrotizing meningoencephalitis (NME), necrotizing leukoencephalitis (NLE), and granulomatous meningoencephalomyelitis (GME). Vet Pathol. 2012; 49:682–692. PMID: 22262353.


23. Pellkofer H, Schubart AS, Höftberger R, Schutze N, Pagany M, Schüller M, Lassmann H, Hohlfeld R, Voltz R, Linington C. Modelling paraneoplastic CNS disease: T-cells specific for the onconeuronal antigen PNMA1 mediate autoimmune encephalomyelitis in the rat. Brain. 2004; 127:1822–1830. PMID: 15201193.


24. Sakai K, Zamvil SS, Mitchell DJ, Lim M, Rothbard JB, Steinman L. Characterization of a major encephalitogenic T cell epitope in SJL/J mice with synthetic oligopeptides of myelin basic protein. J Neuroimmunol. 1988; 19:21–32. PMID: 2456304.


25. Sorjonen DC. Total protein, albumin quota, and electrophoretic patterns in cerebrospinal fluid of dogs with central nervous system disorders. Am J Vet Res. 1987; 48:301–305. PMID: 3826872.
26. Sorjonen DC, Cox NR, Swango LJ. Electrophoretic determination of albumin and gamma globulin concentrations in the cerebrospinal fluid of dogs with encephalomyelitis attributable to canine distemper virus infection: 13 cases (1980-1987). J Am Vet Med Assoc. 1989; 195:977–980. PMID: 2477352.
27. Sospedra M, Martin R. Immunology of multiple sclerosis. Annu Rev Immunol. 2005; 23:683–747. PMID: 15771584.


28. Spitzbarth I, Schenk HC, Tipold A, Beineke A. Immunohistochemical characterization of inflammatory and glial responses in a case of necrotizing leucoencephalitis in a French bulldog. J Comp Pathol. 2010; 142:235–241. PMID: 19815229.


29. Suzuki M, Uchida K, Morozumi M, Hasegawa T, Yanai T, Nakayama H, Tateyama S. A comparative pathology study on canine necrotizing meningoencephalitis and granulomatous meningoencephalomyelitis. J Vet Med Sci. 2003; 65:1233–1239. PMID: 14665754.
30. Talarico LR, Schatzberg SJ. Idiopathic granulomatous and necrotising inflammatory disorders of the canine central nervous system: a review and future perspectives. J Small Anim Pract. 2010; 51:138–149. PMID: 19814766.


31. Thomas L, Paterson PY, Smithwick B. Acute disseminated encephalomyelitis following immunization with homologous brain extracts, I. Studies on the role of a circulating antibody in the production of the condition in dogs. J Exp Med. 1950; 92:133–152. PMID: 15428583.
32. Toda Y, Matsuki N, Shibuya M, Fujioka I, Tamahara S, Ono K. Glial fibrillary acidic protein (GFAP) and anti-GFAP autoantibody in canine necrotising meningoencephalitis. Vet Rec. 2007; 161:261–264. PMID: 17720962.


Fig. 1
Transverse T1W, T2W, and FLAIR images of dog no. 10 that were obtained on 17, 36, 52, and 59 days after immunization starting with the first MRI-detectable lesions appearing on day 17. Brain lesions in the left hemisphere progressively developed. Peripheral contrast enhancement (arrow) was seen at 52 and 59 days on gadodiamide (Gd)-enhanced T1W images. NE: not evaluated.
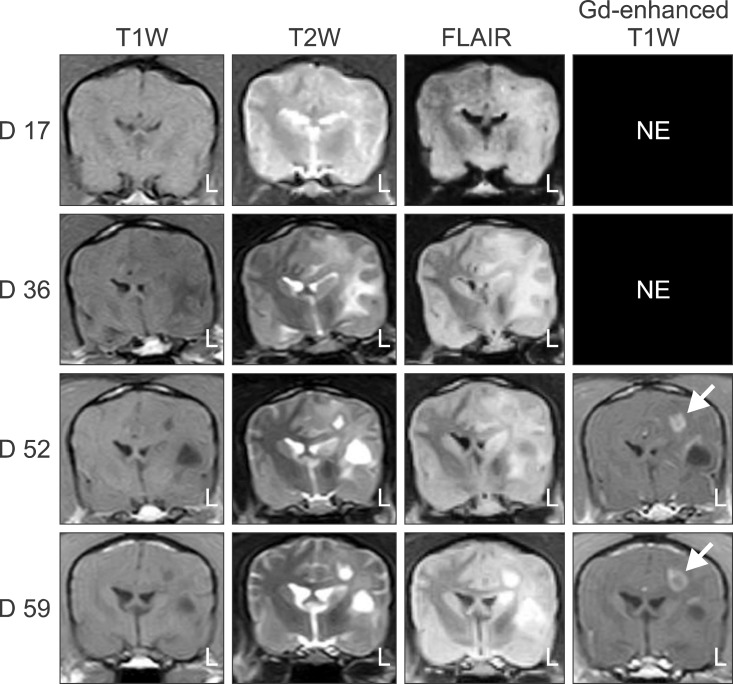
Fig. 2
MRI and cross-sectional gross findings for five dogs (nos. 2, 3, 4, 9, and 10) with EAE. Hyperintense lesions (white arrows) were found on T2W and FLAIR sequences for all dogs. In similar regions, macroscopic examination revealed discoloration and swelling of the cerebral white matter (white arrowheads) in all five animals. Note also the ventricular enlargement in two dogs (nos. 4 and 9) along with focal necrosis and atrophy of the cerebral hemisphere (black arrowhead) in one dog (no. 10).
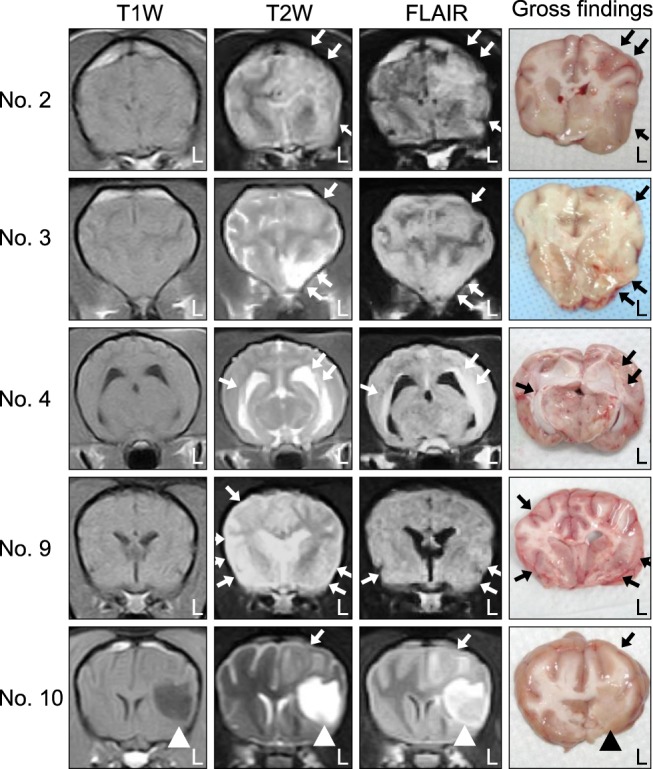
Fig. 3
Histopathologic examination findings of five dogs with EAE. (A) Cerebrum of dog no. 2. Perivascular cuffing, which is caused by neutrophils and mononuclear cells, was present in the underlying white matter. (B) Cerebrum of dog no. 3. Perivascular cuffing, containing neutrophils and mononuclear cells, in the underlying white matter. (C) Cerebrum of dog no. 4. Vasculitis, perivascular cuffs, and infiltration of inflammatory cells (neutrophils and mononuclear cells). (D) Cerebrum of dog no. 9. Vasculitis and perivascular cuff composed of neutrophils and mononuclear cells (E) Cerebrum of dog no. 10. Cavitation and perivascular cuff composed of mononuclear cells. (F) Cerebrum of dog no. 10. Marked malacic lesion and parenchymal infiltration of inflammatory cells (asterisk) were observed in the underlying white matter. H&E staining, 400× (A, B, C, and E), 1,000× (D), 40× (F) magnification.
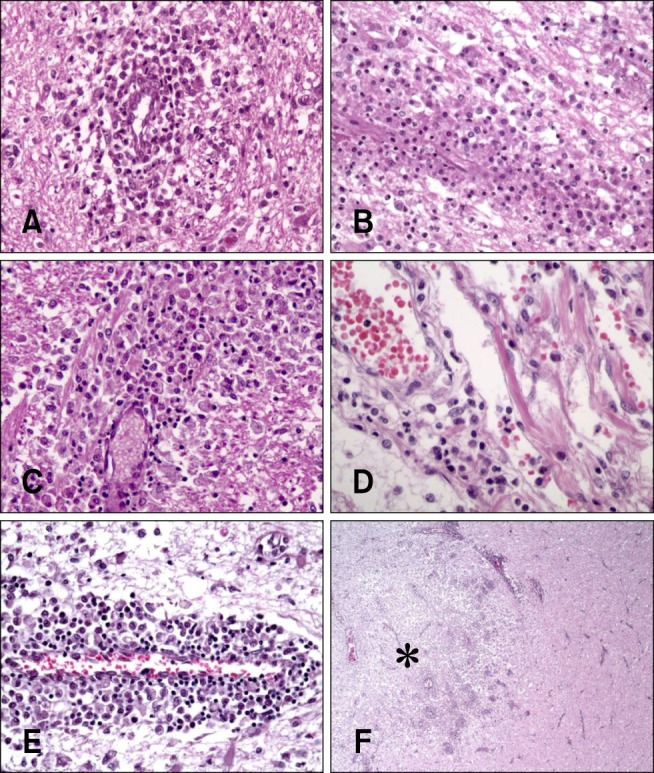
Fig. 4
Immunohistochemical staining of the cerebrum of five dogs (no. 2, 3, 4, 9, and 10) with EAE. (A) Dog no. 2. CD3-positive T cells were observed in the malacic neuroparenchyma. (B) Dog no. 4. CD3-positive T cells were present in the perivascular cuffs. (C) Dog no. 3. Macrophage populations (3H2617) were dense in the neuroparenchyma and perivascular cuffs. (D) Dog no. 9. Macrophage populations (3H2617) were dense in the meninges. (E) Dog no. 4. CD79a-positive B cells were found in the malacic neuroparenchyma. (F) Dog no. 10. CD79a-positive B cells were observed in the malacic neuroparenchyma. (G) Dog no. 4. Glial fibrillary acidic protein (GFAP)-positive astrocytes were present within and around the inflammatory lesions. (H) Dog no. 10. GFAP-positive astrocytes were present within and around the inflammatory lesions. 200× (A, C, E, and F), 400× (B, H, and G), 40× (D) magnification.
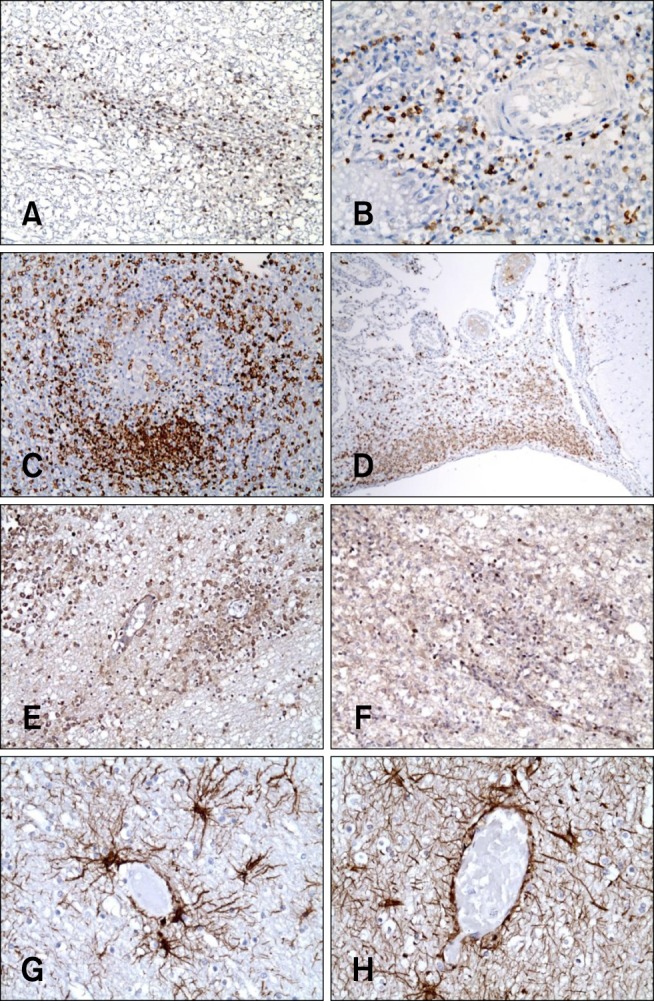
Fig. 5
Immunofluorescence staining with antibodies against GFAP and IgG in the cerebrum of three dogs (no. 2, 3, and 10) with EAE. IgG and IgG-positive plasma cells are represented by green signals produced by fluorescein isothiocyanate, and GFAP-positive astrocytes appear as red signals generated by Alexa 594. The nuclei were stained blue by DAPI. Many IgG-positive plasma cells (green) were adjacent to the GFAP-positive astrocytes (red). In dog no. 10, the cytoplasm of some astrocytes was positive for both IgG and GFAP (yellow).
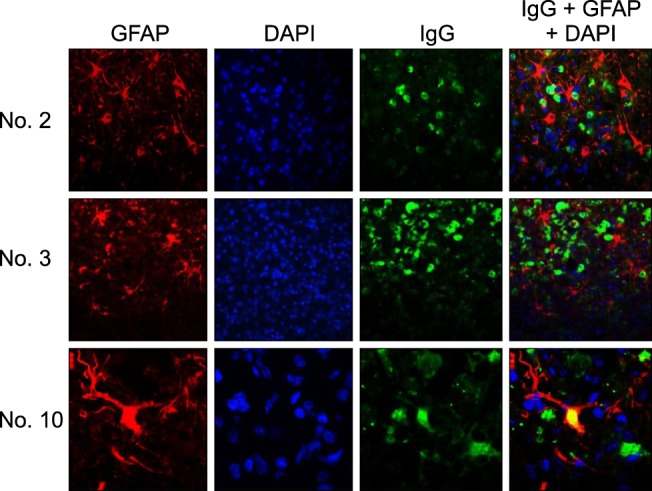